The cacao tree belongs to the family Malvaceae, genus Theobroma L., and is native to the upper Amazon basin, specifically Colombia, Brazil, Peru, and Ecuador. Cocoa beans are used to produce chocolate, cocoa beverages, and as an additive in confectionery, and ice cream, among others. Likewise, they have cosmetic and pharmaceutical applications due to their anti-inflammatory, antidepressant, and antioxidant properties (Vásquez et al. 2019).
Around five million farmers in the world grow cocoa, and it is the main source of income for more than 40-50 million people. According to the International Cocoa Organization (ICCO), world cocoa production in the 2021-2022 season was 4.8 million tons; Ivory Coast, Ghana, Brazil, Ecuador, and Indonesia are some of the main producers. In 2019, ICCO ratified Colombia as an exporter of fine and aromatic cocoa. This maintains the competitiveness of the national beans and the country's qualification as a producer and exporter of fine cocoa by 95%. According to the National Cocoa Growers Federation, in the years 2021-2022, the country recorded a production of 62,741 tons of cocoa beans and kept the upward trend of the last 10 years. The main producing departments were Santander (36.8%), Arauca (16.9%), Antioquia (8.3%), Tolima (5.8%), Huila (5.7%), and Nariño (5.4%). The Agricultural Risk Management Unit published that approximately 65,000 families are cocoa producers.
The generation of large amounts of organic waste -also called residual biomass- is the main drawback that agribusiness, as a whole, faces, which is why strategies are proposed to add value to the by-products according to their characteristics and their possible applications. The world cocoa production indicates that approximately 50 million tons of residual biomass are produced, in most cases they are left on plantations, thus causing environmental and phytosanitary problems (Sarmiento-Vásquez et al. 2021).
The processing and transformation of cocoa consists of four fundamental stages. In the shelling stage, the pods are manually opened to extract the beans, which are covered by mucilage. Subsequently, in the fermentation stage, the mucilage is degraded by microbial action, and the generation of precursors of the aroma and flavor characteristic of chocolate is promoted. In the drying stage, the moisture of the beans is reduced by up to 7% approximately. Finally, in the roasting stage, some chemical changes continue as a result of what happened in the fermentation and drying. Aromatic compounds are generated by the action of temperature due to amino acid degradation reactions and interaction with reducing sugars in the bean. These processes generate by-products such as shell, husk, and exudates from the fermentation process, which represent approximately 74-86% of the total weight of the fruit (Figure 1) (Aprotosoaie et al. 2015; Vásquez et al. 2019).
The husk is the outermost part of the cocoa and is obtained when the cocoa beans are separated from the pod, this constitutes between 52-70% of the total weight of the fruit. Ten tons of husk are generated for each ton of dried cocoa beans (Campos-Vega et al. 2018). The shell is the part that covers the cocoa bean, that is separated from it before or after the roasting process (Rojo-Poveda et al. 2020) and represents about 12-20% of the weight of the dried bean. Considering world production, approximately 700 thousand tons of shell would be produced per year (Okiyama et al. 2017). The mucilage (white pulp) envelops the beans, it constitutes between 30 and 45% of the weight of the pod and is separated from the bean in the fermentation stage, generating about 100-150 L of exudates per ton of cocoa beans (Vásquez et al. 2019).
Currently, different sectors have focused on these by-products to use them and strengthen the development of high-value-added products; for the extraction and application of structural components (cellulose, hemicellulose, lignin, and remaining minerals), biomolecules (theobromine, antioxidants, phenolic compounds, and complex carbohydrates), ant to explore their heat capacity for bioenergy generation (Vandenberghe et al. 2022). These residues can also generate beneficial effects on human health because they contain bioactive substances such as polyphenols and methylxanthines. Their composition could be included in different matrices to develop new products in the market (Grillo et al. 2019). In this context, this research seeks to analyze the potential of cocoa by-products -shell and husk- by bromatological analysis, aromatic compounds, fatty acid profile, polyphenol content, methylxanthines, and antioxidant capacity to identify their application in biorefinery from a bioactive substance's perspective.
