Agriculture, the cornerstone of global food production, relies heavily on water sourced from ground and surface reservoirs. Maintaining the quality of this water is paramount not just for crop yield but also for ensuring the safety of the produce. The microbial content of surface water, due to its dynamic nature, often fluctuates, and at times, environmental runoffs can severely compromise its quality, posing threats to both agriculture and human health (Steele and Odumeru 2004).
In recent decades, a heightened emphasis has been placed on the microbial standards of water utilized in irrigating crops, especially fruits and vegetables. This increased focus stems from recurrent foodborne illnesses related to irrigation water and making a point of concern for public health authorities. Numerous outbreaks, notably those linked with Escherichia coli O157:H7 in spinach (CDC 2006), lettuce (Söderström et al. 2008), and Salmonella Saintpaul in jalapeno peppers (Barton Behravesh et al. 2011), have underscored the critical role that contaminated irrigation water can play in this alarming scenario.
Responding to the growing concerns over waterborne contaminants and the consequent health risks, the U.S. government enacted the Food Safety Modernization Act (FSMA). Beyond the standard regulations, the FSMA emphasizes agricultural water's safety and sustained sanitary quality. This heightened focus presented implementation challenges, especially for small-scale farmers, a fundamental part of local food supply chains. Their dependence on surface water further complicated their compliance efforts, as this water source can be unpredictable in its quality.
Parallel to these challenges, solutions have been sought in science and technology. Zeolites have emerged prominently in this quest, offering hope to those grappling with water purification concerns. These mineral-laden micro-porous rocks, procured naturally and through laboratory synthesis, boast a unique filtering capability. Historically, their prowess in water treatment has centered around removing heavy metals, a testament to their cost-effectiveness, unparalleled ion-exchange properties, and significant absorption capacity (Perić et al. 2004).
The zeolite's ability to seamlessly integrate cationic surfactants within its negatively charged matrix stands out. Cetrimonium Bromide (CTAB), a quaternary ammonium compound, has gained attention in this context due to its surfactant and antimicrobial properties. It disrupts microbial cell membranes and exhibits biocidal activity, making it useful in disinfectants and sanitation in food processing (Tezel and Pavlostathis 2015). Its modified forms enhance contaminant and pathogen removal from water, crucial for safe irrigation practices. However, it is essential to optimize its use to balance efficacy and safety due to its toxicity. When exposed to elevated concentrations of cationic bonding surfactants, zeolites can transform, forming hydrophobic bonds. Research suggests that by introducing cetrimonium bromide (CTAB) to zeolite, it is possible to shift its polarity, transforming it into a robust ally against pathogenic bacteria (Schulze-Makuch et al. 2003). Furthermore, the uniqueness of their ion exchange capabilities can be tailored to target distinct bacterial adversaries. Fresh avenues of research are probing into the prospect of infusing zeolites with specific antimicrobial agents or compounds, thereby amplifying their effectiveness against pathogens like Escherichia coli O157:H7 and Salmonella sp. (Wakweya and Jifar 2023). Combining physical filtration with enhanced antimicrobial activity makes zeolites an effective solution. Such an approach could help farmers comply with the FSMA waters rule and provide an effective solution for purifying surface water, which might be susceptible to intermittent contamination. However, there is limited research exploring the use of surfactant-modified zeolite in managing foodborne pathogens in irrigation water. Therefore, this study aims to demonstrate the effectiveness of surfactant-modified zeolite (SMZ) as a filtration substrate, aiding farmers in securing uncontaminated irrigation water.
MATERIALS AND METHODS
Modification of zeolite using cetrimonium bromide (CTAB)
A natural zeolite, branded as Zeobrite Xtreme®, was supplied by Zeobrite® Corporation. The sample contained more than 71% clinoptilolite. The modification of the zeolite was conducted using cetrimonium bromide (CTAB), a cationic surfactant known for its potential to alter zeolite surface polarity, thereby enhancing its efficiency in water treatment (Margeta et al. 2013). The procedure began by preparing solutions, measured as the mass of CTAB relative to the total mass of the solution, resulting in ratios of 0, 5, 10, 15, 20, 25, and 30% CTAB by mass. Following this, the zeolite was incorporated into each CTAB solution, adhering to a precise mixing ratio of 0.6 mL of CTAB solution per gram of zeolite. This mixture was agitated at a rate of 5.23 rads s-1 for 24 h, ensuring thorough interaction between the zeolite and the surfactant. Subsequently, the mixture was dried in a conventional oven (Thermo Scientific™) set at 150 °C for 30 min to remove excess moisture and achieve the desired modification.
