Purple passion fruit (Passiflora edulis f. edulis), also known as gulupa, is a vine plant of the family Passifloraceae, which in recent years has gained importance in the world fruit market due to its exotic nature, excellent organoleptic properties and high content of bioactive compounds (Dhawan et al., 2004; Fischer and Rezende, 2008). Purple passion fruit is closely related to yellow passion fruit (P. edulis f. flavicarpa) but has a sweeter taste and is less acidic due to a higher content of sugars and a lower concentration of citric acid (Morton, 1987; Manicom et al., 2003). The gulupa fruit is also a good source of iron, vitamins A, B and C, amino acids, carotenoids and flavonoids and can be eaten fresh or used in the preparation of beverages, jams, chutneys and desserts in general (Morton, 1987; Dhawan et al., 2004; Rodríguez-Amaya, 2012). The leaves of purple passion fruit are frequently used in infusions due to their sedative and anti-inflammatory properties (Morton 1987; Ferreres et al., 2007, da Silva et al., 2013); the flowers, on the other hand, are often used as ornamentals (Manicom et al., 2003).
The origin of purple passion fruit has been located to an area comprising southern Brazil, Paraguay and northern Argentina (Morton, 1987) and its cultivation is restricted to tropical and subtropical regions at altitudes between 1400-2500 MASL with temperatures in the 15 to 30 °C range (Jiménez et al., 2012). Gulupa is mainly cultivated in Colombia, Ecuador, Peru, South Africa, Kenya, Zimbabwe, India, New Zealand, Sri Lanka, Uganda, Australia and some Pacific islands (Fischer and Rezende, 2008). Total production of passiflora fruits is estimated at 805,000 t year-1, with Brazil (56%), Ecuador (31.0%), and Colombia (3.7%) as the biggest producers (Manicom et al., 2003; Fischer and Rezende, 2008; Rodríguez-Amaya, 2012).
During the past decade, the demand of gulupa in Colombia has increased significantly to become one of the most important exotic fruit exports in the country, just behind banana and Cape gooseberry (Jiménez et al., 2012). The total cultivated area of gulupa in Colombia comprises approximately 500 ha mostly concentrated in the provinces of Antioquia (36.87%), Cundinamarca (28.8%) and Boyacá (13.51%) and smaller amounts produced in Valle del Cauca, Magdalena, Huila and Santander (Guerrero et al., 2011; Agronet, 2015). In spite of the increasing demand, the cultivated area of gulupa has been in decline since 2009 due to the impact of fungal, bacterial and viral diseases. This effect has been most dramatic in the province of Cundinamarca where the total cultivated area went down from 630 ha in 2009 to 204 ha in 2013, and it has had one of the poorest yields in the country (Agronet, 2015). In 2010, a study on plants showing symptoms of viral infection in the Sumapaz region of Cundinamarca confirmed the infection of gulupa by the potyvirus Soybean mosaic virus (SMV) (Camelo, 2010). Further work, reported SMV incidences of 23.7 and 27.8% in two gulupa producing regions of Cundinamarca (Gordillo, 2011). Besides SMV, there are reports of gulupa infections by another potyvirus, Cowpea aphid-borne mosaic virus (CABMV), which was detected using ELISA in leaves showing mosaic symptoms in Cundinamarca and Valle del Cauca (Gordillo, 2011).
In spite of ranking third in the cultivated area (74 ha), Antioquia has the highest yield of purple passion fruit (31.2 t ha-1) and is also the largest producer with 36.8% of the national production (Agronet, 2015). Unfortunately, commercial gulupa cultivation plots in this region have started to be affected by an unknown disease that results in significant yield reductions. The symptoms of this pathology have been previously observed in gulupa fields in Cundinamarca and are suggestive of an emergent disease of viral origin. A Next-generation sequencing (NGS) study on gulupa fields in the municipality of Jardin demonstrated the presence of a SMV strain infecting this plant in Antioquia and reported the first complete SMV genome infecting a host different to soybean (Jaramillo, 2017). As different viruses have been found to infect P. edulis in the world (i.e., Cucumber mosaic virus, Passionfruit mottle virus, Bean yellow mosaic virus), it is likely that other viruses could be found infecting this crop in Antioquia as a result of the free movement of infected plants and the lack of seed certification programs in Colombia. To further characterize the virome infecting gulupa in Antioquia, in this study a NGS analysis was performed from a producing region in the eastern municipalities of El Retiro and La Ceja. The molecular properties of this virus are discussed and its widespread presence in this region demonstrated by RT-PCR and RT-qPCR tests.
