The coffee postharvest process is very important for preservation of coffee quality bean (Ribeiro et al., 2011; Carvajal et al., 2012). In Colombia, coffee is processed with wet way. The wet process of coffee includes depulping, fermentation, sorting, washing and drying the coffee bean. Coffee is often stored in post-harvest facilities.
Drying is considered a critical step of process. The coffee is dried in the interest of maintaining quality and storing it for extended periods of time (Borém et al., 2007; Ciro et al., 2010; Ciro et al., 2011). The main problems in the cup (taste) arise from poor drying and storage. The development of microorganisms is a factor in the environment that arises only when adverse storage conditions permit excessive moisture accumulation in the grain bulk or when grain is initially stored above permissible safe moisture contents required for its preservation (Puerta, 2008; Oliveros et al., 2013).
As the harvest of coffee occurs in the rainy season in Colombia, some of the time, the external environment has relative humidity above 70%; in the case of natural ventilation, this constitutes a natural bioclimatic limitation (Osorio et al., 2015).
The bioclimatic environment plays an important role in the conservation of parchment coffee quality. Fungi live and reproduce best in a range of 70 to 80% relative humidity, whereas yeast and bacterial development require humidity higher than 85% in the intergranular air; temperatures higher than 50 °C can kill the embryos from coffee seeds and start the decomposition process. In the tropical zones with relative humidity above 70%, and temperatures above 27 °C, freshly harvested grains, are in favorable environment to the development of insects (Navarro and Noyes, 2001).
The biological risk is latent in facilities that perform the wet processing of coffee; for example, according to Puerta (2006), Aspergillus ochraceus (the leading producer of Ochratoxin A) was found in 70% of the facilities tested in Colombia, in coffee postharvest facilities, as well as solar dryers, parchment coffee and green coffee. This author argued that when adequate conditions of humidity, temperature, time and poor hygiene occur, these microorganisms can proliferate.
Approximately, 70% of the volume of coffee that is produced in Colombia is dried mechanically (González et al., 2010), generally inside the wet processing facilities of coffee. In Colombia, this process usually generates a lot of steam and heat in the facilities where the coffee is dried and stored. In post-harvest process, the largest power consumption occurs in the mechanical drying process, which, having an efficiency of 50% (Puerta, 2006), added large amounts of steam and thermal energy to the building, which increases the temperature and moisture inside. Consequently, the biological risk is increased.
Poor control and design of buildings for the wet processing of coffee can compromise product quality due to inadequate bioclimatic environments (Osorio et al., 2015). Humid environments and high temperatures during storage are risk conditions that can physically damage the grain, causing decomposition and deterioration of the quality of the product (Puerta, 2008).
To analyze and suggest bioclimatic and air quality solutions within agro-industrial buildings, the application of mathematical and computational modeling and simulations is increasingly used (Norton et al., 2009). Simulation is a very interesting tool in the design and evaluation of buildings, as the bioclimatic conditions of the buildings involve complex aspects such as energy flows, transient weather variables, stochastic occupancy patterns, etc., that traditional design methods based on experience or experimentation cannot satisfactorily quantify (Bre et al., 2013).
EnergyPlusTM is one of the most used programs for energy and bioclimatic simulation for buildings (DoE, 2012), which is a free open source software developed by the US Department of Energy (DoE). This program was used by Osorio et al. (2015) and Osorio et al. (2016) for the bioclimatic and energy analysis of coffee post-harvest facilities with good results.
Its main input variables are: 3D building design, physical and thermodynamic properties of building materials, internal equipment, and weather file of the site where the building is located, in order to perform transient analysis for energy efficiency, bioclimatic variables and air quality within buildings, across balances of mass, energy and chemical composition.
This study aimed to simulate the thermal environment of three typical installations of the Colombian coffee postharvest, in order to compare and analyze the internal bioclimatic conditions, in terms of temperature and relative humidity in order to preserve grain quality.
MATERIALS AND METHODS
Location of buildings and production
The three buildings are located in the department of Antioquia - Colombia, in the municipality of Barbosa (at coordinates 6°26'15"N, 75°19'50"W), near to Medellin city. For these simulations, the climate file of Medellin was used (at coordinates 6°14'41"N 75°34'29"W, altitude of 1500 m), which has a representative climate for coffee of this zone (average conditions of temperature of 16 to 28 °C and relative humidity of 60 to 80%). The three farms produce the same amount of coffee, with a coffee cherry production of about 156,250 kg per year-1 (31,250 kg per year of parchment coffee). This study was conducted during the month of November (main harvest of 2015).