After drying, the surfactant modified zeolite with cetrimonium bromide (SMZ-CTAB) underwent a washing step. It was rinsed with tap water until no frothing was observed, indicating the removal of residual surfactant from the zeolite surface. The washed SMZ-CTAB was then dried in environmental conditions, specifically at 25 °C, within a desiccator chamber containing t.h.e.® desiccant (MilliporeSigma, Burlington, MA) to maintain a moisture-free environment.
Finally, the dried SMZ-CTAB was sieved using ASTM-graded sieves to obtain a particle size distribution between 355 and 710 μm. This size range was selected based on preliminary assessments (data not shown), demonstrating optimal water treatment application performance. The classification of zeolite particle sizes is illustrated in Figure 1.
Microbial culture preparation
To assess the effectiveness of removing bacteria of SMZ-CTAB, three pathogenic strains were chosen: Escherichia coli O157:H7 (ATCC 43895), Listeria monocytogenes ½ a (Lm F4263, CDC, Atlanta), and a cocktail of nine serotypes of Salmonella sp. These Salmonella serotypes encompassed: Albert (AR Bank #0401), Cubana (AR Bank #0402), Stanley (AR Bank #0403), Heidelberg (AR Bank #0404), Senftenberg (AR Bank #0405), Corvallis (AR Bank #0406), Concord (AR Bank #0407), Typhimurium (AR Bank #0409), and Infantis (AR Bank #0410).
All strains were preserved at -80 °C using Tryptic Soy Broth (TSB) from Neogen Corporation, Lansing, Michigan, enriched with 20% glycerol by weight. For reactivation, strains underwent successive transfers in Brain-Heart Infusion broth (BHI) from the same supplier. This was incubated for 24 h at 37 °C until reaching a bacterial concentration of 5-6 log CFU mL-1. To ensure culture purity, specific selective media were utilized. Escherichia coli O157:H7 was grown on Sorbitol MacConkey Agar with Cefixime-Tellurite supplementation (CT-SMAC, Neogen Corporation, Lansing, Michigan). Salmonella sp. was cultivated on Xylose Lysine Deoxycholate Agar (XLD, Neogen Corporation, Lansing, Michigan). Listeria monocytogenes was grown on Modified Oxford Agar (MOX, Neogen Corporation, Lansing, Michigan).
SMZ bacterial removal testing
Laboratory-based studies utilizing scaled zeolite filtration setups aimed to identify the optimal concentration of SMZ for eliminating Escherichia coli O157:H7, Listeria monocytogenes ½ a, and various Salmonella sp. serotypes from Phosphate Buffered Saline (PBS). For this purpose, filtration columns were assembled using a 60 mL büchner funnel containing 100 g of SMZ modified with CTAB concentrations ranging from 0, 5, 10, 15, 20, 25, and 30%. To prepare the filtration columns, 500 mL of sterile distilled water was passed through to compact the SMZ, followed by a thorough rinse with an additional 500 mL of water before each filtration. The filtration process involved running 1 L of PBS, pre-inoculated with the target bacteria, through the SMZ-packed columns. To mimic real field irrigation intervals and monitor potential issues, such as bacterial proliferation within the filter, a 48 h resting period was instituted between consecutive filtrations using the same batch of SMZ. During these intervals, the SMZ was securely wrapped in aluminum foil and stored at a constant temperature of 25 °C.
Bacterial cultures were developed by incubating each strain in 50 mL of BHI for 24 h at 37 °C. Post incubation, they were centrifuged at 4,500 rpm for 10 min. The resulting bacterial pellets were then re-suspended in 1 L of PBS. The initial bacterial concentration before filtration was determined. This inoculated solution was then passed through the SMZ columns of varying CTAB concentrations. Effluent samples were subsequently retrieved to assess bacterial numbers (Figure 2).
