Serviços Personalizados
Journal
Artigo
Indicadores
-
Citado por SciELO
-
Acessos
Links relacionados
-
Citado por Google
-
Similares em SciELO
-
Similares em Google
Compartilhar
Discusiones Filosóficas
versão impressa ISSN 0124-6127
discus.filos vol.13 no.20 Manizales jan./jun. 2012
¿La clasificación ontica vs clasificación epistémica de los estados cuánticos entrelazados?
Indeterminism in Sciences and Historico-philosophical Transdisciplinar
Advanced Research Center, Italia.
Universidad de Bergamo, Italia. michelecaponigro@unibg.it
Universidad de Bergamo, Italia. enricogiannetto@unibg.it
Resumen
En este breve artículo, y a partir de trabajos recientes, se analiza desde el punto de vista conceptual la siguiente pregunta básica: ¿puede la naturaleza de los estados cuánticos entrelazados ser interpretada ontológica o epistemológicamente? De acuerdo a algunos trabajos especializados, los grados de libertad (y la herramienta de la partición) de los sistemas cuánticos, nos permite establecer una posible clasificación entre los estados entrelazados y los estados factorizables. Sugerimos que la "elección" de los grados de libertad (o partición cuántica), incluso si se justifican matemáticamente, introducen un elemento epistémico, no sólo en los sistemas, sino también en su clasificación. Planteamos, en cambio, que no existen dos clases de estados cuánticos: entrelazados y factorizables sino tan solo una clase de estados: los entrelazados. De hecho, los estados factorizables se vuelven entrelazados para una elección diferente de sus grados de libertad (es decir, que están entrelazados con respecto a otros observables). De la misma manera, no hay particiones de sistemas cuánticos que posean un status ontológicamente superior con respecto a otros. Por todas estas razones ambas herramientas matemáticas utilizadas (i.e., las particiones cuánticas y los grados de libertad) son responsables de la creación de una clasificación incorrecta de los sistemas cuánticos. Finalmente, sostenemos que no se puede hablar de una clasificación de los sistemas cuánticos: todos los estados cuánticos muestran una naturaleza objetiva única, son todos estados entrelazados.
Palabras claveElementos epistémicos vs elementos ónticos, entrelazamiento cuántico, subsistemas (particiones y estados únicamente factorizables).
AbstractIn this brief paper, starting from recent works, we analyze from a conceptual point of view this basic question: can the nature of quantum entangled states be interpreted ontologically or epistemologically? According to some works, the degrees of freedom (and the tool of quantum partitions) of quantum systems permit us to establish a possible classification between factorizable and entangled states. We suggest, that the "choice" of degree of freedom (or quantum partitions), even if mathematically justified introduces an epistemic element, not only in the systems but also in their classification. We retain, instead, that there are not two classes of quantum states, entangled and factorizable, but only a single class of states: the entangled states. In fact, the factorizable states become entangled for a different choice of their degrees of freedom (i.e. they are entangled with respect to other observables). In the same way, there are no partitions of quantum systems which have an ontologically superior status with respect to any other. For all these reasons, both mathematical tools utilized (i.e. quantum partitions or degrees of freedom) are responsible for creating an improper classification of quantum systems. Finally, we argue that we cannot speak about a classification of quantum systems: all quantum states exhibit a uniquely objective nature, they are all entangled states.
Key wordsEpistemic vs. ontic elements, quantum entanglement, subsystems (partitions and uniquely factorizable states).
Systems and partitions
In spite of continuous progress, the current state of entanglement theory is still marked by a number of outstanding unresolved problems. These problems range from the complete classification of mixed-state bipartite entanglement to entanglement in systems with continuous degrees of freedom, and the classification and quantification of multipartite entanglement for arbitrary quantum states.
In this paper, starting form two important works, i) Torre (2010) and ii) Zanardi (2001), we will analyze the possible relationship among these elements: 1) The degrees of freedom of the quantum system.
2) The partitions of the quantum system.
3) The epistemic elements introduced from the procedures (1) and (2).
As we know, the relationship between quantum systems (QS) and their possible quantum entangled systems (QES) is not a trivial question. There are many efforts to understand this dynamics. Zanardi (2001) in his paper argues that the partitions of a possible system do not have an ontologically superior status with respect to any other: according Zanardi given a physical system S, the way to subdivide it in subsystems is in general by no means unique. We will analyze his conclusion in the following sections below.
According Zanardi the consequences of the "non uniqueness of the decomposition of a given system S into subsystems" imply (at the quantum level), a fundamental ambiguity about the very notion of entanglement that accordingly becomes a relative one. The concept of "relative" for an entangled system has been developed by Viola and Barnun (2006).
They concentrate their efforts on this fundamental question: how can entanglement be understood in an arbitrary physical system, subject to arbitrary constraints on the possible operations one we may perform for describing, manipulating, and observing its states? In their papers, the authors proposed that entanglement is an inherently "relative concept", whose essential features may be captured in general in terms of the relationships between different observers (i.e. expectations of quantum observables in different, physically relevant sets).
They stressed how the role of the "observer" must be properly acknowledged in determining the distinction between entangled and unentangled states.
Quantum entanglement: brief overviewFrom a phenomenological point of view, the phenomenon of entanglement is quite simple. When two or more physical systems form an interaction, some correlation of a quantum nature is generated between the two of them, which persists even when the interaction is switched off and the two systems are spatially separated.
Quantum entanglement describes a non-separable state of two or more quantum objects and has certain properties which contradict common physical sense. While the concept of entanglement between two quantum systems, which was introduced by Erwin Schrödinger (1936), is well understood, its generation and analysis still represent a substantial challenge. Moreover, the problem of quantification of entangled states; is a long standing issue debated in quantum information theory. Today the bipartite entanglement ("two-level systems", i.e. qubits) is well understood and has been prepared in many different physical systems. The mathematical definition of entanglement varies depending on whether we consider only pure states or a general set of mixed states (Giannetto)1. In the case of pure states, we say that a given state |Y 〉 of n parties is entangled if it is not a tensor product of individual states for each one of the parties, that is,

