The high consumption and environmental impacts caused by burning petroleum diesel have driven the search for renewable energy sources. Among these alternatives, biodiesel has stood out. This fuel is obtained from vegetable oils and animal fats. Among the plant species, Jatropha curcas L., or popularly Jatropha, has shown promise as a raw material for obtaining biodiesel (Giraldo Ramírez et al. 2014). This species is outstanding mainly due to its high grain yield and high oil content (Laviola and Dias 2008; Cañadas-López et al. 2020). However, for the plant to reach maximum productivity in grains and oil, it must be properly nourished, with the amount of nutrients at ideal and balanced concentrations.
In the plant, nutritional balance is a very complex and dynamic mechanism due to interactions between nutrients. This is because nutrients behave in different ways, and they can also have different pathways or locations for absorption, which may be exclusive pathways or shared by more than one nutrient (Coskun and White 2022). Also, according to the authors, the interaction between nutrients can also occur due to the difference in ion charges, which may compromise or facilitate the absorption of the other.
There are basically three types of interactions between nutrients: two negative (antagonism and inhibition) and one positive (synergism). Antagonism occurs when the presence of an element decreases the absorption of another element regardless of its concentration in the medium. Inhibition occurs when the presence of an excess element decreases the absorption of another element. Inhibition can be competitive and non-competitive. Competitive is when the two elements compete for the same site (place) of absorption by the plant. Non-competitive inhibition is characterized when the absorption sites are different for each element. Finally, synergism, which occurs when the presence of one element favors the absorption of another element, provides a beneficial effect for the plant (Coskun and White 2022).
In Jatropha, Lima et al. (2011)} and Maia et al. (2014)} found the occurrence of antagonism, synergism and inhibition when studying nutritional interaction. These authors also verified that in addition to the effect on the nutrient content in the plant, the dry matter of both the aerial part and the root system were influenced by the interaction between nutrients.
In extreme conditions in mineral nutrition, that is, when the content of some nutrient is available to the plants in the soil solution at high or very low concentrations, the nutritional interaction can lead to toxicity, as this imbalance will negatively influence the development and consequently, crop productivity (Bell 2022).
The concentration of nutrients in the plant and the grain, oil and protein yields are directly correlated, as nutrients are inputs for obtaining these products by the plant. This source-sink relationship, in addition to the direct effect on productive aspects, also influences vegetative growth (Kirkby et al. 2022).
In view of the possible nutritional interactions that may occur in plants and their effects on productivity, the aim of this study was to verify the existence of a correlation among the foliar nutrient contents in Jatropha, and to investigate the presence of correlation with grain yield, oil, and protein contents.
MATERIALS AND METHODS
The experiment was carried out with 4.5-year-old Jatropha clones grown in the "Diogo Alves de Mello" Experimental Field (latitude 20°45'58" S, longitude 42°52'06" W and 676 m average altitude), at the Federal University of Viçosa (UFV), Viçosa, MG, Brazil. The soil of the experimental area was classified as Red-Yellow Latosol (Embrapa 2018). The climate of the region, according to the Köppen classification, is Cwa, hot and humid, characterized by dry and cold winters with minimum temperatures below 10 °C and maximum temperatures above 34 °C. The temperature during the experimental period ranged from 15.5 to 33.4 °C and the accumulated precipitation was 844.5 mm. The duration of the experiment in the field was three months, and the laboratory analyzes were carried out gradually according to the laboratory's demand.
The plants were grown in 2.5x2.5 m spacing, in a rainfed system and free from weed competition and pest and disease attacks. Chemical fertilization was carried out by chemical analysis of the soil after collecting samples in layers from 0 to 20 and from 20 to 40 cm (Table 1). The recommendation was made according to Dias et al. (2007)} quoted by Silva et al. (2021)}, where 100 g plant-1 of the 20-10-15 N-P-K formula was applied at the time of planting.
Table 1 Chemical analysis of the soil in the experimental area at depths from 0 to 20 cm and from 20 to 40 cm.