MATERIALS AND METHODS
Raw material
Agro-industrial residues from Theobroma cacao L. cultivar CCN 51 were used, specifically from the pulping stage (husk), and transformation stage (shell) -i.e., roasting- harvested in 2020 by local regional farmers in Cundinamarca, Colombia. The by-products were subjected to a drying process in a MEMMERT UFE600 forced convection oven at 40 °C for 16 h up to a humidity of about 7%; subsequently, the particle size was reduced in a V-MOLM hammer mill and sieved using a 60 mesh (250 microns) (Nguyen and Nguyen 2017; Pico Hernández et al. 2019).
Bromatological and lignocellulose composition
The moisture and ash content were determined according to AOAC 931.04 and 972.15 methods, respectively. Calcium, potassium, magnesium, manganese, and zinc contents were determined by atomic absorption spectrometry. The phosphorus content was determined by UV-VIS spectrophotometry. The crude protein (Kjeldahl method), fat, and crude fiber content were determined according to the MRE-001 standard. The content of Klason lignin (insoluble acid) was determined based on the TAPPI T 222 om-02 standard, and the content of cellulose and hemicellulose was evaluated according to the ASTM D1695-07 standard.
Aromatic compounds
They were evaluated following the methodology described by Rojas M et al. (2020) with some modifications, using gas chromatography-mass spectrometry equipment (Shimadzu GC/MS-QP 2010 Ultra). Volatiles were extracted using the solid phase microextraction technique (HS-SPME) with a StableFlex Divinylbenzene/Carboxen/Polydimethylsiloxane fiber (DVB/CAR/PDMS). The fiber was preactivated at 250 °C for 30 min. The samples were subjected to initial heating of 10 min at 60 °C, the fiber was exposed to the headspace for 30 min at about 66 °C, and the desorption time was 5 min at 250 °C. The analysis was performed using a Restek Rtx-5MS column (30 m x 0.25 mm ID x 0.1 µm) with helium as carrier gas and a constant flow of 1 mL min-1. The temperature started at 50 °C for 3 min following a gradient of 230 to 10 °C per minute and was maintained at 230 °C for 40 min in the splitless injection mode. The mass spectra of the sample compounds were compared to the National Institute of Standards and Technology database (NIST Library, Gaithersburg, MD, US).
Lipid content analysis
Total lipid extraction was performed. For that, 13 mL of dichloromethane/methanol (2:1% v/v) were added to the sample, the mixture was stirred (Vortex LAB SCIENCE Mixer V8) and centrifuged (HERMLE Z366K) at 10,000 rpm for 10 min and subsequently filtered. 2 mL of 0.73% sodium chloride was added to the obtained extracts, stirred, and centrifuged at 3,500 rpm for 10 min; two phases were formed, and the lower phase was taken to the forced convection oven (MEMMERT UFE 600) at 45 °C until the dried lipid fraction was obtained.
The fatty acid methyl esters were obtained following the methodology described by Villarreal-Peña et al. (2012). For that, 5 mL of sulfuric acid/methanol (1:2% v/v) were added to the glass tubes with the dried total lipids and stirred for 60 min at 60 °C to form the methylation reaction. Subsequently, 2 mL of hexane was added. Fatty acid methyl esters were evaluated using a mass-coupled gas chromatograph (Shimadzu GCMS-QP 2010 Ultra) and a DB-WAX column (30 m x 0.250 mm x 0.25 µm, Agilent J&W), the carrier gas was helium. An injection volume of 1 µL, dichloromethane solvent, injector temperature 225 °C, column flow 1.21 mL min-1, and Split 3:1 were set. The temperature ramp was 100 °C, 4 min, climbed to 3 °C min-1 to 193 °C and finally increased to 1.5 °C min-1 to 240 °C, 10 min with a total run time of 76 min. The equilibration time of the system was 3 min, and the data scanning started at 4 min. Fatty acids were identified by comparing retention times of characteristic peaks generated by 37 methyl ester standards (FAME Mix Supelco 37). Quantification was performed by calibration curves for each fatty acid and the data were expressed in terms of percentage of the total area.
Sonication-assisted extraction
An extraction by sonication and maceration was performed to evaluate the content of total polyphenols, and antioxidant capacity (DPPH and ABTS), and to identify the bioactive compounds by HPLC of the cocoa husk and shell. In a test tube, 8 mL of acetone-water (70:30, v/v) were added to 400 mg of sample, then the mixture was subjected to a Branson 3800 CPXH ultrasonic bath for 15 min at 40 kHz ultrasonic radiation. After sonication, maceration was performed, and the samples were subjected to constant stirring for 4 h at room temperature. The extracts were centrifuged (HERMLE Z366K) at 10,000 rpm for 15 min, the supernatants were removed and stored at -30 °C until use.
