INTRODUCTION
Bone is a hard tissue as a result of a mineralization process. The inorganic phosphate necessary for bone mineralization is obtained from different substrates, such as pyridoxal phosphate, inorganic pyrophosphate or phosphoethanolamine (Saraff et al, 2016). These substrates are hydrolyzed by alkaline phosphatases (ALP) to provide inorganic phosphate for bone tissue development. There is a direct relationship between low ALP activity and bone disorders, which further demonstrates the importance of ALP in the mineralization process (Uday et al, 2019). The production of ALP in different cell lines have been used since 1994 to prove the biological activity of Bone Morphogenetic Protein (BMP) ligands, which are members of the transforming growth factor superfamily (Allendorph et al, 2006; Krishnakumar et al, 2017; Rahman et al, 2015). Of all BMP ligands, BMP-2 and BMP-7 have been shown as the main factors to induce complete bone formation (Kim et al, 2019; Yilgor et al, 2009). Additionally, BMP-2 is a critical factor in the fracture healing process, as demonstrated by the inability of BMP-2 deficient mice to initiate this process (Bahney et al, 2019). The above evidence established BMP-2 as a pivotal mediator necessary for bone formation.
At the tissue level, BMP-2 becomes biologically active after the dimerization of two monomers of 114 residues each one, giving the protein a relative molecular mass of 26 kDa. The dimerization of two monomers is maintained by three intrachain disulfide bridges (Scheufler et al, 1999). The signaling pathways are initiated when BMP-2 binds its receptor, which has a serine/threonine kinase activity. The transphosphorylation occurs after the BMP-2 binds to receptor type II and this receptor proceeds to phosphorylate to receptor type I. The downstream effects after phosphorylation of receptor type II and type I are the phosphorylation of Smad transcription factors (Miyazono et al, 2010). Smads are translocated into the nucleus and bind to regulatory regions of BMP responsive genes, among them RUNX2/CBF (Bahney et al, 2019; Mevel et al, 2019).
As mentioned above, BMP-2 plays an important role in bone formation. Its potent osteogenic abilities have been used to improve fracture repair in the USA (Ruehle et al, 2019). Commercially, a BMP-2 based collagen sponge (Infuse) (Durham et al, 2018) has been developed by Medtronic; however, this device causes swelling in the neck and life-threatening complications. Thus, it is necessary to develop safer and cost-effective devices.
With the development of recombinant technology, mass production of BMP-2 becomes easier. Although multiple commercial houses sell this product (Thermofisher Scientific, R&D systems and Sigma-Aldrich), they sell small amounts at a high cost (317 USD per 10 µg protein). This is insufficient for clinical usage (up to 1.5 mg/mL). Additionally, recombinant BMP-2, like many proteins expressed in bacteria, forms aggregates in structures known as inclusion bodies (IB) (Long et al, 2006). In IB, proteins are unfolded, rendering them inactive. Therefore, an in vitro refolding step is needed to solubilize and activate the accumulated proteins (Krauss et al, 2013). The refolding process is the main bottleneck in protein production. This process is time consuming, increasing the cost of production and limiting its scalability in an industrial setting (Vallejo et al, 2002).
This work aims to establish a protocol to obtain the recombinant human BMP-2 (rhBMP-2) in a cost-efficient way. In this paper, we describe the production of rhBMP-2 in Escherichia coli (E. coli) engineered in a synthetic gene. Our results showed a feasible BMP-2 production system that can synthesize rhBMP-2 in a cost-efficient manner, which has potential applications in bone tissue engineering.
MATERIALS AND METHODS
Reagents
Human recombinant BMP-2 protein was purchased from Gibco. The human cell lines C2C12 and Saos-2 were obtained from ATCC. The basic chemicals were obtained from Sigma-Aldrich, Fermentas and Amresco. The enzymes were purchased from New England Biolab and Fermentas. The protein was dialyzed in Spectra/por 3 regenerated cellulose membranes from Millipore. The Spectra/por 3 (FisherScientific) has a Molecular Weight Cutoff (MWCO) of 3500 kDa, a diameter of 11.5 mm, and a capacity of volume/length of 1 mL/cm. Proteins were concentrated by ultra-filtration (Amicon system) with YM3 membranes (3000 MWCO) (Merck Millipore). For bacteria and mammalian culture medium, Milli Q water (resistivity of 18.2 MΩ cm at 25 °C) was used.