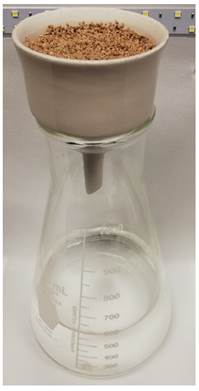
Figure 2 Schematic of the filtration unit featuring a Büchner funnel setup for post-filtration SMZ retention.
For precision, collected samples underwent serial dilution in PBS, spanning 100 to 10-6 concentrations. A volume of 0.1 mL from these dilutions was plated by duplicating their respective selective media. Utilizing the spread plate methodology, these plates underwent a 48 h incubation at 37 °C. Only colonies numbering between 25 and 250 were considered in the final count. The experimental procedure was replicated three times for each bacterial strain. The filtration effectiveness of SMZ-CTAB was evaluated at intervals of 0, 48, and 96 h.
Scanning electron microscopy (SEM) analysis
The morphological evaluation of the samples was conducted using a Quanta™ 3D Dual Beam™ FEG FIB-SEM (FEI Company, Eindhoven, the Netherlands). This microscopy technique integrates the capabilities of a Focused Ion Beam (FIB) with a high-resolution Field Emission Gun Scanning Electron Microscope (FEG-SEM), enabling detailed structural imaging and material characterization at the nanoscale. For the SEM analysis of the SMZ, samples from the 0, 10, 20, and 30% CTAB were used. These samples were mounted on specimen stubs using conductive silver paint to ensure proper grounding. Subsequently, they were sputter-coated with a thin layer of gold to enhance electrical conductivity and prevent electron charging during microscopy.
After sample preparation, they were loaded into the microscope's chamber, which was then evacuated to a vacuum to facilitate electron travel. The samples were then scanned using a focused electron beam, and the emitted secondary or backscattered electrons were detected to generate high-resolution images, revealing the treated sample's intricate surface topography and features. By analyzing these high-definition SEM images, a better understanding of the morphological changes and interactions induced by the varying SMZ-CTAB concentrations on the sample surfaces.
Field testing of SMZ filtration
To determine the effectiveness of SMZ-CTAB under diverse environmental conditions, field tests were performed to factor in extrinsic elements, including native bacterial populations, minerals, organic matter, and natural debris typically present in pond water, all of which can influence pathogen filtration.
Location. The field trial was set up at the LSU AgCenter Botanic Gardens, Baton Rouge, LA (coordinates: Lat. 30°24'32.1012"N, Long. 91°6'21.0132" W).
Crop selection. Strawberries (variety: Strawberry Festival) were selected for the study, given their economic significance and vulnerability in Louisiana. These berries are typically harvested directly from the field to their final packaging, emphasizing the importance of pre-harvest pathogen control (Bruchhaus and Hinson 2005).
Planting and maintenance. Strawberry plants were cultivated following guidelines proposed by the LSU AgCenter (Fontenot et al. 2014). Bareroot plants were arranged in double drill rows, spaced 0.4 m apart, and transplanted during the early fall to anticipate the first mid-October frost. Before transplanting, the field was pre-fertilized with 13-13-13 fertilizer (dosed at 4.5 kg per 30 m-1 row). Post the initial frost, a weekly addition of 12-9-6 fertilizer (at 0.5 L per 37 m2) was introduced via drip irrigation. In the absence of rainfall, supplementary irrigation was administered at 25.4 mm per week.
Weed and pest management. A black plastic mulch of 25.4 µm thickness (sourced from Irrigation Mart, Ruston, LA) was utilized for weed control. Regarding pest management, protective measures were tailored based on specific threats, although two applications of Captan® (dosed at 2 L per 4,047 m2) were routinely applied to manage fungal infections.
Field layout. The entire field spanned an area of 30 x 22 m, subdivided into nine plots, each measuring 1.5 x 3 m (Figure 3). A randomized split-plot design was employed, where six plots were randomly earmarked for each treatment. A buffer zone of 3 m separated each plot to prevent potential cross-contamination.
Frost protection. Given the occasional drops in temperature to below -5 °C during the winter season, the strawberry plots were intermittently shielded using floating row covers from AgFabric® (Vista, California).