Total polyphenol content
The total polyphenol content was determined using the Folin-Ciocalteau reagent. 50 µL of the extract, 425 µL of distilled water, and 125 µL of the reagent were added to each test tube and stirred. Subsequently, 400 µL of 7.1% sodium carbonate (Na2CO3) solution was added. The tubes were left in the absence of light for 1 h. Absorbance was recorded at 760 nm on a THERMO SCIENTIFIC G10S UV-VIS spectrophotometer. The calibration curve was performed using gallic acid solutions at concentrations between 0-500 mg L-1 (R2=0.997). The results were calculated from equation 1 and expressed in mg gallic acid equivalent per gram of dry sample (mg GAE g-1).
Where:
C = Gallic acid concentration (mg L-1) Vtotal = Total volume used in extraction (µL) DF = Dilution factor DW = Dry weight of the sample used in the extraction (g)
Antioxidant capacity
The ability of the samples to trap the DPPH (2.2-diphenyl-1-picrylhydrazyl) radical was evaluated by decreasing the absorbance to 517 nm. 10 μL of the extract and 990 μL of a DPPH solution-which was prepared in methanol (0.05 mM)- were added to a test tube. The tubes were shaken and stored in the dark for 30 min. Absorbance was measured at 517 nm. The calibration curve was constructed with Trolox solutions at concentrations between 200 and 1800 μmol L-1 diluted in methanol (R2=0.994).
The cationic radical ABTS•+ was generated by the oxidation reaction of ABTS (2.2'-azino-bis-3-ethylbenzothiazoline-6-sulfonic acid) with potassium persulfate for 16 h in the dark. In a test tube, 990 μL of ABTS reagent was reacted with 10 μL of the extract. The tubes were shaken and allowed to react in the dark for 7 min. Absorbance was measured at 734 nm. The calibration curve was constructed using Trolox solutions at concentrations between 200 and 2200 μmol L-1 diluted in ethanol (R2=0.993).
The results for DPPH and ABTS were expressed as percent inhibition and μmol Trolox equivalent per gram of dry sample (µmol Trolox g-1), according to the following equations (2) and (3):
Where:
A1 control absorbance and A2 sample absorbance.
Where:
C = Trolox equivalent concentration (µmol L-1) Vtotal = Total volume used in extraction (µL) DF = Dilution factor DW = Dry weight of the sample used in the extraction (g)
Identification of bioactive compounds by HPLC
It was conducted using cocoa husk and shell extracts following the methodology described by Carrillo et al. (2014) with some modifications. High-performance liquid chromatography (HPLC) equipment (Shimadzu Prominence LC-20AT) with a diode detector (PDA, SPD 20AT) was used. The column employed was the C18 Zorbax Eclipse Plus Agilent (150 mm x 4.6 mm, 5 µm) and a Zorbax NH2 4-Pack pre-column (12.5 mm x 4.6 mm, 5 µm).
The analysis was performed in gradient mode using 1% acidified water with acetic acid (A) and 1% acidified acetonitrile (B) as mobile phases. The program started with 0% B, 5.3 min; 0-90% B, 8.7 min; 90-70% B, 3 min; 70-30% B, 2 min; 30-0% B, 2 min; and 0% B, 4 min at a flow of 1.3 mL min-1 and a total run time of 25 min. The injection volume was 10 µL, and the oven temperature was 40 °C. The reading was performed at 280 nm. To identify catechin and epicatechin, a calibration curve between 5 and 200 ppm of the standards (catechin R2=0.997 and epicatechin R2=0.996) was conducted.
Similarly, a calibration curve was conducted between 5 and 75 ppm of the theobromine (R2=0.997) and caffeine (R2=0.998) standards. Mobile phases were 1% acidified water with acetic acid (A) and 1% acidified acetonitrile (B) following an initial gradient of 10% B, 0-0.02 min; 20% B, 0.02-5.3 min; 25% B, 5.3-8 min; 27% B, 8-10 min; 30% B, 10-12 min; 40% B, 12-15 min; 60% B, 15-17 min; 30% B, 17-19 min; and 0% B, 19-25 min. The injection volume was 15 µL and the temperature was 30 °C. The reading was performed at 280 nm.