Synthetic oligonucleotides
The synthetic oligos were purchased from Macrogen. The oligos cover the CDS reported for amino acids 283-396 of the mature part of human BMP-2 (hBMP-2).
PCR_BMP2_fw: CCATGGCGCAGGCGAAACATAAAC
PCR_BMP2_rv: GGATCCTTATTAGCGGCAGC
B5_OLIGOFW:CCATGGCGCAGGCGAAACATAAACAGCGCAAACGCCTGAAAAGCAGCTGCAAACGCCATCCGCTGTATGTGGATTTTAGCGATGTGGGCTGGAACGATTGGATTGTG
B5_OLIGORV:GCATGGTTGGTGCTGTTCAGATGATCCGCCAGCGGAAACGGGCATTCGCCGTGGCAATAAAACGCATGATAGCCCGGCGGCGCCACAATCCAATCGTTCCAGCCC
B3_OLIGOFW:TCTGAACAGCACCAACCATGCGATTGTGCAGACCCTGGTGAACAGCGTGAACAGCAAAATTCCGAAAGCGTGCTGCGTGCCGACCGAACTGAGCGCGATTAGCATGC
B3_OLIGORV:GGATCCTTATTAGCGGCAGCCGCAGCCTTCCACCACCATATCCTGATAGTTTTTCAGCACCACTTTTTCGTTTTCATCCAGATACAGCATGCTAATCGCGCTCAGT
hBMP-2 synthetic gene construction and the DNA sequencing
To produce the synthetic gene that codes for hBMP-2 CDS, we followed a strategy of long primer synthesis and joined the fragments using DNA polymerase and PCR. The CDS was divided into two blocks, a 5’ end and a 3’ end. These blocks retained an overlapping region of 22 bases to facilitate the fusion of the blocks via PCR in a final step. Each block was generated by the annealing and elongation of two long primers that have a complementary region at the end of both oligonucleotides. The conditions for this reaction were: long primers 0.8 µM; Taq DNA polymerase 2.5 U/100 µL; Taq Buffer 1X (Thermo Fisher); dNTPs at 0.05 mM (Thermo Fisher). Incubation conditions in a Biorad C1000 cycler™ were: 95 ºC 1 min, 55 ºC 20 seg, 72 ºC 2 min. After the annealing step, DNA polymerase (Taq) could elongate the long primers upon the primed overlapping region and produced a dsDNA molecule of both blocks independently, a 5’ end and a 3’ end. At the final step, a PCR was performed as follows: 1 µL of the produced dsDNA of each block was added to the reaction as well as a PCR forward primer (PCR_BMP2_fw, 2 µM) directed the 5’ end of the 5’ block and a reverse primer (PCR_BMP2_rv, 2 µM) directed to the 3’ end of the 3’ block. The PCR conditions were: 50 µL final volume; Taq DNA polymerase 2.5 U/100 µL; MgCl2 1.5 mM; dNTPs at 0.2 mM; program: 95 ºC 1 min, (95 ºC 30 seg, 55 ºC 15 seg, 72 ºC 30 seg) x 25 cycles, 72 ºC 20 min. This final reaction produced a full-length synthetic CDS of hBMP-2 gene that was amplified due to the overlapping 22 bases region present within the 5’ and 3’ blocks. Furthermore, these last PCR primers also have the DNA target for the restriction enzymes NcoI and BamHI for the 5’ and 3’ends, respectively. The last PCR products were ligated into pTZ vector according to the manufacturer’s protocol (Thermo Scientific). The ligation reaction was carried out with 3 µL PCR products that were mixed with 1 µL pTZ plasmid plus 1 µL ligation buffer T4 DNA ligase and 1 µL T4 DNA ligase. The ligation reaction was incubated for 2 hr at room temperature. Afterward, the ligation product was transformed into 100 µL of XL1 blue E. coli cells. Transformed cells were spread out on LB agar plates supplemented with 50 µg/mL of kanamycin (Merck). The next day, ten colonies were cultured in 3 mL Luria Bertani broth supplemented with 50 µg/mL of kanamycin.