For instance, in the case of 2 qubits A and B (sometimes called "Alice" and "Bob") the quantum state

is entangled since |Y+〉 ≠ |vA〉A ⊗ |vB〉B. On the contrary, the state

is not entangled, since

A pure state like the one from Eq. 2 is called a maximally entangled state of two qubits, or a Bell pair, whereas a pure state like the one from Eq. 4 is called separable. In the general case of mixed states, we say that a given state p of n constituent states is entangled if it is not a probabilistic sum of tensor products of individual states for each one of the subconstituents, that is,

with {pk} being some probability distribution. Other-wise, the mixed state is called separable. The essence of the above definition of entanglement relies on the fact that entangled states of n constituents cannot be prepared by acting locally on each one of them, together with classical communication among them. Entanglement is a genuinely quantum-mechanical feature which does not exist in the classical world. It carries non-local correlations between the different systems in such a way that they cannot be described classically.
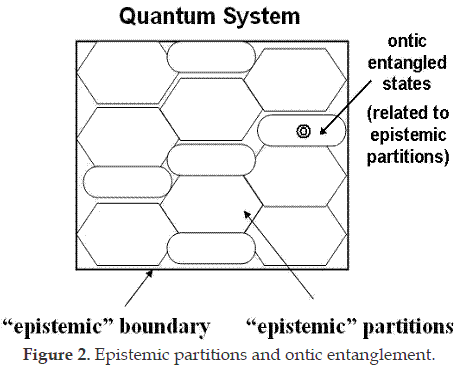
Are quantum states all entangled?
As mentioned above, the recent work by Torre (2010) is a fundamental paper which gives us the possibility to speculate about the nature and the classification of entangled states. The paper demonstrates that a state is factorizable in the Hilbert space corresponding to some choice of degrees of freedom, and that this same state becomes entangled for a different choice of degrees of freedom.
Therefore, entanglement is not a special case, but is ubiquitous in quantum systems. According to the authors, one may erroneously think that there are two classes of states for the QS: 1) factorizable and 2) entangled, which correspond to qualitative difference in the behavior of the system, close to classical in one case and with strong quantum correlations in the other. They argue that this is indeed wrong because factorizable states also exhibit entanglement with respect to other observables. In this sense, all states are entangled; "entanglement is not an exceptional feature of some states but is ubiquitous in QM".
To sum up this conceptual analysis by Torre and Zanardi, we think that there is an unclear relationship among these elements:
1) Factorizable states (Torre).
2) Entangled states.
3) The (choice) of partitions of quantum system (Zanardi).
4) The role of the observer (in determining the distinction between entangled and unentangled states).
We think that all points (except the second point) introduce epistemic elements in the analysis and in the classification of the quantum systems. We suggest that the second point is the key to understand the nature of the underlying physical reality. We argue in the next sections, that the conceptual analysis of Torre and Zanardi differs from what we suggest concerning the epistemic elements introduced in their papers.
Factorizability of a state as epistemic property?An important question is related at the property of factorizability of quantum state. Is the factorizability tool an objective property? Briefly stored, is factorizability an objective property of the system or is it a feature of (our) description of system (i.e. an epistemic property?)
With reference to Torre's paper (2010), the authors show that factorizability and entanglement "are not preserved" in a change of the degrees of freedom used to describe the system, they demonstrate in details case that the factorizability of a state is a property that is "not" invariant under a change of the degrees of freedom that we use in order to describe the system. From mathematical point of view (1), they consider a quantum system with two subsystems S = (SA; SB) that may correspond to two degrees of freedom A and B. The state of the system belongs to the Hilbert space H = HA ⊗ HB and the two degrees of freedom are represented by operators A ⊗ I and I ⊗ B. Suppose it is given that the system has a factorizable, none entangled, state Y = YA ⊗ YB with YA and YB arbitrary states (not necessarily eigenvectors of A and B) in the spaces HA and HB. Then there exists a transformation of the degrees of freedom F = F (A, B) and G = G (A, B) that suggests a different factorization, H = HF ⊗ HG, where the state is no longer factorizable: Y ≠ YF ⊗ YG with YF ∈ HF and YG ∈ HG. The state becomes entangled with respect to the new degrees of freedom; the factorizability of states is not invariant under a different factorization of the Hilbert space.