Statistical Analysis
The statistical analysis was carried out by the STATGRAPHICS Centurion XVI software version 16.1.18, applying the mean comparison -Tukey test with a significance level of P<0.05- to the analysis results of lignocellulosic content, polyphenols, antioxidant capacity, and methylxanthines. All analyses were performed in triplicate.
RESULTS AND DISCUSSION
Bromatological composition of cocoa shell and husk
Table 1 presents the bromatological composition of the cocoa husk and shell. The values of fat, crude protein, zinc, and manganese contents were higher in the shell than in the husk, while the content of crude fiber was higher in the husk. These results are consistent with those presented by Yapo et al. (2013), these authors found a higher protein value in husks from hybrid cocoa in Abidjan, Ivory Coast, as well as a higher fat content. However, Villamizar-Jaimes and López-Giraldo (2017) found similar fat content values in husks from clone CCN-51 collected in San Vicente de Chucurí-Santander, Colombia.
The value of crude protein in cocoa shells (Table 1) is consistent with that found by Nsor-Atindana et al. (2012) in shells obtained from a manufacturing company in Wuxi, China, and by Fakhlaei et al. (2020) in shells from Jengka Pahang, Malaysia. Agus et al. (2018) reported a higher protein and ash content in Jengka Termerloh, Malaysia. The cocoa shell can be used for animal feed thanks to its protein content along with other constituents such as fiber and minerals.
Grassia et al. (2019) and Yapo et al. (2013) studied the proximal composition of cocoa beans and reported a higher fat content (33-56.54 g 100 g-1) relative to cocoa husk and shell, while the protein content (9.9-12.96 g 100 g-1) was lower compared to shell. The ash content in the husk and shell was higher than in the bean (0.9-3.71 g 100 g-1). Furthermore, the fiber contents in the husk and shell were higher than the fiber values reported by Vázquez-Ovando et al. (2016) in cocoa beans from Mexico (3.13 g 100 g-1) and Venezuela (4.23 g 100 g-1). The fiber content gives nutritional value to the husk; thus, it can be used to obtain value-added products (Villamizar-Jaimes and López-Giraldo 2017). The fiber content (Table 1) was similar to that reported by Vriesmann et al. (2011) in husks from Itabuna, Brazil, and by Pico Hernández et al. (2019) in husks from clone CCN-51 collected in Colombia.
Regarding the shell, the fiber content agrees with that reported by Fakhlaei et al. (2020), while Agus et al. (2018) report a lower fiber content; however, they found a similar fat content. Due to its high fiber and low-fat content, the shell has been of great interest in the food industry. Its potential to be used as a functional ingredient in confectionery, bakery, and low-calorie products to achieve a high-fiber diet by decreasing and controlling cholesterol and glucose levels in the blood has been evaluated (Okiyama et al. 2017).
The differences between some parameters in the bromatological composition can be attributed to the origin and variety of the samples, the climatic conditions, the agronomic management of the crop, the fermentation and roasting process, and the processing conditions to which they were subjected (Zapata et al. 2015; Grillo et al. 2019).
Minerals such as phosphorus, calcium, magnesium, and potassium are required for cells to maintain their vital functions; their presence in the cocoa husk makes it suitable to be used as a source of these minerals (Campos-Vega et al. 2018). The contents are similar to those reported by Vriesmann et al. (2011) and Yapo et al. (2013). Moreover, the shell has been identified as a good source of minerals including potassium, magnesium, calcium, phosphorus, copper, and zinc (Cinar et al. 2021). The calcium, phosphorus, and potassium content found in the shell were similar to those reported by Yapo et al. (2013) in samples from a hybrid cocoa plantation derived from Forastero in Abidjan, Ivory Coast, while the magnesium content was lower.
Cinquanta et al. (2016) evaluated the content of zinc (48.8 mg kg-1) and manganese (30.1 mg kg-1) in cocoa beans from Ghana and obtained values lower than those found in the shell. Microelements such as zinc and manganese present in cocoa husk, shell, and beans depend on fertilization in plantations, which is why variations can be attributed to the type of fertilizer and the richness of these microelements in the soil (Lares et al. 2014).
Figure 2 shows the lignocellulosic content of the cocoa husk and shell. The cellulose content showed significant differences -being higher in the husk- while the hemicellulose content was higher in the shell. Similarly, Vásquez et al. (2019) reported a higher content of cellulose in the husk compared to the shell, while the lignin content was higher in the shell.