Plasmids from ten colonies were purified using the miniprep kit (Quiagen Spin miniprep kit Cat. No. 27104). Five microliters of each plasmid were mixed with 20 µL PCR master mix (2.5 µL 10 X Taq buffer, 2 µL of 25 mM MgCl2, 2 µL of 2 mM dNTPs, 1 µL of 2 mM PCR_BMP2_fw, 1 µL of 2 mM PCR_BMP2_rv and 1 µL Taq pol). The PCR reaction was performed to a standard PCR protocol. Positive PCR reaction was extracted, and their plasmids DNA were sequenced at Macrogen company.
rhBMP-2 production and purification
The pTZ/BMP-2 was digested with NcoI and BamHI restriction enzymes, and the fragment that contains the hBMP-2 CDS was cloned into the expression vector pET-28a (rhBMP-2) that carries a gene encoding kanamycin resistance. The expression vector construct pET-28a/hBMP-2 was transformed into E. coli BL21 (DE3) (Stratagene) cells. For purification, cells from 5L cultures were lysed by sonication at 300 watts for 5 min on ice (Sonics Vibra cell 750™ with Ti tip of ½ inch), and inclusion bodies were extracted and purified. Refolding of rhBMP-2 was performed according to published protocols by Ruppert and Colleagues in 1996 (Ruppert et al., 1996), except that SP-sepharose column was used for purification. The expression level of rhBMP-2 in E. coli was analyzed by SDS-PAGE using a mini-protean electrophoresis system (Biorad®). The protein folding and the purification of rhBMP-2 homodimer also were checked by SDS-PAGE. The rhBMP-2 bands were visualized by staining with Coomassie blue R-250. The fractions were pooled and concentrated with Amicon system® to 1 mg/mL. Afterwards, rhBMP-2 was lyophilized and stored at -40 °C until it was used.
Biological activity of rhBMP-2
Murine C2C12 skeletal myoblasts (<30 passages, obtained from the American Type Culture Collection, ATCC) were maintained in growth medium: Dulbecco’s Modified Eagle Medium (DMEM) (Sigma-Aldrich) supplemented with 10% fetal bovine serum (FBS) (Invitrogen) and 1% antibiotic-antimycotic (Invitrogen) in a 37 °C, 5% CO2 incubator. Osteoblast differentiation was induced by seeding C2C12 cells at a density of 50000 cell/mL in growth medium with different concentrations of rhBMP-2 and 300 ng/mL of commercial rhBMP-2 in a 48 well-plate at 37 °C in a 5% CO2 incubator™ (Thermo Fisher Schientific). After 3 days the cells were washed twice with PBS and lysed in 50 mM Tris pH 6.8, 0.1% Triton X-100, 2 mM MgCl2 buffer. The ALP activity of the lysates was assayed at 37 °C in 100 µL of 2-amino-2-methyl-l-propanol buffer, pH 10.3, for 15 min using p-nitrophenylphosphate (pNPP) (Merck) as a substrate. The reaction was stopped with 100 µL of 0.5 M NaOH and the absorbance was measured at 405 nm on a Varioskan™ LUX multimode microplate reader (Thermo Fisher Schientific). The enzyme activity was expressed as micromoles of p-nitrophenol (pNP) produced per min per µg of protein. The protein content was determined using the BCA protein assay kit (Sigma-Aldrich) using BSA as the standard.
