The cultivation of soybean (Glycine max L.) is widely spread around the world, with an estimated surface area of 123 million hectares in 2017 (FAOStat, 2020). Soybean production is an important activity due to the high nutritional properties of grains and the large variety of by-products. The crop has multiple uses for human and animal consumption as well as for industrialization. According to FAOStat (2020), the most important producers worldwide are the USA (119 million tons), Brazil (114 million tons) and Argentina (55 million tons). One of the main destinations of these grains is the export market and the soybean market plays a very important role in the world food consumption. In Argentina, 87% of soybean is exported to China.
In soybean production, pesticides are used to control pests and diseases in the field to increase crop yield, but also during grain storage. To prevent pest attacks, a large variety of pesticides, especially organophosphorus and pyrethroid insecticides, are applied frequently during the storage of grains (Arthur, 1996; Lorini, 2012; Abadia and Bartosik, 2014). Until recently, stored soybeans were treated on the surface of silos (i.e., top dress treatment), to prevent infestations by lepidopteran insects entering clean silos from the upper part (Abadia and Bartosik, 2014). Lorini et al. (2010) identified Lasioderma serricorne as a primary insect pest of soybean and Oryzaephilus surinamensis, Cryptolestes ferrugineus, Ephestia kuehniella and E. elutella as secondary pests. In Argentina, Sitotroga cerealella and Acanthoscelides obtectus have also been mentioned as pests of soybean (Abadia and Bartosik, 2014). These pests cause physical degradation of grains, a fact that imposes barriers to commercialization because of the zero-tolerance policy for live insects in the export market (Arthur, 1996; Lorini, 2012).
In some cases, the doses used during the application of pesticides during storage are higher than those recommended for commercial products, mostly because of the increasing insecticide resistance acquired by insects along the time (Abadia and Bartosik, 2014). As a result, the level of pesticide residues on grains at the moment of commercialization is higher than the maximum residue limits (MRLs) permitted by regulation. Along with the environmental risk, a high level of pesticide residues can affect the quality of the grains and processed products and it may ultimately reach the consumer and cause health hazards. Therefore, to prevent health risks, it is important to monitor the presence of pesticides and regulate their levels in stored soybean grains. To avoid the presence of residues in grains above the MRLs legally allowed (Abadia and Bartosik, 2014; Lorini et al., 2010), pesticides should be appropriately applied, following Good Agricultural Practices. In the European Union, Regulation 396/2005/EC establishes the MRLs of pesticides permitted in products of animal or vegetable origin intended for human or animal consumption (Grimalt and Dehouck, 2016). The MRLs for pesticide residues in soybean grains mostly range between 0.01 mg kg-1 and 5 mg kg-1, depending on the pesticide. The MRLs for dichlorvos, chlorpyrifos-methyl and pirimiphos-methyl are 0.01, 0.2, and 0.05 mg kg-1, respectively (European Commission, 2022).
To measure these low concentrations, highly selective, sensitive and accurate analytical methods are needed in longitudinal experiments to monitor pesticide degradation along time, storage time is a crucial variable. The dissipation dynamics can be modeled by several kinetic models, being the pseudo-first-order kinetics one of the most chosen ones (Fantke and Juraske, 2013). This kinetic modeling allows inferring the half-lives of residues and the acceleration of the residue degradation process. This information is important to determine the residue concentrations that might be found in grains treated with insecticides during storage, and to establish the minimum waiting period required for safe food consumption. The dissipation of insecticide residues has been extensively studied in cereal grains (Afridi et al., 2001; Alleoni and Baptista, 2001; Balinova et al., 2006; El-Behissy et al., 2001; Fleurat-Lessard et al., 1998; Lucini and Molinari, 2011; Pal and Shah, 2008; Sgarbiero et al., 2003, Yu et al., 2014) but is still poorly known in soybean (Lalah and Wandiga, 2002; Zayed et al., 2007; Zhao et al., 2014).
Grains differ widely in the surface characteristics and physico-chemical properties of the tegument, and similar differences may occur among varieties stored under different environments. The lipid content of the grains also affects the retention of pesticides and the waiting period, mainly in oily grains more than in cereal ones since most of the active ingredients are soluble fat. Specific degradation models are thus required to describe pesticide residue degradation for a grain species stored under particular conditions (Fleurat-Lessard et al., 1998). Based on the above, the aim of this work was to monitor the residue levels of dichlorvos, chlorpyrifos-methyl and pirimiphos-methyl applied to stored soybean grains along the storage period, under the standard storage conditions in Argentina.
