Microorganisms represent less than 5% of the soil organic matter (SOM). Still, they are the most active and essential living component, responsible for the dynamics of organic residue decomposition, mineralization of nutrients, degradation of xenobiotic compounds, soil aggregation, and the development of symbiotic relationships with plants to promote their growth (Dalal 1998; Kaschuk et al. 2010). Furthermore, they are essential for the soil to perform its multiple productive and environmental service functions since they participate in almost all the known metabolic reactions of the soil (Voroney and Heck 2015; Paolini 2018).
Different soil uses and management systems can differentially affect the microorganisms that inhabit it, thus modulating the ecosystem services they provide. It is also known that this biological component of the soil responds quickly to environmental changes as an indicator of high sensitivity to edaphic changes produced by management practices (Di-Ciocco et al. 2014).
Many types of agricultural land use have been identified to cause a drastic decrease in total soil microbial biomass and a reduction of total SOC. The latter occurs due to the decomposition of the SOM by microorganisms and is strongly affected by the chemical and physical conditions of the soil (Kaurin et al. 2018).
Dexter (2004) pointed out that the biological status of soil depends very strongly on the prevailing physical conditions, mainly due to poor water infiltration, poor aeration, and poor conditions for root growth. These are also symptoms associated with decreased biological activity in soils. Cui and Holden (2015) found that soil structure strongly correlates negatively with soil respiration and enzyme activity, indicating a decline in microbial activity with a worse soil structural quality.
For this reason, various studies have evaluated the activity of microorganisms in different types of soil use and management. Morais et al. (2016) assessed the effects of crop systems and coverage on soil microbial communities, observing that the number of microorganisms on the soil surface was higher associated with zero tillage. Zagal et al. (2002) evaluated soil microbial activity in different annual crop rotation systems, registering a significant correlation between microbial activity and the rotation system with soil management that enhanced the organic carbon content.
It is known that an essential function of soil is the conduction of water and air, which is necessary for the remarkable growth of roots and microbiological communities. Therefore, microbial processes in soil largely depend on pore structure and soil compaction conditions (Morais et al. 2016). Ishak et al. (2016) found that soils with low compaction contain more soil pores predominated with macropores that enhance soil capacity to retain soil water and provide soil oxygen diffusion, thereby increasing microbial activity. However, in the compacted soils, low microbial activity was observed.
Valenzuela and Visconti (2018) found that the soil bulk density, the content of clays, and the content of silts are physical characteristics that can be used as variables to explain the relationship of physical soil conditions with the stabilization processes of the SOM; therefore, since the decomposition and stabilization of the SOM is a process carried out by soil microorganisms, physical soil conditions are involved (Six et al. 2004).
Recent studies have emphasized that biological characteristics of the soil, such as the total organic carbon, the carbon of the microbial biomass, and the microbial quotient, can gradually increase or decrease depending on the use and management of soil, which is essential for the evaluation of a sustainable agricultural system (Assis et al. 2017; Zornoza et al. 2018). Indeed soil management practices can affect soil microorganism communities (Brevik et al. 2015), and concerning soil physical conditions. Ishak et al. (2016) observed that microbial activity was higher in uncompact soils and decreased as soil compaction increased. In addition, the amount of soil microbes expressed as microbial biomass may be influenced by the amount of soil organic matter, climatic factors, land use, and physical-chemical characteristics of soil (Iglesias 2009). That is the reason why studies must be carried out in those fields.
In this context, this study was conducted to assess the relationship between physical soil conditions and microbiological activity under different agricultural use and management systems in the warm tropical climate of Colombia.
MATERIALS AND METHODS
Study area
The study was conducted in the Zulia River Irrigation District in the rural area of Cucuta, Colombia. The site has a mean annual temperature of 29 °C, the mean annual precipitation is 1,900 mm, and the climate is classified as Tropical Dry Forest, according to Holdridge (IGAC 2006a). On the same farm, located at 8°10' 22.4" N and 72°29' 03.5" W, and at an altitude of 80 meters above sea level (masl), three different soil agricultural use and management fields were selected, delimited, and characterized according to their uses: irrigated rice (IR) cultivation, native forest with cocoa (FC), and pasture (P). According to IGAC (2006a), the selected fields are in a soil classified as Typic Udifluvents.
