Introduction
Cationic antimicrobial agents, such as chlorhexidine, benzalkonium chloride and triclosan have been used as disinfect- ants in clinical settings and household environments for over half a century. Chlorhexidine and benzalkonium chloride are known for their residual activity and prolonged reduction in bacterial counts1. However it is still controversial whether residual products may act as antibiotics inducing reduced bacterial susceptibility and potentially compromising the efficacy of commonly used antibacterials. Biocide tolerant bacteria are more likely to be antibiotic resistant probably due to similar mechanisms of resistance2. In the hospital, cross- contamination with environmental isolates is considered a source of infections whereas in the community the use of cleaning products may exert pressure and develop tolerance in environmental bacteria2, 3.
Despite disinfection protocols 5% to 15% of inpatients will acquire a resistant hospital-acquired infection4. Cross resistance to disinfectants and antibiotics used in therapy has been documented in gram negative bacteria such as P. aeruginosa, Serratia and A. baumannii5. P. aeruginosa and A. baumannii complex usually cause opportunistic infections in intensive care unit (ICU) patients and have increased ability to adapt under adverse environmental conditions harbouring multiple determinants of resistance. These pathogens also have high propensity for clonal spread leading to environmental contamination and hospital outbreaks6. Despite control and hygiene programs, the risk for P. aeruginosa infections remains up to 30% in ICU-hospitalized patients7.
A. baumannii has been also reported as a frequently isolated pathogen at ICU (49%) 64.7% showing multidrug resistance8. One way to reduce the spread of nosocomial infections is through the strict monitoring of cleaning and disinfection protocols9. Most commonly used biocides have bactericidal effects at the concentrations recommended by the manufacturer, however some studies have suggested that over time, bacteria may develop resistance to these compounds, allowing their survival even at concentrations considered to be lethal2. Most importantly, antibiotic resistance is no longer confined to the hospital setting. In the last decade an increase of antibiotic resistant bacteria in the community has been observed. The associated factors for resistant bacteria in outpatients are still matter of study. Despite the usage of biocides in clinical and household environments, the effects of biocide tolerance on these two settings is yet to be determined. This work aimed to analyse the effects on growth, state transitions and susceptibility induced by biocide exposure on environmental isolates from households and a healthcare setting. We focused on particularly resistant pathogens such as A. baumannii complex, P. aeruginosa and E. coli, that can be frequently found in nosocomial outbreaks. The isolates were characterized and the bacterial response was monitored over time after biocide exposure and removal using flow cytometry and agar cultures.
Materials and methods
Strains and bacterial isolates. A total of 128 swabs were taken from sites where biocides are usually applied in house- holds and healthcare settings. Hospital samples were collected from a tertiary care hospital with a history of resistant A. baumannii complex outbreaks. Swabs from hospital and household surfaces were taken in transport media (Stuart media OXOIDÒ) and cultured in nutritive agar. Bacteria were subcultured using selective culture media (Eosin Methylene Blue agar MERCKÒ, and Cetrimide agar MERKÒ) and bacterial identification was performed using biochemical tests (BD Crystal Enteric/Nonfermenter, Becton Dickinson). Identification of Acinetobacter spp. was to the level of Acinetobacter baumannii complex. A total of 20 environmental isolates were obtained: five isolates of P. aeruginosa and five of A. baumannii complex were collected from ICU environments whereas five isolates of P. aeruginosa and five of Escherichia coli were collected from environmental surfaces in house- holds. P. aeruginosa ATCC 9027, A. baumannii ATCC 19606 and E. coli ATCC 25922 were used as reference strains.
Biocides. The following biocides were tested: chlorhexidine gluconate (CHxG) (Electrochemistry West SA), benzalkonium chloride (BAC) (New Chemical SAS) and triclosan (TC) (Ga- maQuim SA). Working solutions for CHxG and BAC were prepared at 0,5% and 0,1% in water. Triclosan was prepared at 0,5% in dimethylsulfoxide (DMSO). Toxicity of DMSO was assessed at concentrations up to 1% which was the highest test concentration.