MATERIALS AND METHODS
Experimental design
The experimental storage assays were performed at the Estación Experimental Agropecuaria of the Instituto Nacional de Tecnología Agropecuaria (INTA), in Manfredi, Córdoba province, Argentina. A completely randomized experimental design was applied, with three repetitions per treatment. The treatments were three insecticides and a control with no insecticide application. The experimental unit consisted of 13 kg of soybean grains, previously confirmed to be free of insecticide residues, and put in a plastic container (20 L) simulating regular storage conditions (20-25 °C daily mean temperature and 40-60% relative humidity) during a 120-day period. Grain moisture content was 14% m.c. Repetitions were three separate lots (13 kg) of treated soybean and five samples over time were taken from each lot of soybean. The organophosphorus insecticides used were dichlorvos (DDVP), chlorpyrifos-methyl+deltamethrin (CPM) and pirimiphos-methyl (PMM), formulated as emulsifiable concentrates. The concentrations (and rates in µg active ingredient per gram of grain) were 100% (20 µg g-1), 14.5% + 0.65% (2.9 µg g-1), and 50% (5 µg g-1), respectively. Grains were spread on a 100-μm polyethylene sheet to obtain a homogeneous insecticide distribution. Insecticides were applied using a hand 1.5 L Giber sprayer (Giber SA, Buenos Aires, Argentina) and grains were vigorously mixed, placed inside the duly labeled containers, capped and stored. The lids were perforated to allow gas exchange. Samples (500 g) were collected from each experimental unit with a bag trier at 2, 30, 60, 90 and 120 days after insecticide application and placed in layered bags, which were then frozen at below -20 °C until analytical residue measurement.
Analytical method for pesticide residue analysis
Samples were processed in the Laboratory of Grain Quality at INTA Manfredi. Grains were milled using Oster blenders equipped with glass jars and stainless steel blades (400 watts). Insecticides were extracted from grains using the QuEChERS (Quick, Easy, Cheap, Effective, Rugged and Safe) technique (Anastassiades et al., 2003; Lehotay, 2007) adapted to dry matrices. The following solvents and reagents were used: acetonitrile (99.98% HPLC grade), toluene (99.5% HPLC grade), sodium chloride (99.7% analytical grade), anhydrous magnesium sulfate (>99.5% analytical grade), deionized water, PSA bonded silica Bulk (Supelco 40 µm) and Discovery DSC-18 SPE (Supelco 40 µm). For validation assays and quality control of the extraction process, the following high-purity analyte standards were used: dichlorvos (99.9%), chlorpyrifos-methyl (99.9%) and pirimiphos-methyl (99.5%) from Sigma Aldrich (USA); and the internal standards ethoprophos (93.1%) (Eto) and triphenylphosphate (99%) (TPP) (Sigma Aldrich, USA). Stock solutions of DDVP, CPM and PMM were obtained by weighing 50 mg of the certified material in a 50 mL flask and stoppering with toluene; the concentration was 1 mg mL-1. Eto and TPP concentration was 2 µg mL-1 using a 25 mL flask. Working solutions (mixture of DDVP, CPM and PMM pesticides) were prepared by combining stock solution aliquots to obtain a final concentration of 40 µg mL-1 of each pesticide. The working solution for Eto had a concentration of 20 µg mL-1, whereas that for TPP had a concentration of 2 µg mL-1. To ensure correct sample processing and analytical performance, a double internal standard was used: Eto for the control of the extraction process and TPP as quality control to isolate variations derived from the chromatographic stage. The acceptance criterion was controlling that the TPP/Eto ratio peaks were within ± 2 standard deviations (SDs) for all the samples analyzed. Moreover, in each set of analysis, were included a quality control (acceptance criterion: recovery percentage between 70 and 120% and residual SD below 25%) and a reagent blank (acceptance criterion: absence of interferences and contaminants).
QuEChERS extraction. Each sample of milled soybean (5 g) was extracted in a centrifuge tube by adding water (10 mL) and acetonitrile (10 mL), and 150 µL of internal standard solution of Eto (20 µg mL-1), 4 g of anhydrous magnesium sulfate and 1 g of sodium chloride. The extract was then homogenized and centrifuged (RCF=5000 g, 5 °C for 5 min). An aliquot (2 mL) of the organic phase supernatant was transferred to a clean-up tube containing 0.3 g of anhydrous magnesium sulfate, 0.1 g of PSA bulk sorbent and 0.1 g of C18, shaken and centrifuged (RCF=5000 g, 5 °C, 5 min). An aliquot of the supernatant (1 mL) was transferred and evaporated to dryness under a stream of nitrogen with the water bath set at 35 °C. The sample was recovered with toluene (500 µL) and shaken; 300 µL was collected and placed in a vial. Then, 50 µL of TPP solution (2 µg mL-1) and toluene (25 µL) were added.