Cell mineralization
To test whether the differentiated C2C12 cells could synthesize calcium nodules; completing their differentiation into functional osteoblasts, C2C12 cells were cultured with 3000 ng/mL of rhBMP-2 in mineralization media: DMEM, 10% FBS and 1% antibiotic-antimycotic, 1 mM of β-sodium Glycerophosphate (Sigma-Aldrich), 50 µg/mL of ascorbic acid (Sigma-Aldrich) and 10-8 M of Dexamethasone (Sigma-Aldrich). After 21 days in culture, cells were washed twice with PBS without Ca2+ and Mg2+, fixed with ethanol for 15 min and stained with 40 mM of alizarin red for 15 min to detect mineralization zones using a Nikon Eclipse TS100-F inverted microscope (NIKON). Osteoblastic cells Saos-2 (<30 passages, obtained from the American Type Culture Collection, ATCC) in mineralization media were used as a positive mineralization control. Mineralization percentage was calculated using ImageJ.
3-D model construction of BMP-2
Protein was visualized with Molecular Graphics Tool (PyMOL) (DeLano, 2002), and using the PDB file 3BMP.
RESULTS
BMP-2 synthetic gene construction and the DNA sequencing
The experiment to produce the synthetic CDS of the BMP-2 mature peptide was successfully performed as described in the methods section. The long oligonucleotides were successfully elongated and finally merged into a DNA molecule of 335 bp (Figure 1). The PCR product was ligated into the NcoI / BamHI sites of the expression vector pET28a. The insert sequence was confirmed by Macrogen service using capillary sequencing with the primers T7promoter and T7terminator (data not shown).
rhBMP-2 purification
The rhBMP-2 was expressed as an insoluble form in the IB of E. coli BL21. To recover the protein, the IB were sonicated using a cell disrupter (Sonics, VibraCell™ 750) at an amplitude of 40% and power of 300 watts. After purification, the concentration of IB of 20% of the wet weight of bacteria paste. The IB were solubilized as described by Rupper et colleagues, but instead of using 2-mercaptoethanol we used 5 mM DTT to obtain the protein. The protein was refolded using a redox system composed of oxidized and reduced glutathione. This process resulted in a BMP-2 concentration of 1 mg/ml. Afterward, about 30% of the total protein was visualized as a monomer and approximately 70% formed a dimer (Figure 2).
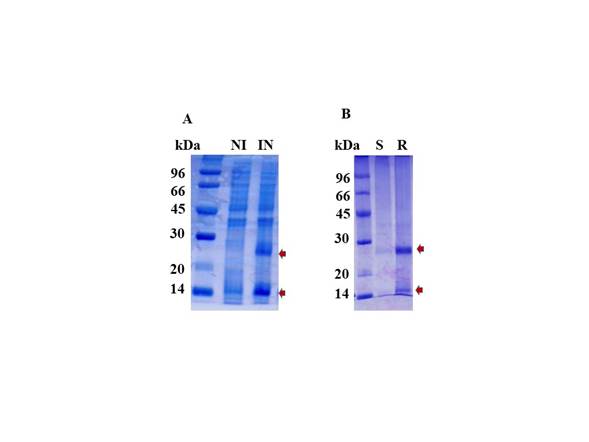
Figure 2 Analysis of the rhBMP-2 expression. (A) Induction of rhBMP-2 protein with IPTG. (B) Refolding of rhBMP-2 and purification by SP-sepharose. Protein was analyzed by 12% SDS-PAGE and stained by Coomassie Brilliant Blue. (kDa) molecular low marker, (NI) no induction, (IN) IPTG induction, (S) flow through from Amicon system, (R) concentrated hBMP-2 fractions by Amicon system after SP-sepharose purification. The red arrows show the rhBMP-2 dimer (26 kDa) and monomers (13 kDa).
SP-sepharose column was employed to separate the BMP-2 monomer from the BMP-2 dimer. Protein-bound to the column was eluted from the SP-sepharose column with a NaCl gradient from 200 mM to 1 M (figure 2). We observed that this solution contained possibly 80% of dimeric BMP-2. It means that after purification, the presence of monomers was highly reduced. After elution, BMP-2 was lyophilized and stored at -40°C. After the 2 L culture, 200 mg of wet IB, the protein recovery was 500 µg.