Bacterial susceptibility. The in vitro activity of selected biocides on bacteria was tested to determine the minimum inhibitory concentration (MIC) and minimum bactericidal concentration (MBC)10. Briefly, bacteria suspensions were prepared in trypticase soy broth from fresh cultures. Starting inocula were adjusted to a 0.5 McFarland standard (1.5 x 108 CFU/ ml) and 100 µl was added to 2 ml of the biocide solution (5- 8x 106CFU/ml final inoculum) before being incubated for 24 h at 35 °C. The biocides were tested over a range of concentrations from 2.45 µl/ml to 5.000 μl/ml. The MIC was taken as the lowest concentration that prevents visible growth. For determination of the MBC, 100 µl of the relevant dilutions (i.e, the one defined as the MIC and the next two concentrations) were cultured in trypticase soy agar and the number of colonies was counted. The MBC was defined as the low- est concentration of biocide where no bacterial growth was observed. The experiments were performed at least twice in duplicate.
Quantitative suspension test. Bactericidal efficacy of biocides was determined as described previously after several contact times11, 12. Briefly, 1 ml of the selected biocide (at working concentrations as above mentioned) was incubated with 100 µl of bacterial suspension adjusted to a 0.5 MacFar- land (1.5x107CFU/ml final inoculum). The resulting solutions were incubated for 10, 15, 30 and 60 seconds of exposure.
At the end of the contact time 9 ml of the neutralizing agent (Tween 80: 30 g/L; thiosulfate: 5 g/L and sodium bisulphite 40% at pH 7.0) was added, mixed and then incubated for 10 min at room temperature. Thereafter, 100 µl of the neutralized test solution was cultured in nutrient agar plates. After incubation, colonies were counted and the number of recoverable bacteria was calculated. The potential effects of the neutralizing agent on the tested biocides and its bacterial toxicity were evaluated as suggested in the literature13. All bacterial isolates were exposed to concentrations of biocides commonly used in both, community and hospital disinfection protocols. The experiments were performed at least twice in duplicate.
Efficacy was expressed as the reduction factor (RF) when comparing the log number of colony forming units (CFU) before (CFUo) and after the contact time (CFUct ) as follows: RF =log10CFUo - log10 CFUct14
Susceptibility to antibiotics. Antimicrobial susceptibility was performed by disk diffusion agar with antibiotics used for the therapy of the tested isolates, such as: gentamicin, amikacin, ceftazidime, imipenem and ciprofloxacin10. For those isolates subjected to resuscitation, the MIC values were determined using MicroScan panels (Dade International Inc.) before and after biocide exposure and recovery. Cefepime, tobramycin, meropenem and ampicillin sulbactam (for A. baumannii complex) were also tested Interpretive standards published by the Clinical and Laboratory Standards Institute (CLSI) were used to categorize the isolates as susceptible, intermediate, or resistant15. Multidrug resistance was noted as non-susceptibility to at least one agent in three or more of the following classes of antimicrobials16: fluoroquinolones, aminoglycosides, carbapenems and broad spectrum cephalosporins.
Resuscitation assays. Selected isolates were exposed for 1 min (contact time with substantial bacterial reduction) at practical concentrations of biocides (i.e. 0.1% BAC, 0.5% CHxG and 0.5% TC). The cells were pelleted and resuspended in nutrient broth and then incubated at 37°C. After incubation, samples were monitored for bacterial viability every two days over seven days using flow cytometry. Bacterial populations were examined and aliquots from samples were cultured on nutritive agar for 24 hours at 37°C.
Flow cytometry analysis. Cell membrane integrity was evaluated using the nucleic acid double-staining based on SYTO9 and propidium iodide (PI)17. Bacteria were washed and resuspended in 500 µL of filtered distilled water before being labelled with 2.5 µM SYTO9 (a marker of viable and non-viable cells) and 750 nM PI (a dead cell marker) (InvitrogenTM Life Technologies).
The cells were incubated for 30 min at room temperature in the dark. Fluorescence emission and light scattering were analysed in a FACS Calibur flow cytometer (Becton Dickinson). The data were measured based on logarithmic scale amplification and over 10,000 events per sam- ple. To measure fluorescence intensity, green fluorescence was detected through a 525 nm, 50 nm band-pass filter (FL1 channel used for SYTO9) and red fluorescence through a 670 nm long-pass filter (FL3 channel, used for PI)17. Background controls of unstained, viable and killed bacteria were included for each subset of experiments. Data were acquired in du- plicate and experiments performed at least twice.