Validations were performed using calibration tests, matrix effect studies and recovery experiments. The fortification levels used were 0.01, 0.05, 0.1, 1, 3 and 9 µg g-1, using a matrix of organic soybean flour; three replicate tests per level were performed. Calibration curves were prepared by artificially contaminating an extract of organic samples and samples not containing matrix with known amounts of analytes. The relationship between the peak area of each analyte and the peak area of Eto was analyzed with respect to analyte concentration. These curves were used to detect the matrix effect and define the linear range.
Recovery experiments were used to calculate recovery, expanded uncertainty (U: a range around the reported result within which the true value can be expected to lie with a specified probability of 95% confidence level), and limits of detection (LoD) and quantification (LoQ). Expanded uncertainty was estimated using the data from the recovery experiment, with Equations 1 and 2:
Where RSD is the relative standard deviation, SDR% is the recovery standard deviation, R%mean is the mean recovery, C is the concentration of the fortified level and k is a statistical quantity (k is ~2 for a confidence level of 95 %). LoD is expressed as three times the SD of the lowest recovery level that meets the accuracy and precision criteria; LoQ was determined with respect to the lowest nominal concentration validated for accuracy and precision parameters. Recovery data were used to assess accuracy; the method precision was calculated and expressed as RSD (European Commission, 2009).
GC-MS analysis
Pesticide residues were determined and quantified using high-resolution gas chromatography-mass spectrometry (GC-MS) at the Food Technology Institute at INTA, Castelar, Buenos Aires, Argentina. The technique was applied using a Perkin Elmer Clarus 600 gas chromatograph coupled to a mass spectrometer with electron impact ion source and quadrupolar analyzer. A programmable temperature vaporizer injection system was used. The initial temperature was 35 °C (4 min), then increased to 290 °C (200 °C min-1 for 2.5 min), and finally decreased to 35 °C (60 °C min-1). The injection volume was 25 μL. Fused silica guard column of 5 m x 0.25 mm (Supelco). Capillary column (Varian, Factor Four VF-5ms cat. CP8944), 30 m x 0.25 mm (id, 0.25 µm) of stationary phase, low bleed poly (5% diphenyl 95% dimethyl siloxane). High purity helium (99.999 %) was used. The initial pressure was 13,789 Pa (3.9 min), reached 172,369 Pa (at 172,369 Pa min-1 for 7 min), and then decreased to 103,421 Pa (at 172,369 Pa min-1), which was maintained until the end of the assay (35 min).
The initial split flow was 100 mL min-1, then closed at minute 3.9 (0 mL min-1) and then set at 20 mL min-1 at minute 10. The initial oven temperature was set at 70 °C (8 min), increased to 170 °C (25 °C min-1), then to 230 °C (5 °C min-1), reaching a maximum of 290 °C (at 20 °C min-1), which was held for 10 min. The transfer line in the detector was held at 290 °C and the collision cell at 200 °C. Chromatography data were obtained by ionization by electron impact (EI+), quadrupole analyzer and monitoring of specific ions (SIM, selected ion monitoring), one ion for quantitation and at least two for qualification for each analyte. For each analyte, the time of relative retention (TRR) of Eto and the ratio of relative abundance relation the quantifier ion and at least two qualifier ions were determined. For DDVP, TRR was 8.4 min, quantifier ion (m/z) 185 and qualifier ions (m/z) 220+145+109; for CPM, TRR was 14.1 min, quantifier ion (m/z) 286 and qualifier ions (m/z) 288+125+290; for PMM, TRR was 14.7 min, quantifier ion (m/z) 305 and qualifier ions (m/z) 290+276+233; for TPP, TRR was 20.9 min, quantifier ion (m/z) 326 and qualifier ions (m/z) 325+77+215; and for Eto, TRR was 17.7 min, quantifier ion (m/z) 126 and qualifier ions (m/z) 139+200+242. The results of the analyses of DDVP, CPM and PMM residues were determined and expressed in micrograms of insecticides per gram of grains (µg g-1). The laboratory performing the analyses of pesticide residues works under a quality system to ensure a consistent and reliable approach with the use of quality control measures.