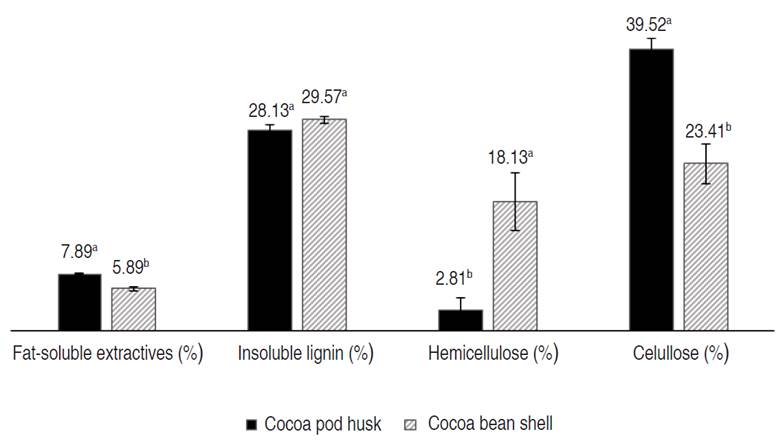
Figure 2 Lignocellulosic content of cocoa husk and shell. Different letters a and b indicate significant differences (P<0.05).
Budaraga and Putra (2020) presented similar values of cellulose, hemicellulose, and lignin in husks from Indonesia. Concerning shell, Wijaya and Wiharto (2020) reported higher lignin and cellulose values and a lower value of hemicellulose content in samples from Enrekang, Indonesia. Gómez Hoyos et al. (2020) reported a lower lignin content in shells from Colombia.
These residues containing lignocellulose are a source of biomass and therefore can be used to produce biofuels, energy, and products of interest for various economic sectors, by applying pretreatments to degrade the structure and obtain substrates rich in fermentable compounds in addition to cellulose to develop biodegradable materials. According to Sakagami et al. (2008), phenolic compounds retained in the lignin fraction of the husk exhibit high antimicrobial and antiviral activity, and some of the components have been used against the HIV-1 virus obtaining promising results.
Fatty acid profile
In the cocoa husk, palmitic, linoleic, and oleic fatty acids were the most prominent; palmitic acid was the highest with 36.92%, as detailed in Table 2. Conversely, in cocoa shell, oleic, elaidic, and stearic acids were predominant, elaidic acid stood out with the highest proportion, 28.68%. The husk showed a higher percentage of saturated and polyunsaturated fatty acids, while the shell had a higher content of monounsaturated fatty acids.
Carta et al. (2020) reported the lipid profile of cocoa husk collected from an Italian food industry. The palmitic acid content was lower, while the percentages of oleic, linoleic, and linolenic acids were higher. The percentage of saturated fatty acids (53.59%) was higher and that of unsaturated fatty acids was lower (46.41%).
Lessa et al. (2018) evaluated the fatty acid profile in cocoa shells from Bahia, Brazil, and reported a higher content of palmitic acid and stearic acid, obtaining a content of oleic acid similar to the one reported in this research. The percentage of saturated fatty acids (64%) was higher, and that of monounsaturated (28.7%) and polyunsaturated (6.03%) fatty acids was lower. Similarly, Botella-Martínez et al. (2021) mentioned that the main fatty acids obtained from the shell of the forastero variety cultivated in Ghana were palmitic, stearic, and oleic acids.
Grassia et al. (2019) identified the fatty acids of cocoa beans in the criollo variety from Ecuador and the hybrid variety from Ghana, reporting the content of three major acids in cocoa beans: palmitic acid, with values lower than that found in cocoa husk; stearic acid and oleic acid, with values higher than that found in the cocoa shell; and lower contents in the main fatty acids i.e., linolenic acid and linoleic acid. The percentage of saturated fatty acids (60.60-63.92%) was higher. The percentage of monounsaturated (32.56-36.62%) was higher than that of the husk and lower than that of the shell. The percentage of polyunsaturated acids (2.08-3.59%) was lower.
According to Rachmawaty et al. (2018), stearic and oleic acids have antimicrobial activity; the latter is also attributed to an antioxidant and anticancer potential. That is why cocoa by-products that contain these acids can be used in the food industry as fat substitutes for certain foods or to obtain products with antioxidant and antimicrobial properties. For example, stearic acid is suitable for producing emulsifiers, emollients, lubricants, cosmetics, and personal care products (Tulashie et al. 2022). In another study, resveratrol and fatty acids, such as linoleic, present in cocoa husk possess skin-lightening properties and show UVB sunscreen potential (Campos-Vega et al. 2018).