MATERIALS AND METHODS
Next-generation Sequencing
High-throughput sequencing of the gulupa transcriptome was performed on a bulked sample of two leaves from approximately 10 plants with symptoms of viral infection such as mosaic, mottling along the veins, enation and severe leaflet deformation (Figure 1) collected at the municipalities of El Retiro (6°03’15.60” N75°30’5.39”W) and La Ceja (6°01’39.4”N, 75°25’53.8”W ) (Antioquia, Colombia). After grinding the leaf tissue with liquid nitrogen, total RNA was extracted with Trizol (Ambion, Thermo Fisher Scientific, EEUU) and rRNA depleted with the Ribo-Zero Plant kit (Illumina, EEUU). Libraries were constructed using the TruSeq RNA Sample Preparation Kit (Illumina, EEUU) and sequencing performed with the Illumina HiSeq 2500 system provided by Macrogen (South Korea) (Muñoz-Baena et al., 2017). Adapter sequences and low-quality bases were removed with Trimommatic (Bolger et al., 2014). Sequence assembly was performed with Trinity (Grabherr et al., 2011) and viral contigs identified by a local BLASTN search using a database of viral reference genomes. The database comprised a collection of 10,884 completely sequenced plant viruses representative of all the taxa and genome types currently known for this group of pathogens and were downloaded from NCBI. The PFYMV genome assembly was confirmed by mapping with BWA (Li and Durbin, 2009) using the Trinity contig as the reference. Sequencing of the purple passion fruit transcriptome resulted in a paired-end library of 21,480,984 reads (101 nt/read) for a total of 2,169,579,384 sequenced nucleotides. The consensus genome sequence was deposited in GenBank under accession KY823429 with PFYMV_Antioquia as isolate name.
RT-PCR and RT-qPCR
To confirm the presence of PFYMV_Antioquia in eastern Antioquia, leaf samples were collected in one gulupa plot (~0.4 ha) in the municipality of La Ceja (LCS= La Ceja symptomatic sample; LCA= La Ceja asymptomatic sample) and three more (<1 ha) in El Retiro (R#S=Retiro symptomatic sample; R#A= Retiro asymptomatic sample) (Antioquia, Colombia); two bulked samples consisting of nearly 20 leaves with and without viral symptoms were randomly collected at each plot. RNA was extracted with Trizol (Ambion, Thermo Fisher Scientific, EEUU) and eluted in 40 μL of DEPC treated water; the purity and concentration were determined by absorbance readings at 260 and 280 nm using a Nanodrop 2000C (Thermo Fisher Scientific, EEUU). Retrotranscription was performed for 30 min at 50 °C in 20 μL containing 200 U of Maxima Reverse Transcriptase (Thermo Fisher Scientific, EEUU), 1X RT Buffer, 0.5 mM dNTP Mix, 100 pmol of the reverse primer, 20 U of RiboLock RNase Inhibitor and 100-500 ng of total RNA (Muñoz-Baena et al., 2017). All primers were designed in this study using the PFYMV_Antioquia genome sequence reported in this work. RT-qPCR amplification of the RNA-dependent RNA polymerase (RdRp) region was carried out with primers Tymo_F_RdRp1 (5’-CGG TAC CCT TCC ACA ACT CC-3’) and qTymo_R_RdRp1 (5’-AGG GAG CAG AAG AAT TTC G-3’), targeting a 137 bp fragment of the RdRp region; primers Tymo_F_CP (5’-AGT CTC CGA CTC AAT CAG CG-3’) and qTymo_R_CP (5’-GCG ACG AGA GAG GTG AGT CG-3’) were used for the capsid protein (CP) segment, which resulted in a fragment of 90 bp. For RT-PCR, primer Tymo_R_RdRp1 (5’-CCT GGT GTA AGT GAA CAG GGC-3’) was used in combination with Tymo_F_RdRp1 resulting in an amplicon of 576 bp and primer Tymo_R_CP (5’-GAA TGG AGT TCG ATC GTG C-3’) was used in combination with Tymo_F_CP, resulting in an amplicon of 405 bp.