Description of the buildings
The facilities volumes 3D were drawn in SketchUp® program (Figure 1). The first geometry, type a (Figure 1A), has two floors (of equal size) in a stepped form. On the first floor was the mechanical drying area, while the second floor contained the area for pulped and fermented coffee. The dimensions of this building are: 5.50 m wide x 9.50 m long x 4.0 m high.
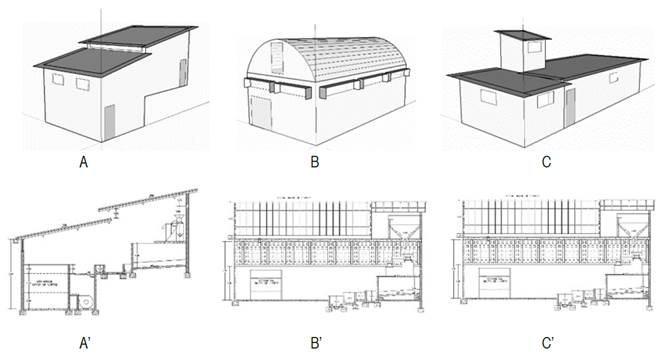
Figure 1 3D geometries and architectural cuts of three buildings for the wet processing of coffee types a (A, A'), b (B, B') and c (C, C').
Type b (Figure 1B) has two separate floors: the first floor is the area for pulping, fermentation and mechanical drying of the coffee and has dimensions of 11.0 m long x 6.0 m wide x 3.5 m high. The second floor consists of a parabolic solar dryer with plastic covering, measuring 11.0 m long x 6.0 m wide x 2.3 m high. Between the first and second floor, there is a lightweight concrete and brick slab.
Type c (Figure 1C) has two rooms: the main room is the area for the pulping and fermentation of coffee, while the second room is a mechanical dryer for the coffee. Type c has dimensions of 10.40 m long x 5.0 m x wide x 2.7 m high. The second room has dimensions of 2.0 m long x 2.0 m wide x 4.60 m high. Unlike types a, and b, in the type c, as its mechanical drying machine protrudes of the building, expels the vapor and heat produced in the drying process to the external environment.
Each floor of type b, as well as the room for the wet processing of coffee and mechanical drying machine of type c, were analyzed as an independent thermal area, while type a was analyzed as a single thermal zone. In each thermal zone, the thermal characteristics of the materials and other details of each patterned surface are described (DoE, 2014).
The three types were built with 15 cm unplastered brick, and the windows of all buildings were open all the time. Types a, and c had a fiber cement roof, whereas type b had a lightened slab between the first floor and the solar dryer (second floor).
In the three types, there were concrete stairs, fermentation tanks, and a coffee hydraulic classifier inside; to these models, one layer of concrete and other of ceramic were added at the bottom, with a volume of 3.5 m3 in order to account for the effect of thermal inertia of this mass. In addition, it were measured the volume and natural ventilation area of each building of each type.
Boundary conditions
The second floor of type b corresponds to the parabolic solar dryer of the coffee, which has a polyethylene plastic cover and a coffee layer with an average humidity of 33% wb and a 0.03 m thick slab. The solar dryer has two openings of 1.6 m2 each for natural ventilation during the day. For the night conditions, it is assumed that the solar dryer was closed. With respect to equipment, for all three types, there are lights, a humidity processing module to peel and sort coffee with a capacity of 2000 kg of coffee cherry per hour, and a mechanical drying machine with a capacity of 1125 kg of parchment coffee per day.
In the contour conditions of the models, for the thermal properties of the washing coffee, we used equations 1 and 2 proposed by Montoya et al. (1990), for the specific heat Cp, J kg−1 °K−1 ) and density of the coffee (ρC, kg m-3). These properties are functions of the moisture content of the product on a dry basis (Mdb, decimal dry basis).
The thermal properties of building materials used as boundary conditions is shown in Table 1.
Table 1 Thermal properties of construction materials.
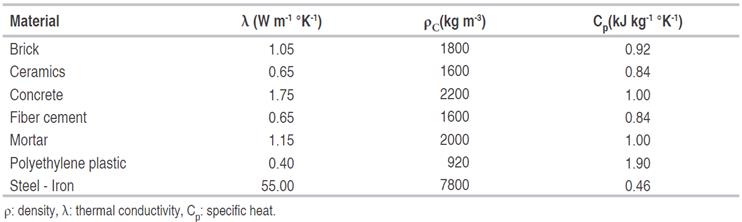
Source: Adapted from NBR-15220 (ABNT, 2003), INMETRO, (2013),and LabEEE, (2015).