The management system of the plot with irrigated rice IR involved cultivation with mechanized tillage in flooded soil (muddy soil) and flood irrigation with periodic water replacement. The sowing was manual and broadcasted with a pre-germinated seed of the Fedearroz 2000 variety; chemical fertilization was broadcasted manually and based on urea, diammonium phosphate, potassium chloride, and complete fertilizer, to contribute 150 kg ha-1 of N, 80 kg ha-1 of P and 120 kg ha-1 of K. Selective pre-emergent and post-emergent herbicide applications and chemical pest and disease controls were performed by back sprayers manually. The harvest was mechanized, and the grain was retrieved from the field in packages transported by a tractor. After the harvest, the straw residues were burned.
The native forest with cacao FC involved agroforestry management of cacao trees (Theobroma cacao) with a shade of tall tropical trees such as Ceiba (Ceiba pentandra), Jobo (Spondias mombin), Apamate (Tabebuia rosea) and the Higuerón (Ficus luschnathiana). The management was reduced to maintenance pruning of the cacao once per year and harvesting of pods twice per year. No fertilizers, herbicides, insecticides, or fungicides were applied.
The plot management with pasture P involved star grass paddocks (Cynodon nlemfuensis) destined for grazing cattle, with weed control manually and without chemical fertilization or irrigation.
Soil sampling
The study consisted of a single-factor experiment with the use and management system as variables (three treatments). Three composite soil samples were taken in each treatment with two repetitions. A 1-hectare plot was allocated for a systematic soil sampling for each treatment. This was carried out, marking three equidistant points on a 100 m long diagonal transept. At each sampling point, a cross with 2 m on each side was marked on the soil to extract subsamples from the intersection, and at the ends of the cross, thus, a composite sample of surface soil (0 to 10 cm depth) was conformed. From the composite soil sample, 1 kg was taken and stored in plastic bags for refrigeration (4 °C) at the laboratory. Also, two undisturbed samples at the same soil depth were taken at each sampling point using metal cylinders 5 cm high and 5 cm in diameter.
Evaluation of soil physical, chemical, and biological properties
To record the differences between fields on soil sampling conditions, the surface soil temperature was measured using a mercury thermometer, and the volumetric moisture content was measured with a dielectric sensor. The mechanical resistance to penetration (MRP) was measured at the surface soil (0 to 10 cm depth) at the same time of soil sampling, using a hand penetrometer, with a conic point of 2 cm2. At the laboratory, the physical properties of the soil were determined, such as particle size analysis by the agitation and sedimentation method of modified Bouyoucos (IGAC 2006b). The Bd and the total porosity were measured by the Uhland-type metal cylinder method, where the core soil sample is saturated with water in immersion trays before weighing at the saturated condition and then dried in the oven at 105 °C for dry soil weight. The MPt was measured as the porous space of diameter >30 µm; using the tension table method, which consists of a porous membrane plate to which suction is applied through a column of water suspended at 100 cm (-10 kPa) (Pla 1983; Pla 2010). The pH and electrical conductivity (EC) were measured in a soil/water suspension (1:1). The cation exchange capacity (CEC) was determined by the standard method of ammonium acetate 1 N and pH=7 (IGAC 2006b).
Within 48 h of soil sampling, the refrigerated composite soil samples were used to determine the carbon of the C-MB by the substrate-induced incubation method modified by Lozano et al. (2005). Also, the oxidable SOC was determined on the composite soil samples by acid digestion with the potassium dichromate oxidation method (IGAC 2006b).
Evaluation of the abundance of bacteria and fungi in the soil
Within 24 h of soil sampling, the refrigerated composite soil samples were used to determine the total cultivable populations of fungi and bacteria in the studied soils 10-1 dilutions were prepared by weighing 10 g of soil and adding sterile saline solution (0.85% NaCl), which were homogenized by vigorous stirring on an orbital shaker for 10 min. A preliminary test with serial dilutions from 10-2 to 10-6 was conducted to select the final dilution to use in round Petri plates of microbe cultures (Vanegas et al. 2013).
Cultures on the Petri plates were made from 0.1 mL of each selected dilution and placed on the surface of the specific growth culture medium. Nutrient agar (NA) was the bacteria-specific medium (dilutions 10-3, 10-4, and 10-5) and Rose Bengal agar for fungi whose selected dilutions were 10-2, 10-3, and 10-4. For each soil sample, this was done in triplicate. The culture plates with nutrient agar for bacterial growth were incubated for 24 h at 37 °C, and those of Rose Bengal for fungal growth were incubated between 5 to 7 days at 28 °C. Quantification was performed using the plate count technique and was expressed as the decimal logarithm of the colony forming units (CFU) per gram of soil (Calvo et al. 2008). Finally, the microbiological quotient was calculated as the ratio of C-MB to the SOC (Paolini 2018; Kaschuk et al. 2010).