Data analysis: Cells were gated to analyse and quantify vi- able and non-viable bacteria. The data acquired were collected and processed using CELL Quest PRO software (BD Biosciences version 1.2) and FlowJo vX.0.7 software (Tree Star, Inc.). This work was approved by the University Hospital ´s Ethics Committee (approval number 200059932013).
Results
Distribution of households and hospital isolates. A total of 128 swabs were taken from sites where biocides are usually applied in household and hospital settings. P. aeruginosa isolates were found in kitchen drains, sinks and bathrooms from both household and clinical environments. E. coli was only found in household environments and A. baumannii complex isolates were only obtained from hospital samples, the latest were mainly isolated from dry surfaces, such as tables, handrails and dispensers (Table 1).
isolates were found in kitchen drains, sinks and bathrooms from both household and clinical environments. E. coli was only found in household environments and A. baumannii complex isolates were only obtained from hospital samples, the latest were mainly isolated from dry surfaces, such as tables, handrails and dispensers (Table 1).
Biocide tolerance and antibiotic susceptibility. MIC and MBCs were used to determine the susceptibility profile to each biocide (Table 2). TC, BAC and CHxG were chosen as these biocides have found to induce changes on antimicrobial and biocide susceptibility2, 18 . As susceptibility breakpoints for biocides remain to be established, the isolates were classified as “more tolerant” and “less tolerant or sensitive” by comparing the MIC or MBC to that obtained for the laboratory reference strains. An isolate was considered more tolerant when the MIC or MBC was at least two fold the MIC or MBC for the reference strain (i.e one fold change) (Table 2). Overall, P. aeruginosa showed higher MIC and MBC values than E. coli and A. baumannii complex. For BAC, household and hospital isolates of P. aeruginosa were more tolerant compared to the ATCC strain with MICs ranging from 19.5 to 312.5 µg/ml and MBC from 39 to 625 μg/ml (Table 2, Figure 1). P. aeruginosa isolates19D, 29H and 7H were the most tolerant (MIC 312.5 μg/ml and MBC ranging from 312 to 625 μg/ml), interestingly one isolate from a household toilet (19D) showed the highest MIC and MBC values. By contrast, E. coli and A. baumannii complex isolates showed lower MIC and MBC values oscillating between 4.9 and 78.2 µg/ml. All the A. baumannii complex isolates tested showed to be sensitive to BAC with MBC values lower than those of the reference strain (Table 2). All isolates were considered sensitive (less tolerant) to CHxG except one household isolate (P. aeruginosa 19D). When testing TC, all E. coli and A. baumannii isolates had MIC values ranging between 1.23 and 2.45 µg/ml, similar to those of the reference strain (2.45 µg/ml), however all the E. coli isolates had MBC values 8-fold higher than that of the reference strain and the A. baumannii isolates showed MBC values up to 4-fold higher than the reference (Figure 1).
Table 1 Distribution of bacterial isolates according to their environmental source
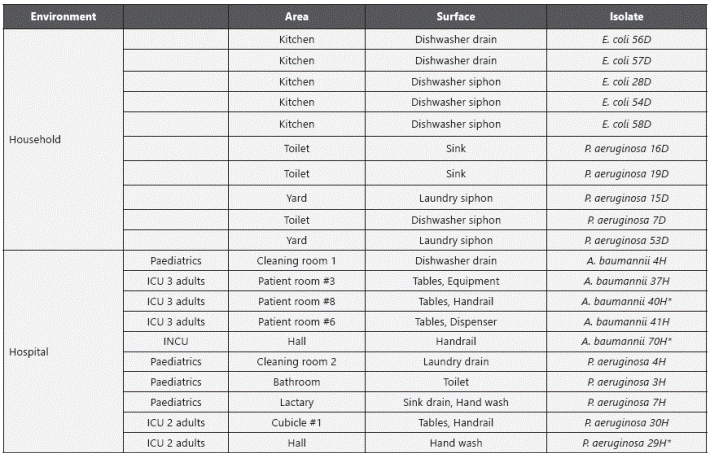
Intermediate Care Unit (INCU), Intensive Care Unit (ICU). * Multidrug resistant isolate.