For the boundary conditions of energy balance, it is necessary to calculate the heat generated within each building (heat generated by machines, luminaries and human metabolism). Table 2 shows the power values of the machines and lighting. For the three types, simulations were performed with heat exchanger drying machines using coal (anthracite) as fuel, which, according to Oliveros et al. (2009) has a consumption of 0.224 kg of coal per kg of dry parchment coffee, with a calorific value of 33440 kJ kg-1.
The metabolic rate, i.e., the metabolic energy that the human body expends while performing physical activities, varies from person to person, according to the activity and working conditions performed. The value per person of 423 W was used in this study for the metabolic rate as, according to ASHRAE (2001); this value corresponds to heavy work activity and the handling of 50 kg sacks.
Table 3 shows the usage patterns of the three wet coffee processing facilities models, that is, when the machines are operating, and how many workers are into facilities and in that schedule. Mechanical drying and pulping require the same working hours for three typologies. The solar dryer (in type b) is only opened during the day to encourage the mass exchange of water, and is closed at night to retain thermal energy, prevent condensation and prevent the ingress of moist air from outdoors.
The internal environment of the buildings was simulated for the month of November, during the main harvest of the year, which coincided with the second season rainfall in this part of Colombia (Oviedo and Torres, 2014).
Statistical analysis
A statistical analysis of variance (P<0.001) and test media (Tukey, P<0.05) was performed for the analysis of temperature and relative humidity (hourly), with four treatments: outdoor, type a, b, c and outdoor as a control.
In addition, an analysis of the number percentage of hours that facilities remained with a relative humidity between 90 and 100%, 70 and 90% and less than 70% was performed, in order to assess the bioclimatic environment and biological risk for the preservation of the parchment coffee quality .
RESULTS AND DISCUSSION
Comparing type a, and b, the effect of natural ventilation area on lowering internal temperature was observed, agreeing with Osorio et al. (2015), and Osorio et al. (2016). Nevertheless Table 4 shows that the type c building although had the smallest ventilation area, the steam and heat of the mechanical drying process is removed from the building, as its mechanical drying machine protrudes from the building, to expel the vapor and heat produced in the drying process to the external environment. Its average temperature and relative humidity were statistically lower (Table 5).
On the other hand, type a, presented an internal environment with an average temperature higher than above 27 ºC and average relative humidity above 70%, which according to Navarro and Noyes (2001), constitutes an environment of high risk of attack of fungi and bacteria in grain storage; this means that type a, presented the higher biohazard in its indoor environment, due to its high average temperature and high relative humidity.
Taking into account that the grain moisture contents that are in equilibrium with the surrounding air containing a lower relative humidity than 70%, are considered safe (Navarro and Noyes, 2001; Puerta, 2008), and the internal environment of type a, was close to saturation 9.70% of the time, and spent more than half of the time with a relative humidity greater than 70%, 51.52% of the time (Table 6), this leads to a danger of proliferation of fungi and bacteria that are detrimental to grain quality.
On the other hand, type c, presented the most adequate bioclimatic conditions to conserve the quality of dried parchment coffee (under storage conditions), since in the month of harvest peak and rainiest in the study area (November) the Relative humidity was in a safe range 70.64% of the time, with an average relative humidity of 63.4% and an average temperature of 23.5 °C, decreasing the risk of attack by fungi, bacteria and insects (Navarro and Noyes, 2001; Puerta, 2008).
In Colombia, it is common to store dry parchment coffee during the harvest in buildings of processing of coffee for several weeks, in order to increase the volume of dry parchment coffee to transport and save money on freight costs (Osorio et al., 2015). In this context, type a, had a greater biological risk regarding the creation of fungi, bacteria and insects (hot and humid environment), meaning that the grain may be re-moistened and damaged (Puerta, 2008). In the same context, type c showed the most innocuous bioclimate (safe biologically), with a lower average temperature of 27 °C (Table 5), an internal relative humidity less than 70% most of the time, with a humidity close to saturation for almost no time (Table 6).
CONCLUSIONS
In the buildings of post-harvest coffee with steam and heat generation inside, the effect of ventilation on lowering internal temperature was observed. However, in this study was observed that the best bioclimatic results for coffee parchment were presented in the case where mechanical drying steam from coffee was thrown directly into the external environment (type c).
The indoor environment of type a (average temperature above 27 °C, and average humidity above 70%), had a greater biological risk of the creation of fungi and bacteria, meaning that the grain may be re-moistened and damaged.
In type c, as its mechanical drying machine protrudes from the building, to expel the vapor and heat produced in the drying process to the external environment, this type showed the most innocuous bioclimate conditions (safe biologically), with average temperature of 23.5 °C and average relative humidity 63.4% relative humidity.