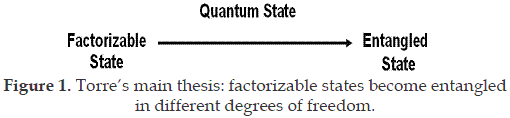
To conclude, they have shown that for any system in a factorizable state, it is possible to find different degrees of freedom that suggest a different factorization of the Hilbert space where the same state becomes entangled; for this reason they argued that every state, even for those factorizable, it is possible to find pairs of observables that will violate Bell's inequalities. The figure above (n. 1) summarize Torre's thesis.
The authors analyze also the inverse problem: the fact that the appearance of entanglement depends on the choice of degrees of freedom can find an interesting application in the "disentanglement" of a state; one can, sometimes, transform an entangled state into a factorizable one by a judicious choice of the degrees of freedom.
To conclude, we think that the epistemic element is inherent in the possibility to "choose" the degrees of freedom of the quantum system: this possibility affects the classification of quantum states in entangled or factorizables. In fact, it is simple to ask these epistemological questions: a) what are the degrees of freedom for a quantum system? b) Is it a complete set that describe all quantum properties? Can be a particle entangled in one context be factorizable in another context?
The partitions of quantum system as epistemic property?As we have seen, given a quantum system, the way to subdivide (to partition) it in subsystems in "not unique". We call this first phase "epistemic", as in fact we are able to decide how to partition the quantum system.
The conclusion of this operation is most important of its premise: in fact if we find (in the subsystems) an entangled state, this state has an ontological nature "but only if referred" to that kind of particular partition. We have, in other words, an objective entangled state for an epistemic partition! For these reasons, the notions of an entangled state become a relative concept and the relativity of this concept is linked to us, at the choice of partitions or degrees of freedom. At the same time, the property of the entangled state is objective. The figure above (n. 2) represent our view of Zanardi's problem.Some considerations and conclusions
We have seen that quantum systems admit a variety of tensor product structures depending on the complete system of commuting observables chosen for the analysis; as a consequence we have different notions of entanglement associated with these different tensor products.
We notice that, in the determination of whether a state is factorizable or entangled, the factorization of the Hilbert space is crucial and this factorization depends on the choice of the observables corresponding to the degrees of freedom. In the same way, as Zanardi stressed, given a quantum system, the way to subdivide it (via partitions) in subsystems it is "not unique"; the partitions of a possible system have not an ontologically superior status with respect to any other.
Based on these points, we argue that the criteria of partitions and factorizability (or partitions) contain an a priori epistemic element, the figure (n. 3) summarize our position.
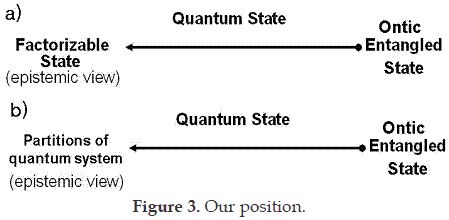
In conclusion, we suggest that all quantum system exhibit an objective nature that is entangled, at basic level the underlying physical reality is entangled. A quantum state could be non-entangled if and only if it would be factorizable for every possible partition or choice of degrees of freedom, but this can never occur. The epistemic level emerges with the "observer" (partitions or degree of freedom) the physicists and philosophers should consider these arguments in their debates.
Footnotes
1 Where it is discussed the reason why entanglement generally requires a density matrix formalism.
Bibliographical references
De la Torre, Alberto Clemente et al. "Entanglement for all quantum states" Eur. J. Phys. 2010: 325-332. Print. [ Links ] [ Links ]
Schrödinger, E. "Discussion of probability relations between separated systems". Proceedings of the Cambridge Philosophical Society. Oct. 1936: 555-563. Print. [ Links ] [ Links ]
Viola, Lorenza and Howard Barnun. "Invited contribution to the Proceedings of the Boston Colloquium for Philosophy of Science on "Foundations of Quantum Information and Entanglement". Quantum Physics. Boston: Cornell University Library, 2006. Online. [ Links ]
Zanardi, Paolo. "Virtual quantum subsystems". Phys. Rev. Lett. 2001. Print. [ Links ]