Filtration system design and execution
A filtration system was designed using the SandPro™ Model 50D pool filters (GAMe, Scottsdale, AZ). Three different treatments of irrigation water were explored. Firstly, there was the control, which used pond water without filtration. Secondly, the Sand-Filtered Water method was applied, where water was directed through a filter filled with 22.68 kg of 20 g silica sand. This specific silica sand, with its particle size range of 0.45-0.55 mm, was chosen for its optimal filtration capabilities. Lastly, the SMZ-CTAB Double Filtration method was introduced. This involved a two-stage process: initially, water was filtered through the silica sand, and subsequently, it passed through a secondary filter loaded with 11.34 kg of SMZ-CTAB. The results of the previous experiment supported the choice to use a 20% by-weight concentration of CTAB for this field experiment. The waterhead had a diameter of 21.4 mm. After filtration, the water pressure was regulated to 68.95 kPa for the field while the pressure in the tank reached 241.32 kPa. This setup resulted in a flow rate of 2.35 L s-1 throughout the system. To maintain the integrity of the treatments and reduce the risk of unintended interferences, distinct header lines were designated for each irrigation system. This strategy also facilitated an enhanced randomization approach in the field.
The water source was from a pond, notable for its natural contamination from goose droppings. To simulate pathogenic conditions while ensuring safety, the pond water was spiked with a non-pathogenic strain, Escherichia coli ATCC 25922. The inoculation process involved growing a generic Escherichia coli culture in BHI broth at 37 °C for 48 h. The culture was centrifuged to form a pellet and re-suspended in 50 mL PBS buffer. This concentrated bacterial solution was mixed with 950 L of pond water, aiming for a resultant concentration of around 5-6 log CFU mL-1 of generic Escherichia coli. The sampling of the irrigation water was performed after 500 L passed through the system (Figure 4).
Microbial sampling and analysis
Strawberries from each plot were harvested monthly after applying the three irrigation treatments. This ensured the immediate capture of microbial responses to each irrigation method. Simultaneously, post-irrigation water samples from each header line were collected into 100 mL sampling cups. Both water and strawberry samples were promptly refrigerated at 4 °C to preserve the microbial state and prevent any potential growth or injury. Once transported to the laboratory, strawberry samples from each plot were pooled to create a composite sample. Two sub-samples weighing 25 g were extracted from this pooled sample and homogenized in 225 mL of buffered peptone water. This step was crucial, as the buffered peptone water counters the potential effects of fruit acidity on bacterial viability and activity.
The homogenized strawberry and water samples underwent microbial analysis to detect Coliforms and Escherichia coli. The 3M Escherichia coli / coliform Petrifilm™ was employed for this examination, a medium renowned for its precision in quantifying these bacteria. The procedure and interpretation of results derived from the Petrifilm™ strictly adhered to the AOAC™ Official Method 991.14 (AOAC International 1998), which stipulates an incubation period of 24±2 h at 35±1 °C for coliforms, with an additional incubation of 24±2 h for Escherichia coli, ensuring the reliability and reproducibility of the bacterial counts obtained. The experimental setup was repeated annually over two years, with each repetition of the experiment spanning the three-month harvest season, during which samplings were carried out monthly.
Water chemical quality analysis
Comprehensive chemical analyses were conducted at the Soil Testing and Plant Analysis Laboratory, Louisiana State University, to understand potential water composition changes from using SMZ. The assessments were focused on detecting variations in specific elemental concentrations. Parameters examined encompassed Calcium (Ca), Chloride (Cl-), conductivity, Iron (Fe), Magnesium (Mg), Manganese (Mn), Nitrate (NO3 -), Potassium (K), Sodium Adsorption Ratio (SAR), Sulfur (S), and Bromide (Br-). Additional attributes, such as water hardness (based on Ca and Mg levels), alkalinity, and pH, were also assessed to provide a comprehensive profile of the water's chemical quality. Determining alkalinity involved titrating the water sample until it reached a pH=4.5, using 0.02 N of HCl as the titrant. Advanced instrumental analysis was harnessed to ensure accurate determinations of elemental concentrations. Elements like Ca, Cl-, Fe, Mg, Mn, K, S, and SAR were quantified through Inductively Coupled Plasma- Atomic Emission Spectroscopy (ICP-AES), adhering to the guidelines of the Environmental Protection Agency's ICP-AES Method 200.7, Revision 4.4. The concentration of NO3 - was ascertained using a direct reading on the Hach DR900 Colorimeter (Hach, Loveland, CO). Bromide (Br-) levels were determined directly via the HI96716 Photometer (Hanna Instruments, Carrollton, TX).