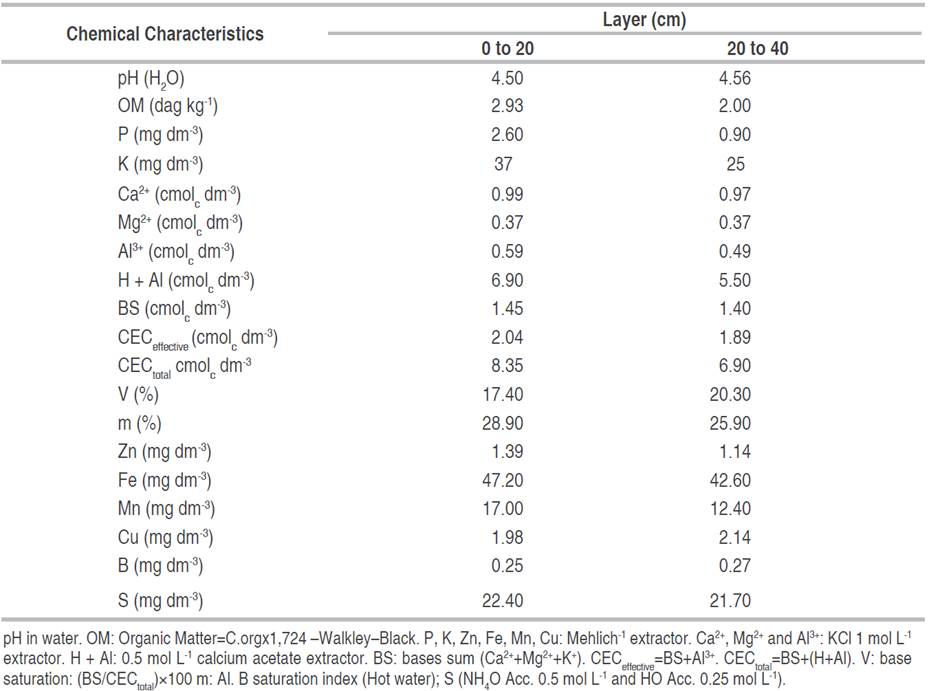
In the first year of planting, 150 g plant-1 of formula 20-00-15 were applied and, in the three subsequent years, in installments, 200, 300 and 400 g plant-1 of formula 20-10-15 were applied, respectively. The soil was corrected annually by liming. During the data collection period liming was not performed.
A randomized block design with four replications was used. The treatments consisted of clones from six J. curcas populations, from the Minas Gerais municipalities of Janaúba (J1, J2, J3, J4 and J5) and Bomfim (B1). Each plot contained eight plants, and for the study the four plants from the useful area were used, thus totaling 96 plants.
Leaf macro and micronutrient contents, grain yield and oil and protein contents in the grains were determined. To determine the foliar nutrient contents, four leaves were collected from each of the 96 plants at random, in three samplings, at flowering, when the fruits were yellow and the last one when the fruits were dry. The leaf for the leaf analysis was chosen according to Kurihara and Silva (2015)} recommendations, from between the 6th and 15th leaf of the floral branch. Fully expanded leaves between the sixth and eighth leaf below the apex free from apparent nutritional deficiency and/or pest and disease attacks were collected. These samples, as soon as they were collected, were sent to the Agroenergy Laboratory at UFV, washed with deionized water and dried in an oven with forced air circulation at 70 °C, until constant weight. Then, the samples were weighed, ground in a Willey mill and subjected to chemical analysis at the Laboratory of Mineral Nutrition at UFV.
To determine the total nitrogen content, the samples were submitted to sulfuric digestion by the Kjeldahl method. To determine the levels of phosphorus, potassium, calcium, magnesium, sulfur, zinc, iron, manganese and copper, the leaf tissues after drying and grinding were submitted to nitric-perchloric digestion. P was determined by the phosphomolybdate reduction method by Vitamin C. K was determined by flame photometry. The contents of Ca, Mg, Zn, Fe, Mn and Cu were quantified by atomic absorption spectrophotometry. The nutrient S was determined by sulfate turbidimetry. All the macro and micronutrient analyses of leaf tissues determined in this study were obtained by the methods presented by Lana et al. (2016)}.