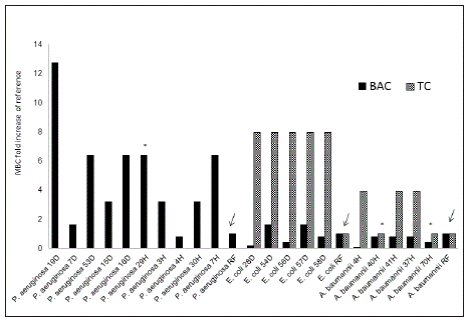
Figure 1 Biocide tolerance of P. aeruginosa (from household and hospital settings), E coli and A baumannii. MBC fold increase was calculated as the MBC value for each BAC or TC compared to that of the respective reference (RF) strain (arrows). *Multidrug resistant isolates. Reference strains were P. aeruginosa ATCC 9027, E. coli ATCC 25922 and A. baumannii ATCC 19606.
Susceptibility to antimicrobials is described in table 3. Four hospital isolates were resistant to at least one antimicrobial tested, two of them were also more tolerant to biocides (P. aeruginosa 29H was more tolerant to BAC and A. baumannii complex 41H more tolerant to TC). Three isolates were multidrug resistant: two isolates of A. baumannii complex (70H and 40H, an imipenem-resistant isolate) and one isolate of P. aeruginosa (29H). None of the isolates obtained from house- holds was resistant to the antibiotics tested.
Efficacy of biocides. After 1 min of exposure, all biocides were bactericidal against most of the isolates (RF > 2.4) except A.baumannii complex 4H. A 0.5% CHxG solution was highly efficient against household isolates, obtaining RFs > 2 after 10 seconds of exposure. However, to obtain the same RF for P. aeruginosa 30H, 19D, 7H, 16D and A. baumannii 4H, more than 30 seconds of exposure was needed. The results obtained with the 0.1% BAC solution were similar to those reported for CHxG. As expected, a 0.5% TC solution yielded only slight reductions in cell viability in the P. aeruginosa isolates even after 1 min of exposure (RF 0.05-0.3).
Survival of bacteria. Six isolates were subjected to resucitación assays, five considered more tolerant to biocides and one sensitive (Table 4). A BAC and CHxG tolerant isolate, P. aeruginosa 19D, (from household toilet, MBC 625 μg/ml for BAC and 156.5 μg/ml for CHxG ) was monitored after exposure to a bactericidal concentration of CHxG. Samples obtained at day zero showed only dead cells with PI uptake and no culture growth, however after four days the SYTO9 uptake started shifting to the right indicating an increase in the fluorescence and full recovery of viability in culture (Figure 2). Interestingly, a BAC tolerant P. aeruginosa 29H (from a ICU hand wash, MBC 312.5 μg/ml for BAC and 78.2 μg/ml for CHxG) recovered viability 2 days after bactericidal exposure to CHxG (45% as defined by the increase in SYTO 9 uptake), but remained uncultivable for 4 days suggesting that this isolate entered into a non-growing state (Figure 2). A. baumannii complex 40H was recovered in culture after 4 days of exposure to BAC with a concurrent increase in SYTO 9 uptake. Non-growing bacteria were also detected in E coli 28D using flow cytometry 5 days after bactericidal (625 μg/ml) exposure to BAC. A. baumannii complex 4H was recovered after 6 days in culture, however it became resistant to aminoglycosides after recovery and the resistant phenotype showed to be stable over time. The remaining resuscitated isolates showed no changes in the susceptibility profile.
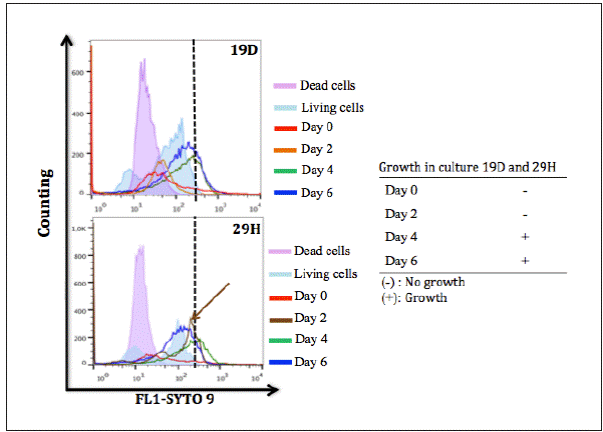
Figure 2 Cell viability of P. aeruginosa 19D and P. aeruginosa 29H after one min exposure to 0.5% CHxG. The arrow and dotted lines indicate still viable but non-growing cells.