Data analysis
Data were analyzed using Statgraphics Centurion statistical version XVI software using an analysis of variance (ANOVA) after checking the normality and variance homogeneity assumptions. In case of violation of these assumptions, the data were analyzed using the Kruskal Wallis non-parametric test. In the comparison of means, the least significant difference test was applied at a confidence level of 0.05%. Additionally, correlation graphs and Pearson's correlation coefficient were used to establish the degree and type of association between variable pairs. A multivariate analysis was made to interpret each variable's variance and influence.
RESULTS AND DISCUSSION
Physical and chemical properties of surface soil
The particle size analysis results in the studied fields (Table 1) show that soils are of fine texture; the plot with FC has a silty clay loam texture, while the soils with IR and P have clay texture.
Results obtained from the physical properties of soils in each use and management system (Table 2) show limitations due to compaction; given that soils in IR and P had high soil Bd values according to their texture (Dexter 2004). The lowest Bd was observed in the FC, with a value that can be by the texture. Regarding the Pt, it is observed that the soil of the three use and management systems shows a porous space reduction (Table 2). The IR and P presented MPt values below 0.10 (10%), which indicates physical degradation by poor aeration (Dexter 2004; Pla 2010). A different situation was observed in the soil under the FC system, where the MPt was 0.12 (12%), which indicates a favorable physical condition.
The volumetric water content registered in the surface soil was near saturation in the IR, while the FC had a 21.3% lower soil moisture in comparison with IR, while the driest soil was P with 32.9% less water content. The surface soil temperature was the same for IR and P with a value of 39.3 °C and was slightly lower in the FC at 38.7 °C.
Using the Bd, MPt, and MRP as indicators to evaluate soil compaction in each plot, the soil of P revealed physical degradation because of high Bd, low MPt, and high MRP. Also, the soil of IR can be considered compacted since Bd and MPt are out of normal ranges as considered by Pla (2010). Although the soil of IR presented the lowest MRP, it is understood that the high moisture content and high clay content influenced this physical behavior.
However, the evaluation of the soil's physical properties evidenced a degradation in the soil used with IR because of management practices such as tillage with flooding, which generate compaction of the soil, resulting in high Bd and low MPt (Table 2) as other studies have reported (Ruiz et al. 2005; Valenzuela et al. 2015). The soil in P uses evidenced compaction generated by the trampling of animals during the grazing process (Medina 2016).
The analysis of the chemical properties of the soil (Table 3) revealed that the pH was higher in the FC (5.77), which indicates a moderately acidic soil condition. The pH is classified as strongly acid for the IR and P, with values of 5.47 and 4.76, respectively.
The electrical conductivity results in the soil of the three use systems showed values between 34.53 and 166.73 µS cm-1 (Table 3), indicating no salinity problems.
Impact of soil use system on the activity of microorganisms
The results of the variables measured to know the impact of each soil use system on the activity of the microorganisms are presented in Table 4.
The statistical analysis of the results for SOC expressed a statistically significant difference between the means, resulting in the IR soil having the lowest content of SOC and the P and FC soils constituting a homogeneous group with higher SOC values.
The SOC content was statistically higher in the P and the FC (Table 3). In the P system, it may be due to adding organic matter from animal manure and residues from pasture. In the FC case, it may be due to the contribution of litter from shade trees and cacao trees. Furthermore, in these two systems, there is no soil disturbance by tillage and no residue burning that could negatively affect the SOC (Vimal et al. 2017; Valenzuela and Visconti 2018). According to the C-MB analysis, a statistically significant difference between the means was found, resulting in the soil in IR having the lowest content of C-MB and the soils in P and FC constituting a homogeneous group of higher content in C-MB. The C-MB quantifies the global quantity of microorganisms present in the soil (Sánchez et al. 2005), and the results show that the system of use in P and FC presented significantly higher values of C-BM compared to the IR (Table 3). This can be associated with the higher SOC content since it contributes to increasing the activity of microorganisms; by constituting a source of energy and nutrients for microorganisms, which contributes to their development and higher microbiological activity (Sánchez et al. 2005; Anderson and Domsch 1989). It also confirms what Paolini (2018) affirms, that microbial biomass is associated with greater incorporation of organic matter and nutrients into the soil.