For the qPCR, the Maxima SYBR Green/ROX qPCR Master Mix (2X) kit (Thermo Fisher Scientific, EEUU) was used in 25 μL of the reaction containing 12.5 μL mix, 10 μL DEPC water, primers at 0.3 μM each and 50-100 ng cDNA. Samples were considered positive if they exhibited fluorescence values higher than the threshold before the 35th cycle. Primer specificity was verified by High-Resolution Melting (HRM) in the 50 and 99 °C range. For RT-PCR, the amplification mix included 17.8 μL water, 1X enzyme buffer, 1.8 mM MgCl2, 0.2 mM dNTPs, 0.2 μM primers, 1 U Taq DNA polymerase (Thermo Fisher Scientific, EEUU) and 50-100 ng de cDNA in a final volume of 25 μL. The amplification consisted of an initial 3 min incubation at 95 °C, followed by 35 cycles that included denaturation at 94 °C (30 s), annealing at 52 °C (1 min) and extension at 72 °C (1 min); the amplification ended with an extension cycle at 72 °C for 5 min. Amplicon size was determined by 1.8% agarose gel electrophoresis stained with GelRed 1X (Biotium, EEUU) in a Bio Doc Analyze transilluminator (Biometra, Alemania). Sequences of the RT-qPCR and RT-PCR amplification products were confirmed by the Sanger method using an ABI Prism 3730xl sequencer (PE Applied Biosystems, EEUU) at Macrogen (South Korea). Sanger sequences were deposited in GenBank under accession codes KY823430-KY823445.
Bioinformatic analysis
Open reading frames (ORF) were identified by comparison against known tymoviral sequences using BLASTX with default parameters (scoring matrix: BLOSUM62, word size: 6, gap opening: 11, gap extension: 1) with the exception of E-value, which was set up with a cut-off of 1e-10 (Gish and States, 1993). Functional domains were identified by a search against the Pfam database using the Conserved Domains search at NCBI using default parameters with an expect value threshold of 0.01 (Marchler-Bauer et al., 2017). Protease cleavage sites were identified by finding matches to the sequences reported by Jakubiec et al. (2007) in Turnip yellow mosaic virus (TYMV) using an amino acid sequence alignment with the PFYMV_Antioquia RdRp protein. The 5’ hairpin loops 1 and 2 (HP1-2) and the tRNAval-like motif at the 3’ end of this tymovirus genome were predicted using the minimum free energy algorithm (Zuker and Stiegler, 1981) available in the ViennaRNA Package (Gruber et al., 2008). Evolutionary analyses were conducted in MEGA7 (Kumar et al., 2016) using the Maximum Likelihood (ML) method based on the General Reverse Time Reversible model with 1,000 bootstrap replicates (Nei and Kumar, 2000) using a discrete Gamma distribution with five categories. The evolutionary model was selected with ModelTest (Posada and Crandall, 1998). All Sequence alignments were performed with MUSCLE using gap opening and extension penalties of 1 and 0, respectively, a maximum of 16 iterations, and UPGMA as clustering method (Edgar, 2004).
RESULTS AND DISCUSSION
Next-generation Sequencing
Sequencing of the purple passion fruit transcriptome resulted in a total of 2,169,579,384 nucleotides. After sequence assembly, a contig of 6,088 nt with high sequence similarity to members of the genus Tymovirus (family Tymoviridae) such as PFYMV (84.79%, AF5467107), TYMV (73%, KJ690173), Grapevine fleck virus (GFkV) (73%, JF927942) and Watercress white vein virus (WWVV) (71%, JQ001816) (Table 1). Due to high sequence similarity with the partial RdRp (96.3%) and CP (97.0%) protein sequences of PFYMV, the viral contig from P. edulis f. edulis was named as PFYMV_Antioquia. The PFYMV_Antioquia sequence was assembled from a total of 3,823,738 paired-end reads for an average sequence depth of 123.58x and an abundance of 65,922.13 fragments per kilobase per million reads (FPKM) (Figure 2).
Table 1 Percent nucleotide (nt) and amino acid (a.a.) sequence identities of the PFYMV_Antioquia genome and proteins against selected tymovirus species representing the genus variability. Nucleotide sequence identities are shown for the complete genome and the predicted ORFs encoding the Movement protein (MP), RdRp and CP proteins. Protein comparisons are with respect to predicted amino acid sequences of MP, RdRp, and CP in PFYMV_Antioquia. Dashes represent missing sequences.