Statistical analysis
Residue decline (considering a 100% value measured at 2 days after application) and daily dissipation rates (DDR) were calculated. A nonlinear mixed model was adjusted to evaluate the effect of days after application on the degradation of each insecticide. The model included a random effect to induce correlations among repeated measurements of the same experimental unit. The pseudo-first-order kinetic model was the model chosen to fit the dissipation kinetics according to equation 3:
Where Ct is the residue concentration at time t, C0 is the apparent initial concentration or nominal rate and k is the dissipation constant. The parameters estimated were used to calculate the average half-life (HL) of each insecticide in stored soybeans by using Equation 4:
Fisher LSD test was used to determine statistical differences between treatments. Analyses were performed using the statistical software InfoStat (Di Rienzo et al., 2019).
RESULTS AND DISCUSSION
Validation of the QuEChERS+GC-MS methodology
The QuEChERS technique was validated for the insecticides DDVP, CPM and PMM applied to stored soybean grains. The values of average recovery, RSD, expanded uncertainty (%U), LoD (μg g-1) and LoQ (μg g-1), and the regression equations of the calibrations are shown in Table 1. An effect of the matrix was observed for all analytes and the quantification was performed through calibration curves, which were linear for the concentration range studied (R2>0.98). Recovery values in the study range were acceptable for validation in pesticide analysis (70 to 120%), showing adequate reproducibility. These results are consistent with previous validation of QuEChERS in grains (Strada et al., 2021; Mastovska et al., 2010).
Insecticide residues in stored soybean
Residues of the active ingredients studied were found in all the samples treated with insecticide, whereas the control samples were negative for residues of the applied insecticides. Residue levels (mean ± SEM), residue decline (%) and DDR in treated soybean grains at 2, 30, 60, 90 and 120 days after applications are presented in Table 2. The dissipation dynamics for PMM was well fitted with the following first-order kinetic model root function one and a half order, for CPM was half order and for DDVP the fitted models were second order. All insecticide residues decreased with time, as previously reported for these active ingredients in other matrices. In addition, insecticide residues below the LoQ were still found at 120 days (Table 2).
Table 2 Residues, daily dissipation rate (DDR) and residue decline for dichlorvos, chlorpyrifos-methyl and pirimiphos-methyl in stored soybean at 2, 30, 60, 90 and 120 days after application.
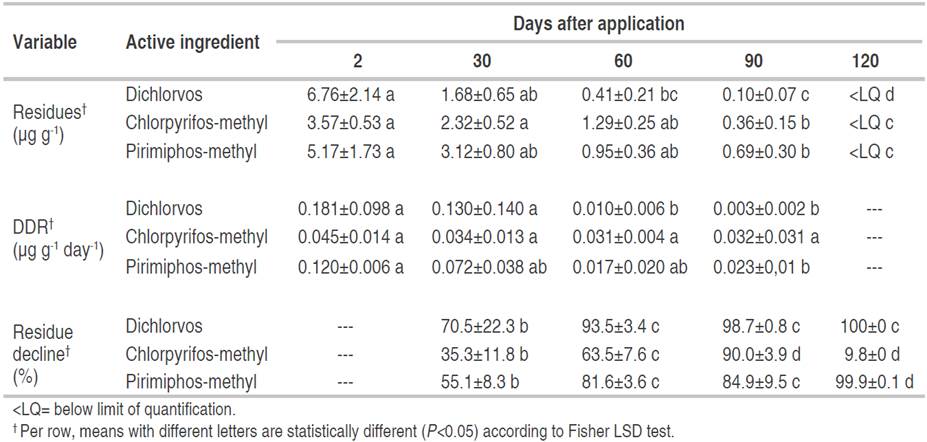
Lalah and Wandiga (2002) reported that residues in oily matrices can be more persistent due to the liposoluble nature of these active ingredients, which generates a biochemical phenomenon of pesticide retention by the grain lipid content. However, in this study, storage for 120 days was found to be effective in reducing residue levels in soybeans.
The magnitude and variation of DDR values depended on each active ingredient and days after application, showing statistically significant effects (P<0.05) for DDVP and PMM (Table 2). For DDVP, the DDR values until 60 days after application were different from the values found at 90 days. For PMM, the DDR values were higher at 2 days after application and only different from those obtained at 90 days. By contrast, the DDR values for CPM were not statistically different. For DDVP and PMM, residues declined by more than 80% with respect to the nominal rates at 60 days after application (Figure 1), whereas for CPM, residues declined by 60%, with the decrease being statistically significant (P<0.05) for all active ingredients (Table 2). By contrast, other studies on the dissipation of insecticide residues in stored grains showed a slow decrease (Arthur, 1996; Holland et al., 1994). For DDVP, some works determined declines in fresh matrices of over 95% at 30 days after application, depending on the initial residue (El-Behissy et al., 2001), whereas Zayed et al., 2007) found residues between 15 and 21% after 30 weeks of storage in soybean grains. With respect to CPM in soybeans, some authors determined a decline of 62% of residues at 112 days, with this decline being statistically significant. (Zhao et al., 2014) and other researchers indicated a 91% reduction of residues 6 months after application (Pal and Shah, 2008). Other studies found that PMM declined by 71% at 120 days after application (Sgarbiero et al., 2003), by up to 75% at 180 days after application in corn (Fantke and Juraske, 2013) and by 65% at 126 days after application in rice (Yu et al., 2014).