Statistical Analysis
The experimental design employed was completely randomized. Experiments were replicated three times for the laboratory experiment and the field test twice, and the combined data were analyzed using an Analysis of Variance (ANOVA) through the SAS software package (Version 9.4, SAS Institute Inc., N.C., U.S.A.). Post-hoc tests were performed using the Tukey method to identify differences among treatment groups. Differences were deemed statistically significant at a threshold of P<0.05.
RESULTS AND DISCUSSION
This study was to ascertain the capability of SMZ-CTAB, when integrated into a filtration system, to remove foodborne pathogens from irrigation water. A laboratory approach was employed to determine the most effective concentration of SMZ-CTAB in removing pathogens like Escherichia coli O157:H7, Listeria monocytogenes, and Salmonella sp. from sterile Phosphate-buffered saline (PBS).
Filtration of Escherichia coli O157:H7
The SMZ-CTAB concentrations ranging from 15 to 30% could remove more than 4.0 log CFU mL-1 of Escherichia coli O157:H7 from PBS (Table 1). These results align with previous research on zeolite modifications. For instance, the antimicrobial properties of zeolites, especially those modified with metal ions, have been recognized and validated in several studies (Zampino et al. 2011). A similar study using a Copper-activated Zeolite biofilter achieved a >1.5 log CFU mL-1 reduction in Escherichia coli (Li et al. 2016). They further support the findings that utilizing a zero-valent iron bios and filter coupled with zeolite achieved a significant >6 log removal of Escherichia coli O157 (Ingram et al. 2012). After three filtration cycles (96 h), a resurgence in Escherichia coli counts was observed. The 100 g of SMZ-CTAB was nearing its saturation point post-filtering 3 L of water. Nevertheless, concentrations above 20% of SMZ-CTAB maintained a removal of >4.0 log CFU mL-1 for Escherichia coli O157:H7.
Another observation was the pronounced increase in bacterial counts after 96 h of filtration. This trend hints at the filtration system nearing its operational limit, emphasizing the need for system maintenance or periodic regeneration, echoed by several filtration studies (Margeta et al. 2013). Table 1 outlines Escherichia coli O157:H7 counts in PBS following filtration using various SMZ-CTAB concentrations across different time intervals.
Filtration of Salmonella sp.
A preliminary exploration was executed to gauge the effectiveness of a 30% SMZ-CTAB filtration system in eliminating distinct serotypes of Salmonella from PBS, as detailed in Table 2. Remarkably, there was no statistically significant variance between the serotypes of Salmonella sp. when filtered through 30% SMZ-CTAB. Regardless of the specific Salmonella sp. serotype, bacterial counts plummeted to non-detectable levels when subjected to the higher 30% SMZ-CTAB concentration.
Salmonella sp. is renowned for its ability to survive in various environmental conditions, and its prevalence has been a concern in water sources (Eng et al. 2015). It is essential to highlight that the unique membrane compositions of Salmonella sp. can lead to variances in susceptibility to surfactants such as CTAB. Bacterial strains, due to differences in their outer membrane structures, may show varying levels of resistance or susceptibility to surfactants and disinfectants (Tezel and Pavlostathis 2015). Quaternary compounds, including CTAB, can potentially compromise bacterial cell membranes, resulting in cell lysis (Gerba 2015). Nonetheless, their effectiveness can be strain-dependent, varying based on the bacterial outer membrane composition.