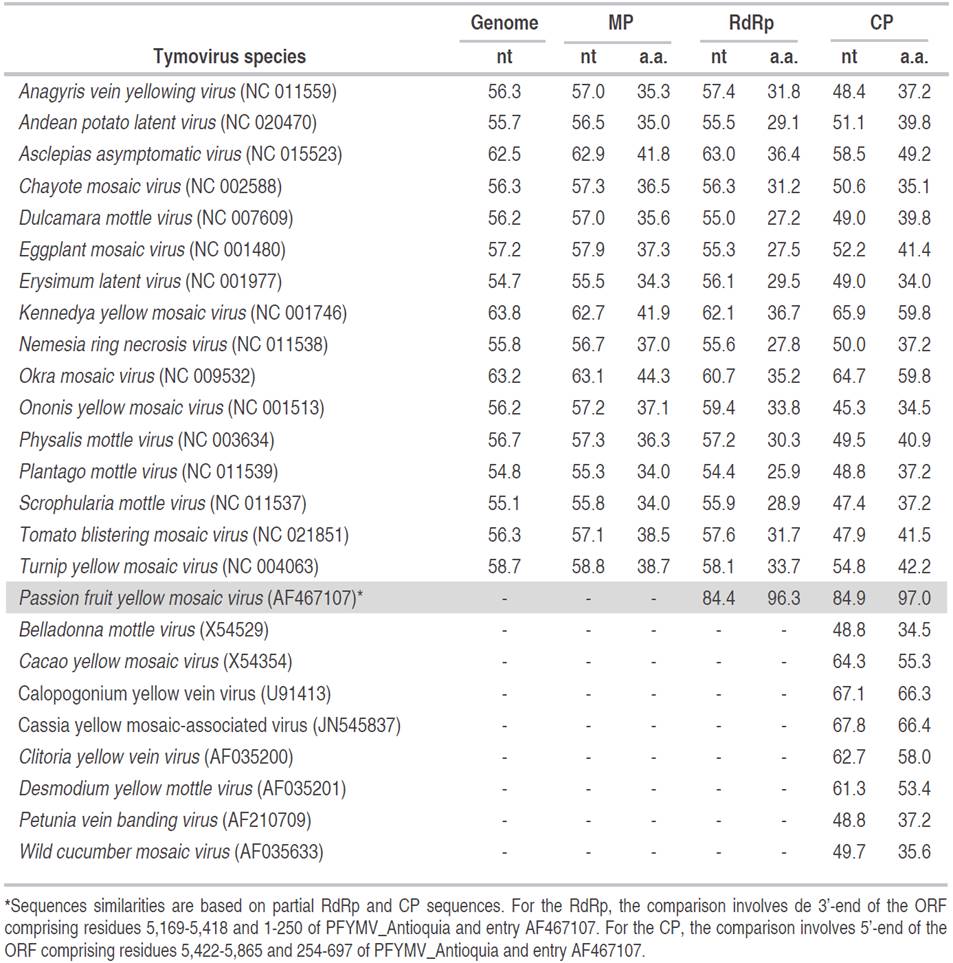
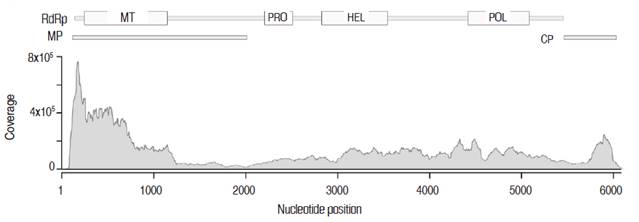
Figure 2 Sequence coverage of the PFYMV_Antioquia genome. The contig was assembled from a total of 3,823,738 paired-end reads for an average sequence depth of 123.58x and an abundance of 65,922.13 fragments per kilobase per million reads (FPKM). The relative position of the ORFs encoding for the MP, RdRp and CP proteins are shown on top and include the location of predicted methyltransferase (MT), protease (PRO), helicase (HEL) and polymerase (POL) domains.
Three ORFs were identified in the PFYMV_Antioquia genome (Figure 3A). ORF1 is located at nucleotide positions 112 to 5,418 and encodes for a putative replication polyprotein of 1,768 a.a. (197.6 kDa) containing methyltransferase (Mtr, pfam01660), helicase (HEL, pfam01443), RNA-dependent RNA polymerase (POL, pfam00978) and protease (PRO, pfam05381) domains. In TYMV, the protease domain mediates cleavage of the 206K polyprotein precursor between the HEL/POL and PRO/HEL domains (Jakubiec et al., 2007); in the PFYMV_Antioquia genome, the polyprotein is predicted to be cleaved at amino acid positions 802 and 1,186 with recognition sequences PKLLGS/S and PRLLGS/H, respectively. ORF2 is located at positions 105-2,006, 7 nt upstream of ORF1, and encodes a movement protein (MP) of 68.6 kDa. ORF3 was identified at positions 5,422-6,003 and encodes the capsid protein (CP, 19.8 kDa). This protein product is predicted to be translated from a subgenomic mRNA transcribed from a viral promoter, or tymobox, with sequence GAGUCUGAAUUGCUUC and identical to that found in TYMV (Schirawski et al., 2000). The tymobox is located at positions 5,383-5,404 within ORF1 and 39 nt upstream of the ORF3 initiation codon (Figure 3A).