To measure grain yield, all the ripe and dry fruits of the 96 plants in the useful area were harvested; after threshing, the grains were weighed, extrapolating the value obtained to 1 ha. The oil contents in the grains were obtained by the nuclear magnetic resonance method (Oxford Instruments). For this measurement, the grains were initially heated to 40 °C in a block and these samples were placed in test tubes and weighed. The tube has a marking that corresponds to the range of magnetic rays, and its function is to delimit the amount of grains used in the analysis. On average, 10 grains weighing 0.5 g each were used in each sample, totaling a sample of 5 g.
Protein contents were obtained using a near-infrared spectrophotometer (FT-NIR). The sample preparation methodology was similar to the oil content by resonance; the difference was in the reading, as the test tube is placed on top of the lens emitting infrared rays. The FT-NIR also requires calibration prior to performing the analyses.
With the data obtained, the Pearson's correlation coefficient and the Student t test were calculated, as described by Ferreira (2018)}. Thus, in all possibilities, grain yield was correlated with oil, protein, and macro and micronutrient contents in leaves and fruits.
RESULTS AND DISCUSSION
Table 2 shows the Pearson's correlation coefficient (r) between grain yield, oil, protein and leaf macro and micronutrient contents. As for grain yield, statistical significance (P<0.05) was found only for leaf Mg content (r=-0.594). This association can be explained by two hypotheses: the first corresponds to the reduction of Mg levels due to fruit production, since Mg is easily translocated to regions of active growth; the second is the reduction in productivity due to the increase in the concentration of Mg in the tissues. According to Hawkesford et al. (2022)}, the demand for Mg is high in the reproductive growth stage, since the transport of photoassimilates is influenced by the Mg content and, the distribution of carbohydrates between the source and drain organs can be negatively affected if the Mg contents are insufficient for the fruit formation demand.
Table 2 Pearson's linear correlation matrix (r) between the means of grain yields (Yield), oil (Oil) and protein (Protein) contents in grains and macro and micronutrients in Jatropha curcas L leaves.
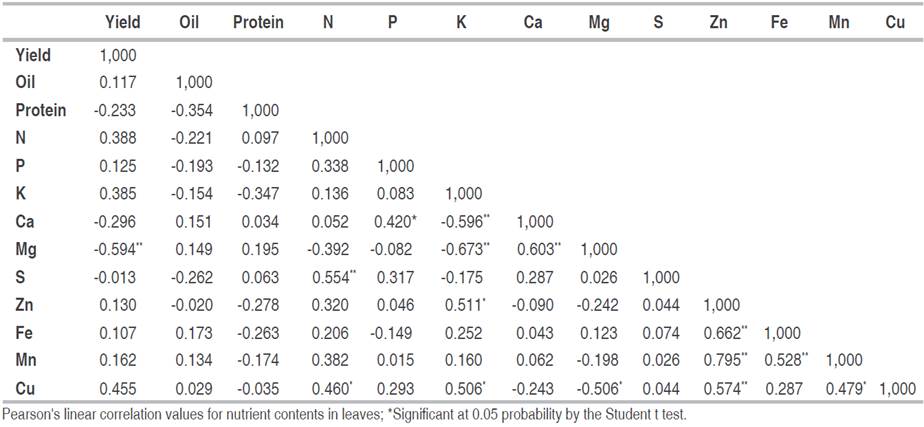
Regarding leaf nutrient contents, significant correlations (P<0.05) by the Student's t test were obtained for the following pairs: (N and Cu); (P and Ca); (K and Zn); (K and Cu); (Mg and Cu) and (Mn and Cu) and at P<0.01 for the following pairs: (N and S); (K and Ca); (K and Mg); (Ca and Mg); (Zn and Fe); (Zn and Mn); (Zn and Cu); (Fe and Mn) (Table 2).