Additionally, the results coincide with Núñez et al. (2012), who reported high C-BM values for grassland with intensive grazing (297 mg C kg-1) and grassland with light grazing (291 mg C kg-1), indicating that the grazing systems improve the biological activity of soils. The ANOVA did not show a significant difference between the soil use system on the count of bacteria (Log CFU bacteria) or fungi (Log CFU fungi). However, the use of IR reported the lowest value in the bacteria and fungus count. In comparison, using P reported the highest value in the count of bacteria and the count of fungi (Table 3).
On the other hand, this coincides with the study by Hernández et al. (2013), who found a decrease in the bacterial populations present in the soil in different cultivation systems with the application of agrochemicals.
Hernández and Lizarazo (2015), when studying populations of soil bacteria in conserved areas of Paramo and cultivated soils, reported an average of cultivable bacteria of 8.90 log CFU g-1 of soil, and 3.60 log CFU g-1, respectively. Thus, the importance of soil use and management over the quantity and diversity of microbial populations was demonstrated.
The results of the fungus count allowed observation that the soil in use with IR had the least amount of cultivable fungi (Table 3). This behavior could be associated with the compaction of the soil and the lower SOC content, which affects the survival of the microorganisms (Calvo et al. 2008). When observing that the use of P showed a more significant amount of fungi, it is related to the fact that the largest abundance of fungi is found in the superficial layers of soil, where the microclimate, environment, and availability of nutrients are favorable for their development and growth (Lavelle and Spain 2001).
In the case of the MQ, the analysis of variance allowed us to find a significant effect of the soil use system on this parameter, resulting in the use of IR showing the lowest microbial quotient. In contrast, the uses of FC and P showed a higher quotient, confirming that the IR use and management system has degraded soil microbiological activity.
Relationship between soil Physical conditions and soil microbiological activity
The evaluation of the relationship between the physical conditions of soil and the parameters of microbiological activity, using the correlation graph between Bd and C-MB (Figure 1), reveals that the C-MB decreased while the Bd increased, which means that these two parameters are inversely proportional; because an increase in Bd implies that the soil is more compact, and therefore the oxygen supply to microorganisms is decreased, (Ishak et al. 2016; Visconti and Valenzuela 2023).
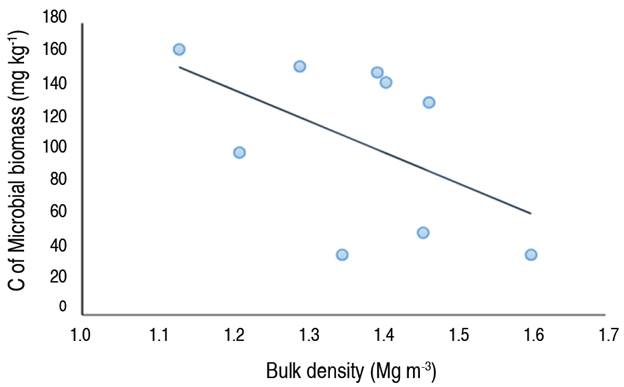
Figure 1 Correlation graph between the bulk density (Bd) and the carbon of microbial biomass (C-MB) (Pearson correlation=-0.53).
In the relationship between the macropores and the CFU of bacteria (Figure 2), it is observed that increases in soil macropores corresponded to slight increases in the number of colonies forming units of bacteria, indicating that they are directly proportional since soil macropores allow an increase in soil aeration, thus increasing the availability of O2 for bacteria (Cui and Holden 2015).
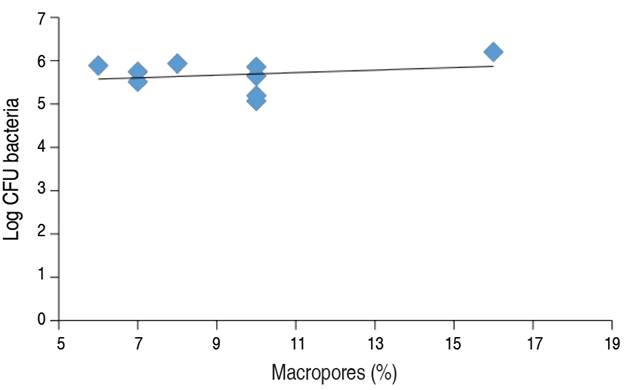
Figure 2 Correlation graph between Macropores and Log of CFU of Bacteria (Pearson correlation= 0.55).
Morugán-Coronado et al. (2019) pointed out that changes in soil moisture affect soil microbes' survival. In this case, the relation between soil moisture and the CFU of Fungi (Figure 3) shows that increasing the soil moisture content slightly decreases the number of CFU of Fungi.