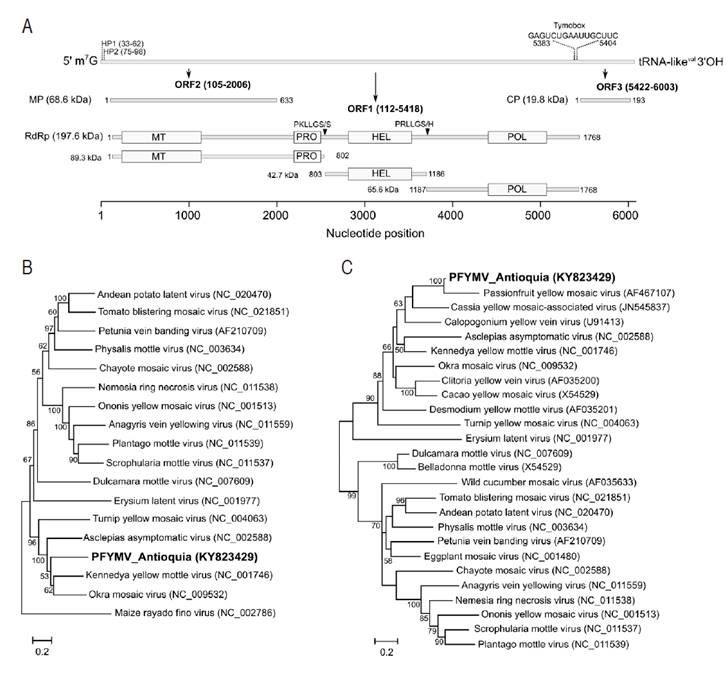
Figure 3 Genome annotation and phylogenetic relationships of the PFYMV isolate from Purple passion fruit in Antioquia. A. Molecular features of the PFYMV_Antioquia genome. The first bar diagram represents the complete genome; nucleotide positions of HP1, HP2 and the tymobox are indicated. The diagrams below are drawn to scale and indicate the position of predicted ORFs and functional domains. Putative protease cleavage sites within the RdRp polyprotein sites are indicated as triangles; B. Maximum-likelihood (ML) phylogenetic tree using available completely sequence tymovirus genomes; C. ML tree using CP sequences. GenBank accession numbers are shown in parentheses.
In agreement with other tymovirus genomes (Giegé et al., 1993; Bink et al., 2003) RNA secondary structure predictions suggests the formation of two hairpin sequences at positions 33-63 (HP1) and 75-98 (HP2) in the 5’ UTR and a tRNA-like structure with a valine anticodon sequence (CAC) in 3’ UTR (Figure 4).
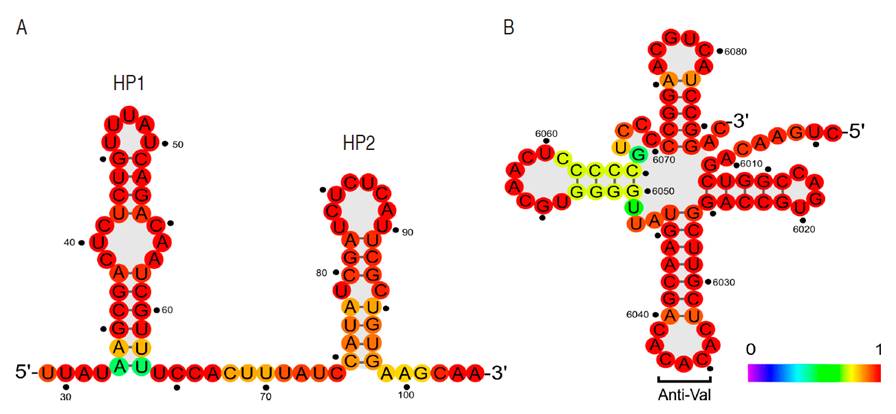
Figure 4 Predicted RNA secondary structure elements in PFYMV_Antioquia. A. Hairpin loops HP1 and HP1. B. tRNAval-like structure at the 3'UTR. Secondary structure predictions were performed using the minimum free energy algorithm package available in the ViennaRNA Package. The color scheme represents the probability for a given base to adopt the secondary structure presented in the diagram.