For the levels of N and S, the correlation was positive and moderate (r=0.554) (Table 2). This effect is attributed to the high functional relationship between these ions in amino acid and protein metabolism (Vitti et al. 2018). The relationship between N and S in plant metabolism is so eminent that it is often possible to define the nutritional status of the plant based on the relationship of the contents of these two elements in dry matter (Vitti et al. 2018; Bell 2022). However, a different result was found by Maia et al. (2014)} when analyzing the effect of nutrient omission in Jatropha. These researchers verified that in treatments with no nitrogen in the nutrient solution there was no reduction in the sulfur content in the shoots, when compared to those cultivated in the complete solution. They also observed that in the treatments with sulfur omission in the nutrient solution there was no reduction in the nitrogen content in the shoot.
The correlation between the N and Cu levels was positive (r=0.460) (Table 2). The occurrence of a positive interaction between these two elements was due to the fact that Cu has a strong affinity with the nitrogen atom of the amino group, indicating that soluble nitrogen compounds, such as amino acids, act as Cu carriers in the xylem and phloem (Huang et al. 2021). On the other hand, in citrus, the exaggerated supply of N caused Cu deficiency (Hippler et al. 2017; Huang et al. 2021). With the exception of the biological fixation of legumes, no results were found to explain the increase in N concentration as a result of the increase in Cu levels. However, it should be considered that a fraction of this micronutrient appears to be linked to plastocyanin and some proteins (Dechen et al. 2018). In Jatropha, Maia et al. (2014)} found that leaf nitrogen content was not affected by the omission of copper in the nutrient solution.
The correlation between leaf P and Ca contents was also positive (r=0.420) (Table 2). The increase in P content led to an increase in Ca content in chloroplasts and mitochondria, where, in these organelles, there are about 60% Ca in the leaves (Lima et al. 2018). The increase in foliar P content due to the increase in Ca concentration was due to the increase in P availability through liming (Bell 2022). Lima et al. (2011) evaluated the effect of phosphate fertilization on growth and macronutrient content in Jatropha seedlings and found reductions in Ca levels with increasing concentrations of P. Maia et al. (2014)} observed that in treatments with P omission in the nutrient solution, the calcium content in Jatropha was 19.3% lower than those plants cultivated in the complete treatment of the solution. These same authors also verified that the P content in the shoot was higher than the complete treatment when the Ca was absent from the nutrient solution.
The correlations between the leaf contents of K and Ca, and K and Mg, were significant, but negative (r=-0.596 and r=-0.673, respectively) (Table 2). This effect is attributed to the competitive inhibition existing between the high levels of K in the tissues and those of Ca and Mg, and vice versa (Lima et al. 2018; Bell 2022). The cause of this nutritional imbalance is due to the existence of competition for the same carrier site. Lima et al. (2011) also found the nutritional imbalance between the levels of K and Ca, and K and Mg in Jatropha leaves. These authors found that while the K content in the leaves collected from the vegetative stage to senescence reduced from approximately 37.0 to 14.0 g kg-1, the calcium and magnesium contents increased, respectively, by 5.0 to 48.0 g kg-1 and from 3.80 to 9.80 g kg-1. Cañadas-López et al. (2020)} also found significant interactions between K, Ca and Mg when studying the effects of pruning and fertilization on the production of Jatropha cultivated in a dry environment in the tropical region of Ecuador. These researchers found that potassium fertilization reduced the absorption of Mg and increased that of Ca.
As for the correlations between leaf K contents and the micronutrients Zn and Cu, there was a moderate positive association (r=0.511 and r=0.506) (Table 2). Considering that K is absorbed by many species in amounts higher than those necessary for vegetative metabolism ("luxury consumption"), in this condition plants may have influenced the absorption of cations, as long as they are not competing for the same carrier site (Coskun and White 2022). Thus, it is assumed that the foliar K contents positively influenced the Zn and Cu contents. Regarding the K and Zn interaction, Maia et al. (2014)} found similar effects, as they observed that in treatments with Zn omission in the nutrient solution, K levels in the shoot decreased. These authors did not find correlation between K and Cu contents, they only verified a negative interaction between Cu and Ca contents.