Identification of aromatic compounds
Pyrazines, aldehydes, ketones, alcohols, esters, furans, acids, pyrroles, and terpenes are some of the volatile organic compounds that characterize the aroma of cocoa (Barbosa-Pereira et al. 2019). The amount and type of volatile compounds in cocoa beans are important to define their quality, as well as their commercial value based on the unique and complex flavors and aromas of chocolate (Utrilla-Vázquez et al. 2020). Table 3 presents the aromatic compounds identified in the cocoa husk and shell with their respective description of the aroma reported in the literature. Identifying them can contribute to the search for potential alternatives to obtain new products and add value to these residues.
The taste and aroma of different cocoas are the result of several factors, among them, the most important is the genetic origin, followed by the environment where the trees grow. It is influenced by the edaphoclimatic conditions and vegetation, in addition to factors such as primary management (soil preparation, agronomic management) and post-harvest (pulping, fermentation, drying, roasting).
A study conducted by Barros (2017) identified 50 volatile compounds in cocoa husks from Pará, Brazil, out of which 16 were alcohols, 11 hydrocarbons, 8 aldehydes, 7 ketones, 5 esters, 2 amines, and isovaleric acid. Values greater than those identified in the husk.
Barbosa-Pereira et al. (2019) conducted a study to classify and characterize the volatile compounds of cocoa shells obtained from beans from different cultivars and collected in various geographical origins. They identified a total of 101 compounds comprising 15 aldehydes, 9 ketones, 4 sulfur compounds, 8 esters, 2 hydrocarbons, 3 furans, 21 pyrazines, 7 alcohols, 4 pyrroles, 10 terpenes, 10 acids, 3 lactones, and 5 other compounds. Values greater than those obtained in the shell.
Compounds such as acetic acid, 2-phenylethyl ester; benzeneacetaldehyde; 2-heptanol; 2-phenylethanol; and trimethyl-pyrazine enhance the shell as a food ingredient, so it is important to describe and study its volatile composition to define the quality and flavor of the product. For instance, shell has been used to produce chocolate flavor with a real chocolate aroma using enzymatic technology to make products such as cookies and bread. For its part, the husk has demonstrated potential as an inert support for the bioconversion of secondary metabolites by fungal strain, that is, obtaining volatile fragrance components in a solid-state fermentation system (Campos-Vega et al. 2018).
Polyphenols, methylxanthines, and antioxidant capacity
The total polyphenol content and antioxidant capacity of the husk and shell are presented in Table 4. The content of total polyphenols and the antioxidant capacity, DPPH, and ABTS, in the husk showed significant differences with respect to the shell; the values of the husk are higher. The total antioxidant capacity of the soluble fractions of the husk exhibits greater synergistic interactions between various compounds with antioxidant properties, including low molecular weight phenolic compounds, medium molecular weight proanthocyanindines, and oligosaccharides and/or polysaccharides derived from the cell wall matrix peptide (Yapo et al. 2013).
The total phenol content can vary due to environmental factors such as sample origin, variety, maturity, climate and processing, roasting, tempering, alkalization, and storage (Hernández-Hernández et al. 2018). It depends on extraction methods, solvent polarity, and sample/solvent ratio (Campos-Vega et al. 2018), which in turn affects the antioxidant properties of a given extract (Schinella et al. 2010).
Sotelo et al. (2015) performed an extraction process in husks of the clone TSH 565 from Tierralta, Córdoba (Colombia) by stirring with ethanol-water acidified with 1% HCl and obtained a lower content of polyphenols of 19.26±0.46 mg GAE g-1. Quiroz-Reyes et al. (2013) obtained a similar content of polyphenols (25.34±1.82 mg GAE g-1) using a first extraction with water-methanol and a second phase with acetone-water in husks from Mexico.
With respect to the cocoa shell, Hernández-Hernández et al. (2019) obtained a lower polyphenol content (11 mg GAE g-1) in samples of different genotypes from Mexico using a methanol-water extraction with HCl acidified stirring. Lessa et al. (2018) reached a similar polyphenol content (21.2 mg GAE g-1) in shells from Bahia, Brazil, using an alcoholic solution of hydroethanol as solvent.