Table 2 Biocide MIC and MBC values for hospital and household environmental isolates
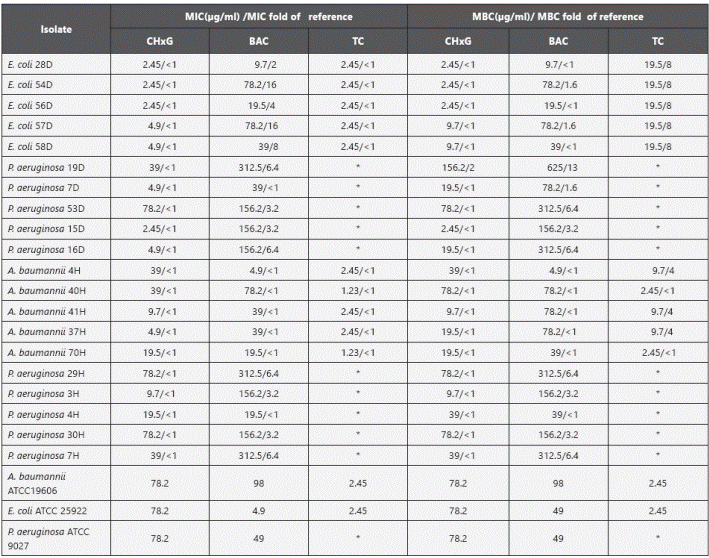
* Intrinsic resistance to triclosan, BAC: Benzalkonium chloride, TC: Triclosan, CHxG: Chlorhexidine gluconate. MIC or MBC fold of reference was calculated as follows: biocide MIC of isolate / biocide MIC of reference strain.
Discussion
Limited studies have analysed and compared the response of environmental bacteria after biocide exposure in isolates from hospital and household settings. Because the lack of a clear definition for biocide “resistance”, and the variability of the techniques that can be taken as reference19, in this work, we have analysed the susceptibility to biocides by comparing the MIC and MBC values of the tested isolates with laboratory strains. A. baumannii complex and E. coli isolates included in our study were considered sensitive to BAC and CHxG, whereas tolerance to TC was observed in all E. coli isolates from households and some of the hospital isolates of A. baumannii complex.
P. aeruginosa was more tolerant to BAC and TC. The MBC values of P. aeruginosa for BAC were considerably high being up to 13-fold higher than that of the reference strain. As referred by other authors, TC showed no efficacy against P. aeruginosa isolates due to the documented intrinsic resistance 20. Remarkably, one isolate of P. aeruginosa recovered from household was highly tolerant to all the biocides tested with the highest MBC values for CHxG, BAC and TC.
Our findings agree with previous studies by Gilbert & Moore showing that P. aeruginosa is relatively non-sensitive to qua- ternary ammonium compounds due to faulty biocide penetration from the outer membrane to the cytoplasmic mem- brane, where the target of their antimicrobial effect is located1. Likewise, Koburger et al. has shown MIC values for CHxG and TC of 32 µg/ml and >520 µg/ml respectively. The same authors showed a bactericidal effect of CHxG in P aeruginosa only after 5 minutes of exposure with 500 µg/ml and tolerance to TC concentrations above 5 000 µg/ml11.
The present study highlights the tolerance of P. aeruginosa to biocides observed in both, household and hospital environ- ments. This may be associated with the bacterial persistence and faster recovery P. aeruginosa after biocide exposure. As reported by others, bacteria often have the capacity to modify themselves to several transition states known as viable non-culturable (VBNC), persisters and dormant bacteria21. These refer to living cells that have lost the capacity to grow on routine culture media and differ from dead cells be- cause the latter have sustained membrane damage and loss of chromosomal and plasmidic DNA21. This unique survival strategy could be the key for success in adverse environmental conditions21. Some studies have found that processes considered normally bactericidal for bacteria could generate cells that remain in a non-replicative state22. However, injured bacteria may also remain uncultivable before dying or growing depending of the extent of damage. Therefore detection and differentiation of non-replicative states in bacteria are still matter of controversy and may require physiological markers21. Here, SYTO9 and PI were used to differentiate vi- able and dead cells based on cell membrane integrity, thus SYTO 9 has affinity for nucleic acids in cells with intact and damaged membranes and compete with PI which will only penetrate in damaged membranes. Fluorescence intensity of SYTO9 remains strong in live cells while in dead cells PI quenches the signal17. Therefore, a viable but non-growing state was considered when an increase in the SYTO 9 uptake was detected but no growth in culture was present.