The correlation between Ca and Mg contents was positive and high (r=0.603) (Table 2). This result differs from those found by other authors, as high levels of Ca affect the absorption of Mg, and vice versa (Lima et al. 2018; Bell 2022). This inhibition is related to the similarity in chemical properties, such as valence and mobility, thus providing competition for sites of adsorption in the soil and absorption by roots (Guimarães Júnior et al. 2013; Coskun and White 2022). Considering that high Ca levels reduce Mg absorption, and vice versa, and that in this study the soil of the experimental area had low levels of these nutrients and low pH, the effect of reduction was not verified (Table 1). For Jatropha, Cañadas-López et al. (2020)} found that Ca supply reduced Mg absorption.
For Mg and Cu, the correlation was negative and moderate (r=-0.506) (Table 2). This effect occurred due to the impairment in the absorption of one due to the increase in the other, provided by the strong competition between the cationic elements, indicating that Mg and Cu are antagonistic (Lima et al. 2018). In the literature, no associations were found between Mg and Cu contents in Jatropha.
The Zn and Fe leaf contents also showed positive and high correlation (r=0.662) (Table 2). High Zn levels in plant tissues prevent Fe reduction and may compromise its transport (Dechen et al. 2018). On the other hand, high Fe concentrations cause reductions in Zn mobility, and the presence of iron oxides and hydroxides in the soil induces low Zn mobility in the soil profile (Dechen et al. 2018; Martínez and McBride 2023). Research on associations between Zn and Fe leaf concentrations in Jatropha were not found in the literature Ferigolo (2017)} verified the existence of a negative correlation when analyzing the associations between the Fe and Zn contents in the leaves of Brazilian Ginseng (Pfaffia glomerata (Spreng)). In maize, a negative interaction was also found for leaf Fe and Zn contents, thus indicating the existence of competitive inhibition in the absorption process promoted by excess Zn (Kume et al. 2021).
For the Zn and Mn content, the correlation was also positive and high (r=0.795) (Table 2). This effect differs from those presented by Dechen et al. (2018)} and Martínez and McBride (2023)}, because according to these authors, plants with low Zn leaf concentrations have high Mn levels in the leaves due to Zn fixation and Mn release from the clay structure. In maize, Kume et al. (2021)} found a marked reduction in leaf manganese levels caused by the increase in zinc concentration in the nutrient solution. In the literature, no associations were found between the Zn and Mn leaf contents in Jatropha.
For Zn and Cu contents, the correlation was positive and moderate (r=0.574) (Table 2). There is evidence that these elements are antagonistic (Dechen et al. 2018). In Jatropha, Maia et al. (2014)} did not find a significant association between the Zn and Mn leaf contents.
The correlation between Mn and Fe leaf contents was 0.528 (Table 2). Divergent results were found in the literature, where plants with high Fe contents have low Mn concentrations due to competitive inhibition between them, due to competition for the same absorption site (Coskun and White 2022). The presence of MnO2 oxidizes the reduced Fe to the unavailable ferric form (Dechen et al. 2018). Maia et al. (2014)} found that Jatropha cultivated in treatments with Fe omission showed reductions in Mn levels. In the case of Mn omission in the nutrient solution, only a reduction in the Ca levels in the plant tissues was verified.
The correlation was positive and moderate for Mn and Cu contents (r=0.479) (Table 2). In the literature, a different effect was observed, where the reduction in leaf Cu content due to the increase in Mn concentration occurred due to the adsorption process, provided by the presence of manganese oxide and hydroxide in the soil (Martínez and McBride 2023). As for the reduction in Mn content as a function of the increase in Cu concentration, it appears that this effect is not as influenced as the opposite effect. Maia et al. (2014)} did not find a significant interaction between Mn and Cu levels in Jatropha cultivated in nutrient solution.