In another study, Hernández-Hernández et al. (2018) applied the extraction by maceration technique to cocoa beans from Mexico, using ethanol-water acidified with HCl as a solvent and a second extraction with acetone-water. They reported a polyphenol content of 49.46±2.50 mg GAE g-1, higher than that found in cocoa husk and shell.
The DPPH and ABTS methods were used to calculate the antioxidant capacity in the extracts, which varies according to the composition of the raw material and the system used for the extraction. Both methods are influenced by many factors; therefore, it is necessary to use several methods to include various mechanisms that contribute to the antioxidant action.
Rachmawaty et al. (2019) performed maceration extraction on cocoa husks from West Sulawesi, Indonesia. They used acetone-water and 70% ethanol as solvents and evaluated DPPH antioxidant capacity (percent inhibition). They obtained values of 61.58 and 69.19% for ethanol and acetone-water, respectively. The result obtained using acetone-water as solvent was very similar (Table 4). The acetone extract generates a higher DPPH buffering activity than that of ethanol because the content of phenolic compounds is easily soluble in acetone compared to ethanol. Sotelo et al. (2015) applied the ultrasonic extraction technique with acidified ethanol-water (1% HCl) to husks of the clone TSH 565 to analyze their ABTS antioxidant capacity and obtained a value greater than 229.61 µmol Trolox g-1.
Jokić et al. (2018) applied the subcritical water extraction technique (Temperature 170 °C) to cocoa shells from a chocolate factory in Osijek, Croatia, to obtain bioactive compounds by evaluating their antioxidant capacity by DPPH inhibition percentage. They obtained a similar value of 56.11%, while Lessa et al. (2018) obtained a higher DPPH inhibition percentage, 79.2%, in shells from Bahia, Brazil.
Zapata et al. (2015) used methanol maceration extraction on cocoa beans of the clone CCN 51 from Cauca, Colombia, to determine the trapping activity of the ABTS radical, which yielded a value of 384.36 µmol Trolox g-1. García-Alamilla et al. (2017) found a DPPH inhibition percentage of 84.83% in cocoa beans from Tabasco, Mexico, using an aqueous extraction by shaking for 2 h. According to the two previous investigations, the cocoa beans had a higher antioxidant capacity than the husk and shell.
Table 4 shows that the higher the content of total polyphenols, the higher the antioxidant capacity. It indicates a possible correlation between these variables. The extracts obtained from the cocoa husk and shell have antioxidant and antimicrobial activity attributed to polyphenols and methylxanthines. Therefore, these by-products are promising and of great interest in various fields of application such as human health, the food and cosmetics industries, and bioremediation.
The concentrations of polyphenols (catechin and epicatechin) and methylxanthines (theobromine and caffeine) obtained by HPLC are presented in Figure 3. Rojo-Poveda et al. (2020) reported an interaction between flavonoids and cocoa methylxanthines, which helps increase the plasma concentration of epicatechin metabolites and improve the vascular effects attributed to flavonoids.
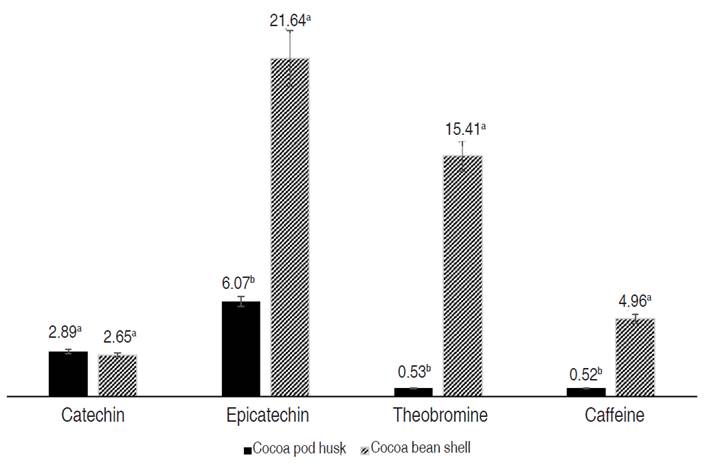
Figure 3 Phenols and methylxanthines in cocoa husk and shell extracts by HPLC. Values were expressed in mg g-1 of dry extract. Different letters a and b indicate significant differences (P<0.05).