Maximum likelihood analysis of complete tymoviral genomes, using the marafivirus Maize rayado fino virus (MRFV) as outgroup, confirms PFYMV_Antioquia to be a member of the genus Tymovirus with phylogenetic affinity to a clade comprising Asclepias asymptomatic virus (AsAV), Kennedya yellow mottle virus (KYMV), Okra mosaic virus (OMV) and TYMV (Figure 3B). Analysis of CP sequences confirms that PFYMV_Antioquia is more closely related to PFYMV and forms part of a subclade that also includes Cassia yellow mosaic-associated virus (CYMaV) and Calopogonium yellow vein virus (CAYVV) (Figure 3C). The only PFYMV sequence available corresponds to a 1,115 nt segment of the 3’ end obtained from a passion fruit plant with conspicuous yellow mosaic leaf symptoms in Colombia (Morales et al., 2002). It is important to note that the currently available PFYMV sequence (AF5467107) seem to contain errors in the 3’-end of the CP ORF which could mislead future work on PFYMV (Figure 5).
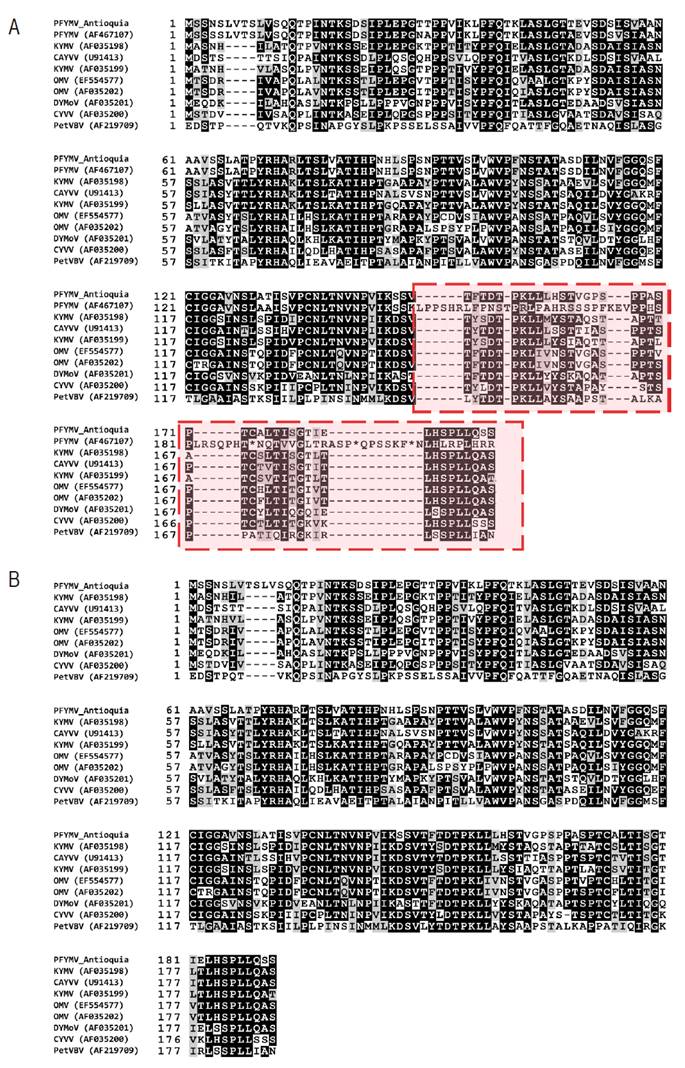
Figure 5 Multiple sequence alignment of the CP region of tymovirus closely related to PFYMV_Antioquia. A. Despite high amino acid sequence identity (97.0%) at the N-terminus (1-143) with PFYMV isolate (AF467107), premature codons are observed at the C-terminus suggesting errors in the deposited sequence (in red); B. Removal of the previously sequenced PFYMV segment (AF467107) reveals that the PFYMV_Antioquia is in good agreement with other previously sequenced tymovirus.