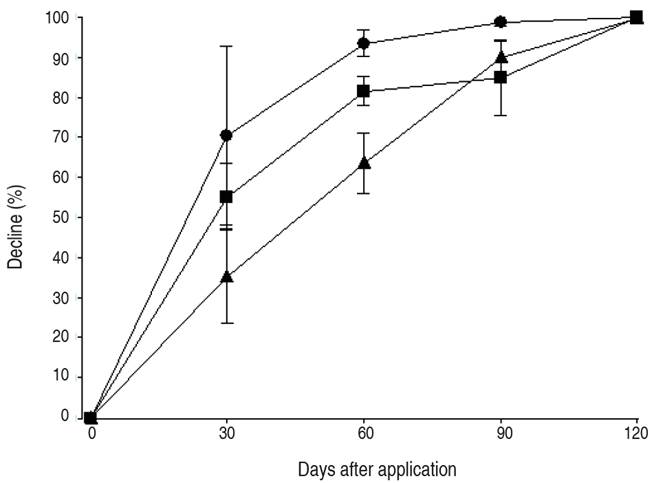
Figure 1 Decline (%) of residues of dichlorvos (dots), chlorpyrifos-methyl (triangles) and pirimiphos-methyl (squares) in stored soybean grains at 2, 30, 60, 90 and 120 days after application.
The dissipation kinetics has three phases: the "stripping phase", which is mainly due to mechanical factors not operating in storage trials (such as wind and rain); the "degradation phase", which is due to physical and chemical factors; and the "persistence phase", determined by residue retention in the material (Coscollá, 1993). In the present study, DDVP showed the highest k values (0.542), followed by PMM (0.023) and CPM (0.018). For DDVP, the first-order kinetics established the highest average k values of the three active ingredients. These high k values may respond to the high volatility characteristic of this insecticide, which provides short-term protection (15 days) (CASAFE, 2020). Thus, first-order kinetics should be used to predict the behavior of residues only at the "stripping phase" of dissipation. For CPM, some authors have found a similar model with a k value of 0.007 (Zhao et al., 2014), whereas for PMM, in a work involving wheat and oats, no degradation kinetics was detected due to the persistence of residues (Lucini and Molinari, 2011).
Regarding the HL of organophosphorus pesticides, previous studies have found high variability (0.7 to 55 days) (Fantke and Juraske, 2013). In this study, HL was found to be greater for CPM (38.8 days) than for PMM (30.1 days) and DDVP (1.3 days); these differences were associated with the physico-chemical characteristics of each active ingredient (Balinova et al., 2006; Lalah and Wandiga, 2002). Reported estimates of HL vary between two weeks for DDVP in wheat (Holland et al, 1994), between 70 and 169 days for CPM (Afridi et al., 2001; Fleurat-Lessard, 1998), depending on the grain moisture, and 99 days for CPM in soybean (Zhao et al., 2014). In addition, a slower dissipation has been reported for PMM, with a HL between 100 and 490 days in wheat (Afridi et al., 2001; Holland et al., 1994) and between 85 and 96 days in corn, depending on the dose (Alleoni and Baptista, 2001). The results of our study suggest that the HLs here observed in soybean are lower than those reported in other studies of soybean grains.
CONCLUSION
Insecticides residues in soybean decreased below 80% of the initial concentration at 60 days after application and below quantifiable levels at 120 days. Residues followed a pseudo-first-order dissipation dynamics [Ct = Co×exp(-k.t)], with the dissipation constant (k) and half-lives being 0.538 and 1.3 days for dichlorvos, 0.018 and 38.8 days for chlorpyrifos-methyl, and 0.023 and 30.1 days for pirimiphos-methyl, respectively. These results allow concluding that, at the recommended dosage, these insecticides are safe for use on soybean grains stored under standard conditions commonly nowadays used in Argentina. The knowledge of the dissipation curves in these three insecticides allows us to predict the post-harvest interval necessary to attain a certain level of residues in order to comply with current legislation.