Cocoa husk presented a significant difference in the concentrations of epicatechin, theobromine, and caffeine compared to shell; however, the large amount of husks discarded by the cocoa industry makes it a cheap, renewable, and sustainable source for the extraction of these compounds.
The extraction process, solvent, and extraction time greatly influence the concentration of phenols and methylxanthines. The genotype, origin, degree of maturity, and processing of cocoa also influence the variation of concentrations. According to Carrillo et al. (2014) when acids are added to the HPLC mobile phase, the separation of the polyphenols improves, since it allows the reduction in ionization of both hydroxyl and carboxyl phenolic groups.
Sotelo et al. (2015) evaluated the concentration of epicatechin, caffeine, and theobromine in the husk extracts obtained by stirring and ultrasound with and without HCl. Stirring and ultrasound extraction yielded the highest values, both with HCl, without significant differences between them. The values of epicatechin (0.342-0.350 mg g-1), caffeine (0.039-0.043 mg g-1), and theobromine (0.032-0.038 mg g-1) were lower.
Rahayu et al. (2019) applied ethanol microwave-assisted extraction to the cocoa husk from Indonesia and evaluated the concentration of catechin in the extract. They obtained a concentration range between 47.80-51.03 mg L-1 of catechin, greater than that found in the husk extract obtained by sonication-assisted acetone (28.88 mg L-1).
Quiroz-Reyes et al. (2013) conducted a study in Mexico and determined the concentration of catechin and epicatechin in the bean and cocoa shell by extraction with maceration and ultrasound, using water-methanol and acetone-water as solvents. The values of catechin (maceration 0.28 mg g-1 and ultrasound 0.32 mg g-1) and epicatechin (maceration 2.64 mg g-1 and ultrasound 2.77 mg g-1) in the shell were lower, while the concentrations of catechin (maceration 4.62 mg g-1 and ultrasound 4.26 mg g-1) and epicatechin (maceration 132.88 mg g-1 and ultrasound 144 mg g-1) in the cocoa beans were higher.
Hernández-Hernández et al. (2018) carried out a study on cocoa beans and cocoa shells in Mexico evaluating the concentrations of theobromine, catechin, and epicatechin extracts obtained with acidified water and stirring methanol. The concentrations of theobromine (12.00 mg g-1) and epicatechin (17.70 mg g-1) in the shell were similar, while those of the fermented cocoa bean (theobromine 9.79 mg g-1 and epicatechin 6.13 mg g-1) were lower. The concentration of catechin was lower in both the bean (1.48 mg g-1) and the shell (1.2 mg g-1).
CONCLUSIONS
Cocoa husk and shell had a similar lignin content. Protein and fat content were higher in the shell, while the husk had a higher cellulose content. In the shell, pyrazines, esters, and oleic, elaidic, and stearic fatty acids predominated, in contrast to the husk, where aldehydes, alcohols, and palmitic, oleic, and linoleic fatty acids prevailed. Cocoa residues are known for the presence of polyphenols, which are correlated with antioxidant capacity. The husk presented a higher content of total polyphenols (26.64 mg GAE g-1), antioxidant capacity DPPH (157.85 µmol Trolox g-1) and ABTS (214.67 µmol Trolox g-1) than the shell (19.18 mg GAE g-1, 116.27 µmol Trolox g-1, and 113.91 µmol Trolox g-1, respectively). The shell had higher values of epicatechin (21.64 mg g-1), theobromine (15.41 mg g-1), and caffeine (4.96 mg g-1) than the husk (6.07, 0.53 and 0.52 mg g-1, respectively).
The bromatological and chemical characterization of cocoa shells, and husk is essential to understand their potential as high-value-added by-products in various industries, such as food, pharmaceuticals, and cosmetics. Thanks to its properties, it has potential application as an ingredient in bakery and pastry products, in the production of biofuels, or in obtaining extracts with antioxidant and antimicrobial properties. The use of these by-products can benefit cocoa farmers, protect crops from contamination, and promote the sustainable development of the entire cocoa industry. For future research, it is proposed to conduct an economic, social, and environmental study of the use of cocoa by-products, investigate the development of new products and innovative applications, evaluate alternative methods to extract bioactive compounds -including the evaluation of the antioxidant capacity by other methods such as FRAP, ORAC, or superoxide anion- and explore the properties of mucilage and its potential for various applications.