This study has shown that under favourable conditions (i.e temperature and nutrient media) environmental isolates of P. aeruginosa, E. coli and A. baumannii complex can recover replicability, at least two days after removal of the biocide. Non-growing bacteria were detected by flow cytometry in P. aeruginosa after exposure to CHxG and E. coli after exposure to BAC. In P. aeruginosa, non-growing bacteria were detected in one multidrug resistant isolate from the hospital which was able to recover 48 hours faster than its counterpart from the household setting. This may suggest an increased ability of resistant hospital isolates of P. aeruginosa to survive and recover after biocide exposure however the precise mechanism of survival needs further investigation. One hospital isolate of A baumannii complex also showed changes in an- tibiotic susceptibility to aminoglycosides after resuscitation. It has been suggested that biocide exposure induces gene expression related with resistance mechanisms for aminoglycosides, ciprofloxacin, carbapenems and tetracycline18.
Table 4 Susceptibility profile of resuscitated isolates
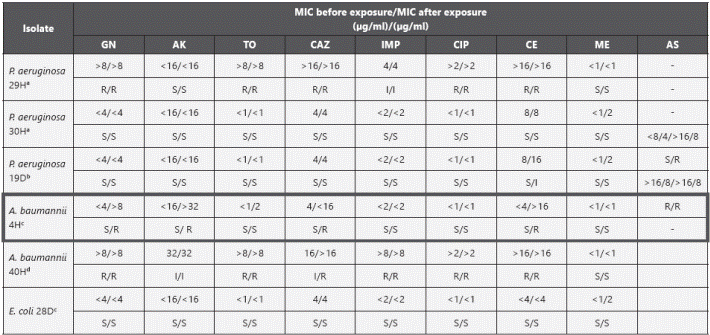
a BAC tolerant isolate; b BAC and TC tolerant isolate; c TC tolerant isolate; d Biocide sensitive isolate. Isolates recovered after exposure to CHxG are in bold the remaining isolates were recovered after BAC exposure. The box shows one isolate with susceptibility changes. GN: gentamycin, AK: amikacin, TO: tobramycin, CAZ: cetazidime, IMP imipenem, CIP: ciprofloxacin, CE: cefepime, ME: meropenem and AS: ampicillin sulbactam.
Although in our experiments it is possible that CHxG and BAC may cause sublethal injury, the conditions used for the resuscitation assays would favour the recovery of non-replicative states rather than injured bacteria suggesting that a non- replicative state may be involved in the long term survival of P aeruginosa isolates23.
To ensure that biocides continue to be effective, the regulation of biocide usage is urgently needed. Our findings may give insights of conditions inducing the spread and persistence of community and hospital bacterial isolates and particularly those multidrug resistant strains at the hospital level. In the community, a matter of concern is the TC tolerance found in E. coli isolates and BAC tolerance in P. aeruginosa. Triclosan, a biocide that may be still part of the formulations in household products, has been found to foster proliferation of P. aeruginosa and induce the expression of multidrug-resistant efflux pumps24. Intrinsic resistance of P aeruginosa to TC is well known, but the potential transfer of the molecular mechanism of resistance to other bacteria is a concern as it may induce cross resistance to ciprofloxacin24.
Despite of the efficacy of disinfectants at concentrations and contact times recommended by the manufacturer, hospital isolates often required longer contact times compared to household isolates. We found that even after one minute of bactericidal exposure, recovery of bacterial viability may be expected in hospital and household isolates and this ability to recover may be mediated by the transition to long term survival states (non-growing states) and/or biocide injured bacteria. Importantly, one isolate became resistant to antibiotics after recovery. Protocols for terminal disinfection to eradicate multidrug resistant strains should be revisited considering the recovery times for bacteria such as P. aeruginosa and A. baumannii complex and the efficacy of these protocols should be also assessed by non-culture based methods. Surveillance of biocide effects on antimicrobial or biocide susceptibility should be addressed to determine predictor markers of biocide resistance and cross-resistance over time. Furthermore, the effects of changes in biocide formulations and rotation should be also assessed.