The data (Table 3) revealed patterns, particularly during the initial filtration phase, which indicated a consistent decline in bacterial counts as SMZ-CTAB concentrations increased. Still, the evident differences in microbial counts at reduced concentrations were noteworthy when exposed to a mixture of Salmonella sp. serotypes, as displayed in Table 3. Over time, however, the filtration efficiency decreased, with pronounced reductions at elevated concentrations. Owing to their porous nature and high cation-exchange capacity, zeolites have been explored for their role in water treatment and pathogen elimination (Wang and Peng 2010). This potential is amplified when surfactants modify them. Surfactant-modified zeolites (SMZ) show greater effectiveness in pathogen removal from contaminated water than their untreated counterparts, with the underlying mechanism often ascribed to electrostatic attractions and hydrophobic interactions (Tran et al. 2018). Supporting this premise, a study incorporating fractal silver nanoparticles on zeolites yielded significant reductions in both Escherichia coli and Salmonella sp. populations in sterile water (Guerra et al. 2012). Their outcomes closely mirrored the observations with SMZ modified with CTAB, bolstering the belief that net charges and hydrophobicity remain pivotal mechanisms in bacterial removal. However, while higher concentrations of CTAB show enhanced bacterial removal, too much of it can lead to reduced effectiveness. High surfactant concentrations might create a condition where the surfactant molecules could start associating with each other rather than the bacteria, thereby reducing their antibacterial effect (Li et al. 2016).
Moreover, considering Salmonella sp. inherent hydrophobicity and its negative surface charge (Ukuku and Fett 2002), it becomes evident why higher concentrations, which augment both net charge and hydrophobicity due to increased CTAB bilayer, render them more efficient in pathogen removal. However, it is pivotal to consider other potential factors, such as Salmonella sp. resistance to quaternary ammonia like CTAB, which might also influence removal effectiveness (Tandukar et al. 2013). While the SMZ-CTAB filtration system shows promise in removing various Salmonella serotypes, understanding the interplay of bacterial properties, surfactant concentration, and filter characteristics is crucial for optimizing the system's effectiveness.
Filtration of Listeria monocytogenes
Listeria monocytogenes susceptibility to surfactants, especially quaternary ammonia compounds like CTAB, has been a focal point of interest in recent studies. When exposed to SMZ-CTAB, even at concentrations as low as 10%, Listeria monocytogenes was reduced to non-detectable levels in PBS (Table 4). This high susceptibility can be attributed to Listeria monocytogene's inherent sensitivity to the antimicrobial activity of quaternary ammonia compounds (Aarnisalo et al. 2007). This vulnerability could be related to the absence of resistance genes in Listeria monocytogenes that protect against surfactants like CTAB (Mereghetti et al. 2000).
Salmonella sp. and Escherichia coli O157:H7 revealed a diminishing trend in filtration efficiency for Listeria monocytogenes across subsequent filtration trials. However, it is intriguing that Listeria monocytogenes exhibited a more pronounced reduction in the last filtration than other bacteria. At concentrations surpassing 10 and 15%, CTAB successfully eliminated 4.0 log of Listeria monocytogenes and Escherichia coli O157:H7, respectively.
Table 4 showcases the efficiency of SMZ-CTAB against Listeria monocytogenes over time, with almost consistent elimination at higher surfactant concentrations. Given the importance of waterborne transmission in spreading pathogens, these findings underscore the potential of SMZ-CTAB as an effective barrier against bacterial contamination, mainly when optimal concentrations are utilized (Park et al. 2009).
Morphological Analysis of Bacterial Response to CTAB Concentrations
The SEM FIB images (Figure 5) elucidated the extent of the effect of different concentrations of CTAB on bacterial cells. Most bacterial cells displayed intact cell membranes at 0 and 10% CTAB. However, with 20% SMZ-CTAB, bacteria had suffered extensive cell membrane damage due to the bactericidal action of the quaternary ammonium compound. No bacteria were visible at the higher concentration of 30%. This suggests that a filtration device composed of SMZ at 20% CTAB would effectively retain bacteria until they are inactivated. In contrast, a concentration of 30% might release viable but non-culturable bacteria into the system. The bactericidal action of quaternary ammonium compounds, such as CTAB, primarily targets the bacterial cell membrane, causing disruptions and eventual cell death (Wessels and Ingmer 2013).
Strawberry field testing
Based on the results of laboratory-scale experiments, 20% CTAB demonstrated high effectiveness in bacterial removal at 96 h and was therefore selected for field trials. The results revealed that the SMZ-CTAB filtration device removed over 4.0 log CFU mL-1 of coliform and Escherichia coli (Table 5) over two production cycles, maintaining its efficiency. Notably, merely using sand in the filtration system exhibited no discernible bacterial removal. Only the modified zeolite filtration device ensured that pond water met optimal irrigation levels.
Table 5 Coliform and Escherichia coli removal in pond water filtered through sand and 20% SMZ-CTAB: Petrifilm method.