RT-PCR and RT-qPCR
The presence of PFYMV_Antioquia was confirmed in composite samples consisting of either symptomatic (R1S, R2S, R3S, and LCS) or asymptomatic (R1A, R2A, R3A, and LCA) leaves from four purple passion fruit plots in Antioquia using RT-qPCR and RT-PCR with primers targeting RdRp and CP regions (Figure 6). All samples tested positive for this tymovirus. In RT-qPCR, Tymo_F_RdRp1/qTymo_R_RdRp1 reactions exhibited Ct values between 4.16-18.57 and a narrow Tm range (83.6±0.15 °C), while reactions for Tymo_F_CP/qTymo_R_CP had Ct values between 2.33 and 17.72 and Tm of 86.6±0.15 °C, suggesting low sequence variability (Figure 7). Sanger Sequencing of the RT-PCR amplicons and phylogenetic analysis confirmed the presence of the PFYMV (Figure 7).
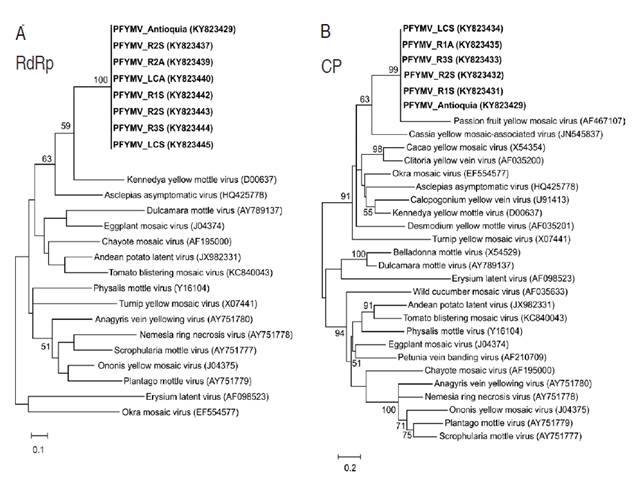
Figure 6 Maximum-likelihood phylogenetic trees of RT-PCR amplicons from PFYMV isolates infecting commercial Purple passion fruit plots. A. ML tree derived from RT-PCR amplification and sequencing of a RdRp segment using primers Tymo_F_RdRp1/Tymo_R_RdRp1. B. ML tree derived from RT-PCR amplification and sequencing of a CP segment using primers Tymo_F_CP/Tymo_R_CP.
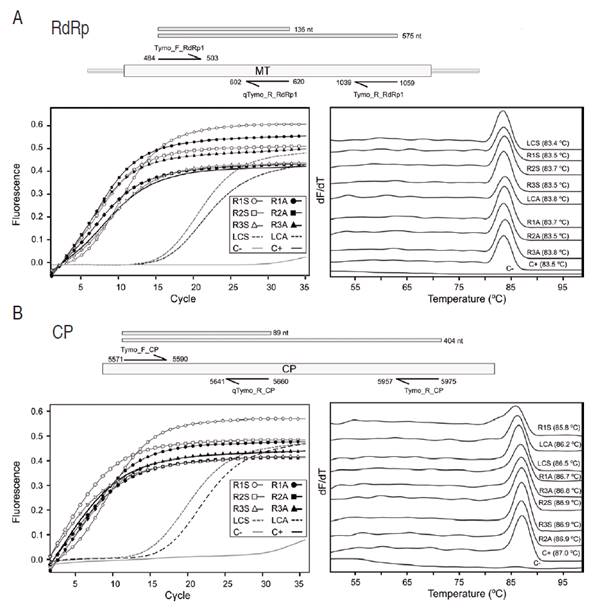
Figure 7 Real-time PCR detection of PFYMV_Antioquia in field samples. Amplifications were performed on symptomatic (R1S, R2S, R3S, and LCS) and asymptomatic (R1A, R2A, R3A, and LCA) bulked leaf samples from commercial P. edulis f. edulis plots in Antioquia with primers targeting either A. the RdRp or B. CP regions. Primers were designed based on the PFYMV genome presented in this work. Bar diagrams on top of each panel represent the relative position of each primer within the PFYMV genome. Panels to the right and left correspond to amplification curves and melting temperature profiles, respectively
This work confirms the presence of a tymovirus infecting purple passion fruit and phylogenetically related to the PFYMV isolate infecting the yellow passion fruit (P. edulis f. flavicarpa). Before this work, the only available PFYMV sequence was obtained from passion fruit plants with conspicuous yellow mosaic leaf symptoms in Colombia. Partial genome sequencing showed this isolate to be distinct from other tymoviruses but with similar pathogenic and antigenic properties to PFYMV infecting Passiflora spp. (Morales et al., 2002). The Brazilian PFYMV isolate was identified in yellow passion fruit plants with symptoms of yellow net, yellow mosaic, and leaf crinkle at Papucaia, in the State of Rio de Janeiro (Crestani et al., 1986). The Brazilian PFYMV isolate has a host range limited to species of the genus Passiflora and can be transmitted by graft or mechanical inoculation and by the chrysomelid beetle Diabrotica speciosa (Crestani et al., 1986). The Colombian PFYMV isolate, on the other hand, was first detected in the passion fruit-producing regions of Antioquia, Caldas, Santander and Valle del Cauca as an isometric virus with serological relationship to Desmodium yellow mottle virus with particle morphology, which induced symptoms similar to tymoviruses (Varón de Agudelo et al., 1992). In contrast to the Brazilian PFYMV, inoculation of the Colombian PFYMV isolate can induce symptoms in other Passiflora species such as Passiflora adenopoda, P. edulis, P. pinnatistipula, P. cuadrangularis as well as solanaceous plants such as Physalis angulata, P. floridiana and P. peruviana (Morales et al., 2002). However, the Colombian PFYMV isolate failed to be transmitted by chrysomelid bettles of the genus Cerotoma, Colaspis and Diabrotica (Morales et al., 2002).