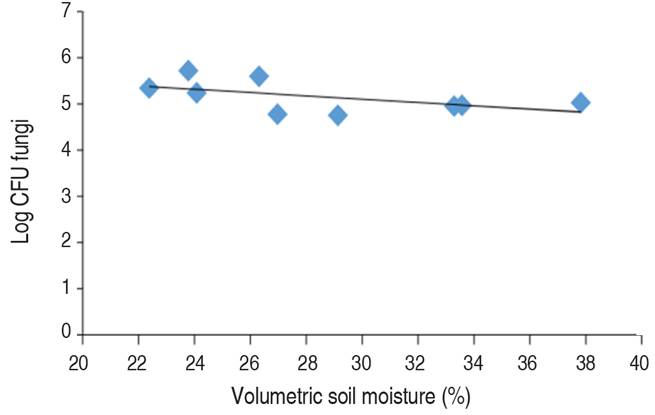
Figure 3 Correlation graph between volumetric soil moisture and Log of CFU Fungi (Pearson correlation=-0.54).
In Figure 4, it was evident that increases in soil moisture content are related to marked decreases of C-MB, which can be explained considering that the excess of water in soil displaces the air and, with this, the availability of O2 for the microorganisms, reducing their survival, (Siebielec et al. 2020; Visconti and Valenzuela 2023).
In addition, the correlation analysis between the soil's physical variables and the soil's microbiological activity confirmed that the decrease in quantity and size of pores due to soil compaction affects soil microorganisms, which implies a danger to soil biodiversity (FAO 2015).
On the other hand, it is considered that the response of soil microorganisms to the increase of macropores is because of the improvement of soil aeration and drainage (Lipiec et al. 2012; Santamaría et al. 2018). Furthermore, the destruction of soil macropores leads to a higher proportion of micropores, which hinders the movement of water, creating conditions of water excess and oxygen deficit that affects the activity of microorganisms (Figure 2) (Ishak et al. 2016).
The correlation between soil moisture and fungi count (Figure 3), showed that increasing soil moisture decreases the number of fungal colony-forming units. Calvo et al. (2008) expressed that excess moisture in soil saturates the pores and creates conditions of lack of O2. Furthermore, Santamaría et al. (2018) explained that water is necessary for all living organisms. Still, fungi survive better in semi-arid regions and suffer more when soil moisture is high enough to provoke oxygen shortage in the soil. In this same sense, Vanegas et al. (2013) expressed that the excess of water inhibits the survival of the microorganisms; as found in this study (Figure 4), since under flooding, the air in the soil pores is replaced by water and oxygen is rapidly consumed by root respiration and microbial activity (Morugán-Coronado et al. 2019).
The analysis of principal components for the variables measured in this study has allowed us to interpret that the thirteen variables are grouped into three main parts capable of explaining 77.07% of the variability in the data (Table 5).
Interpreting the weight with which the variables are grouped in each component allows us to analyze the influence of the measured physical characteristics on the presence of soil microorganisms.
The principal component analysis interprets that the variables C-MB, SOC, Fungi, and bacteria count are grouped with greater weight in component one. This allows it to point out the critical influence that the content of organic matter has on the activity of soil microorganisms. Therefore, the SOC directly influences biological activity and largely determines the variability of the parameters related to soil microorganisms' activity, as Zhao et al. (2019) mentioned.
In the case of component two, the variables porosity, macropores, and fungi count are grouped with greater weight. This indicates the critical influence of the physical soil conditions involved with the soil aeration and drainage relationships and the presence and survival of fungi in the soil, confirming that these microbes grow better in dryer conditions.
Finally, in component three, it was found that the variables with the greatest weight were: soil temperature, soil moisture, pH, and electrical conductivity, which indicates that climatic conditions had a direct influence on the environment that the soil offers to microorganisms, and this affects their survival, as mentioned in other studies conducted by Liu et al. (2018).
CONCLUSIONS
According to the results of this study, soils with less anthropic intervention and in which a permanent plant cover exists, such as the use of forest with cocoa and pasture, presented the highest soil organic carbon content, which favored the presence of microorganisms in the soil, evidenced by a higher carbon content of microbial biomass. This also coincides with better soil physical conditions, expressed by bulk density and macroporosity.
The results also indicated that, among the fungi and bacteria, the first can survive better with less soil water content, so physical soil conditions have a differentiated effect on each microorganism. Especially the total amount of pores and their size distribution, due to their role in aeration and water retention. As a result, this research emerges as promising material for evaluation of the microorganisms under different conditions environmental in agriculture use systems.