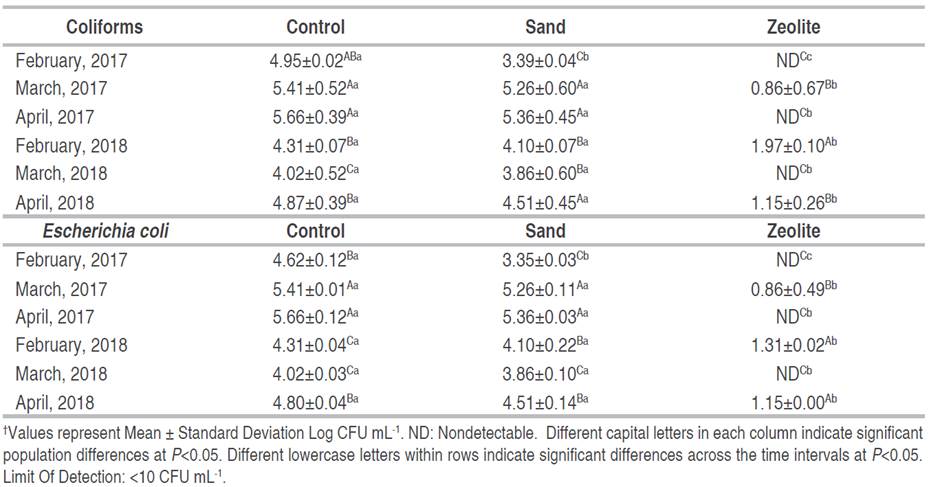
The contamination of irrigation water with pathogens can have severe implications, especially for crops consumed raw, like strawberries (Suslow et al. 2003). The safety of irrigation water is essential for food safety and agricultural systems' overall productivity and sustainability (Havelaar et al. 2015). The results indicated that the levels of Escherichia coli were effectively reduced by the SMZ-CTAB treatment to below the recommended threshold of <126 CFU 100 mL-1 (Weller et al. 2017).
Strawberry samples from the initial 2017 production cycle were subjected to analyses for Escherichia coli and coliform counts (Table 6). Notably, the results revealed no statistically significant disparities among the treatments. This outcome may be attributed to the prevalent practice in Louisiana of utilizing plastic covers during cultivation. Such measures exclude direct contact between irrigation water and the fruit. Nevertheless, the region's susceptibility to flooding accentuates the imperative of employing uncontaminated water sources for irrigation (Park et al. 2009). The potential for pathogenic contamination due to flooding can lead to outbreaks, affecting public health and the agricultural economy.
Water Chemical Quality Analysis
A comprehensive view of the chemical profile of irrigation water post-filtration for 2017 and 2018 in Table 7 displays how distinct parameters like alkalinity exhibit variations when processed through the zeolite system, potentially highlighting the ion-exchange properties of SMZ-CTAB. Notably, bromide levels remained low, suggesting no noticeable leaching of bromide post-filtration. However, the analysis of variance shows that parameters between the two years were not statistically significant for any treatments (P<0.05). While zeolite filtration demonstrates the potential to modify the irrigation water's chemical attributes, these changes remain consistent across the years studied. Such consistency suggests that other external or design-related factors influence the slight variations observed. Further research would be beneficial to determine the long-term impacts of zeolite filtration on water quality and its subsequent influence on agriculture.
CONCLUSIONS
The Food Safety Modernization Act (FSMA) underscores the imperative of maintaining stringent water quality standards in agricultural and food processing settings. In alignment with such directives and the objective of this study, this research evaluated the effectiveness of surfactant-modified zeolite (SMZ) modified with cetrimonium bromide (CTAB) to remove bacteria. These findings indicate that using CTAB (at 20 and 30%) will significantly have bactericidal effects, compromising the integrity of bacterial cell membranes. Such observations are corroborated by SEM FIB imaging, highlighting the impacts of CTAB on bacterial morphology. The filtration media performance across two years reaffirms its reliability. The SMZ-CTAB filtration system emerges as a potential candidate to meet the water quality directives outlined by the FSMA. As the global community grapples with mounting challenges in ensuring food safety, the findings of this experiment provide a pathway that warrants further exploration in the context of scalable water treatment solutions for food production.