This report, on the complete genome sequence of a PFYMV isolate infecting purple passion fruit, is a new addition to the growing number of NGS sequencing reports on viruses affecting Passiflora species in South America. For example, a recent work by Vidal et al. (2018) showed that blisters, mosaics and leaf deformation symptoms observed in passion fruit crops in Bahia (Brazil) was due to a mixed infection with CABMV and Cucurbit aphid-borne yellows virus (CABYV) (Polerovirus, Luteoviridae). This work is also the first record of CABYV in passion fruit in the world (Vidal et al., 2018). Fontenele et al. (2018), on the other hand, discovered a divergent geminivirus co-infecting a diseased passion fruit plant together with CABMV in the state of Mato Grosso do Sul (Brazil) using NGS sequencing of circular DNA. This new geminivirus has been tentatively named as Passion fruit chlorotic mottle virus (PCMoV) and is the third ssDNA virus affecting Passiflora species together with Passion fruit severe leaf distortion virus (PSLDV), identified infecting this plant in Brazil and Passion fruit leaf distortion virus (PLDV), a proposed species found on Passiflora edulis in the province of Valle del Cauca (Colombia) (Vaca-Vaca et al., 2017). Finally, Jaramillo et al. (2018) reported the complete genome sequence of a SMV strain infecting purple passion fruit in south eastern Antioquia (Colombia) and associated with variegation, rugose mosaics and dark green islands symptoms using RNAseq of symptomatic leaves.
We have given evidence for the existence of a new PFYMV isolate within the genus Tymovirus. The species demarcation criteria within this genus are: overall genome sequence identity of less than 80%, capsid protein sequences less than 90% identical, differences in the 3’-terminal structure, differential host range and serological specificity (King et al., 2012). At the genome level, the PFYMV_Antioquia shares between 54.8% to 63.8% nucleotide sequence identities with available complete genome sequences of tymovirus being most similar to AsAV (62.5%), KYMV (63.8%) and OkMV (63.2%). A comparison of sequences of the capsid protein reveals that the more closely related species are CYMaV and CYVV with 66.4% and 66.3% amino acid sequence identity, respectively. Tymoviruses have a very restrictive host range and can be transmitted mechanically or by beetles of the families Chrysomelidae and Curculionidae in a semi-persistent manner (King et al., 2012).
CONCLUSIONS
Using Next-generation sequencing of the gulupa transcriptome from a bulk sample of leaves from eastern Antioquia, a new isolate of Passion fruit yellow mosaic virus (PFYMV) was identified and its complete genome sequence of 6,088 nt was obtained. To our knowledge, this represents the first complete PFYMV genome in the world. The three ORFs of tymoviruses were identified: ORF1 encodes a replication polyprotein of 1,768 a.a. (197.6 kDa), ORF2 encodes the movement protein (MP), it is located 7 nt upstream of ORF1 and has a predicted molecular weight of 68.6 kDa (633 a.a.) and finally, ORF3 encodes a coat protein (CP) of 19.8 kDa.
Two primer sets targeting the RdRp polyprotein and CP were designed using the PFYMV_Antioquia genome as the reference to confirm the presence of PFYMV by RT-qPCR. Primers were validated using the NGS sample as a positive control and tested in composite samples of both symptomatic and asymptomatic leaves from gulupa plots. Except for the negative control, all samples tested positive for PFYMV. Further work should investigate the host range and vector transmission of PFYMV as well as its serological specificity.