Table 3 shows the grain, oil, and protein yields, as well as the nutrient contents in Jatropha leaves. Grain yield was 138.22 kg ha-1, much lower than that estimated by Laviola and Dias (2008)}. The average oil content was 31.38%, very close to the 34% found by Laviola and Dias (2008). The protein content was 20.71%, relatively well below the range of 31 to 35% found by Machado and Silva (2019)}. It is believed that the reason for obtaining lower grain and protein yields is due to reduced micronutrient levels, since the aforementioned studies showed the highest leaf contents only for iron, manganese and copper.
Table 3 Averages of grain yield (Yield), oil (Oil) and protein (Protein) contents in grains and macro and micronutrient contents in leaves and fruits of Jatropha curcas L.
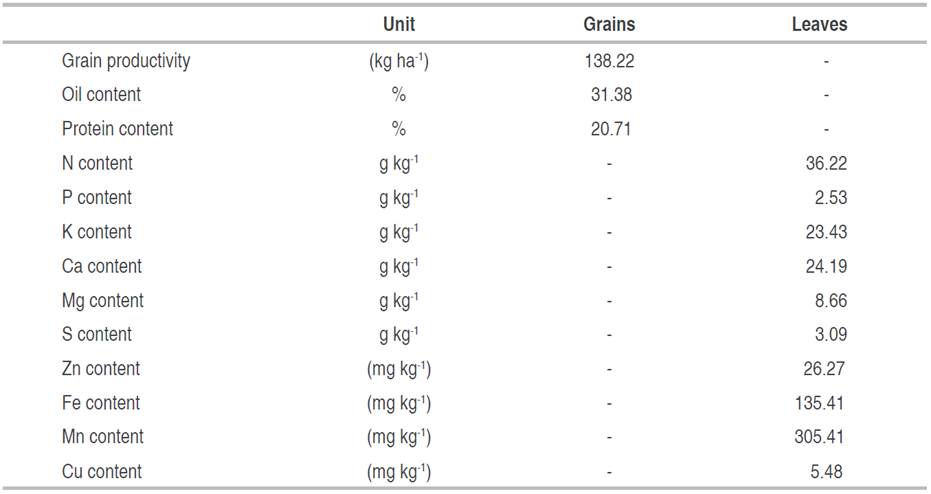
Regarding the nutrient contents in Jatropha curcas leaves, some divergences were observed when comparing the leaf contents obtained by Laviola and Dias (2008)}, as shown below. The nitrogen concentration was 36.22 g kg-1, slightly higher than the 31.40 g kg-1 found by the researchers. The phosphorus content was 2.53 g kg-1, a little lower than the 2.8 g kg-1 found. The potassium content was 58.47% higher than the study used as a reference. The leafs calcium and magnesium content were 78.64% and 55.42% higher, respectively. The S content found was almost triple that found by the authors in the study used as a reference. Regarding the zinc content in Jatropha leaves, a similarity between the values was observed, with 26.27 mg kg-1 in the present study and in the study by Laviola and Dias (2008). In the present study, the levels of iron, manganese and copper were lower than those obtained by the research used as a reference. The reduction was 89.97, 97.10 and 54.80% for the leaf Fe, Mn, and Cu contents, respectively.
CONCLUSIONS
A significant interaction was found between grain yield and leaf magnesium content, showing a negative correlation. No significant correlations were found between leaf contents and oil and protein yields. Significant positive interactions for nutrient content in Jatropha leaves were obtained for the following pairs of nutrients: (N and S); (N and Cu); (P and Ca); (K and Zn); (K and Cu); (Ca and Mg); (Fe and Mn) and (Mn and Cu). Negative significant interactions were obtained for the following combinations: (K and Ca); (K and Mg) and (Mg and Cu). Knowledge of the interactions between nutrients, as well as their responses in grain, oil and protein productivity can be used as a basis for studies on fertilizer recommendation, plant improvement through nutritional efficiency, and studies in the field of biochemistry. Therefore, the mechanisms should be investigated that influence nutritional interactions.