Biological activity of rhBMP-2
The refolded human BMP-2 bioactivity was tested by measuring ALP production in mouse myoblastic cell line C2C12. After 3 days in culture, there were statistical differences between cells cultured with 3000 ng/mL of our rhBMP-2 and without rhBMP-2 (controls), increasing ALP activity by approximately 137%. Additionally, we found significant differences between cells cultured with 300 ng/mL of commercial rhBMP-2 and controls. However, there were no statistical differences between cells cultured with our rhBMP-2 (3000 ng/mL) and commercial (300 ng/mL) rhBMP-2. According to these results, we were satisfied to prove that the rhBMP-2 derived from our synthetic CDS could induce the differentiation of C2C12 cells towards an osteogenic lineage (Figure 3 and Table 1).
Table 1 The bioactivity of different concentrations of hBMP-2 and 300 ng/mL of commercial BMP-2
Tukey's Multiple Comparison Test | Mean Difference | q value | Significant? p < 0.05? | Summary | 95% CI of difference |
---|---|---|---|---|---|
0 vs 50 | 0.2903 | 1.949 | No | Ns | -0.4172 to 0.9978 |
0 vs 300 | 0.002748 | 0.01845 | No | Ns | -0.7048 to 0.7103 |
0 vs 1000 | -0.4391 | 2.948 | No | Ns | -1.147 to 0.2684 |
0 vs 3000 | -0.8764 | 5.885 | Yes | * | -1.584 to -0.1689 |
0 vs 300° | -0.7116 | 4.778 | Yes | * | -1.419 to -0.004075 |
50 vs 300 | -0.2876 | 1.931 | No | Ns | -0.9951 to 0.4200 |
50 vs 1000 | -0.7294 | 4.898 | Yes | * | -1.437 to -0.02186 |
50 vs 3000 | -1.167 | 7.835 | Yes | ** | -1.874 to -0.4592 |
50 vs 300° | -1.002 | 6.728 | Yes | ** | -1.709 to -0.2944 |
300 vs 1000 | -0.4418 | 2.967 | No | Ns | -1.149 to 0.2657 |
300 vs 3000 | -0.8792 | 5.904 | Yes | * | -1.587 to -0.1717 |
300 vs 300° | -0.7143 | 4.797 | Yes | * | -1.422 to -0.006823 |
1000 vs 3000 | -0.4374 | 2.937 | No | Ns | -1.145 to 0.2702 |
1000 vs 300° | -0.2725 | 1.830 | No | Ns | -0.9800 to 0.4350 |
3000 vs 300° | 0.1649 | 1.107 | No | Ns | -0.5427 to 0.8724 |
CI: confidence interval, Ns: not significant
*p value <0.05, **p value <0.01
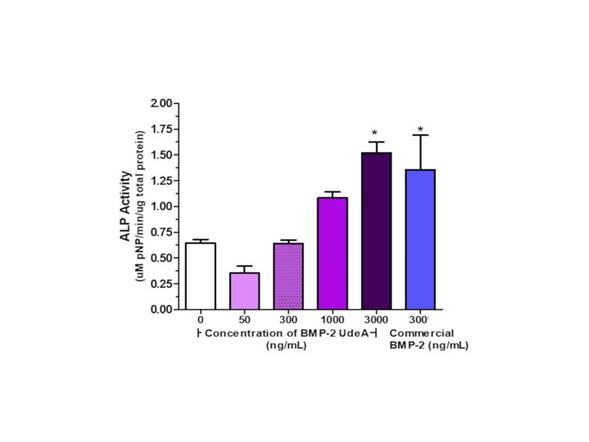
Figure 3 rhBMP-2 bioactivity. Refolded and SP-sepharose purified rhBMP-2 bioactivity was tested by ALP production, which hydrolysis p-NPP into pNP, per total protein content in C2C12 cells cultured for 3 days with different concentrations of rhBMP-2 (in house) and 300 ng/mL of commercial rhBMP-2. A representative experiment of three independent experiments. Mean + Standard error. Anova and Tukey's Multiple Comparison Test. * indicates statistical differences between concentrations of BMP-2 and 0, 50 or 300 ng/mL.
After 21 days in mineralization media, Alizarin red staining showed that both Saos-2 (osteoblastic osteosarcoma) and C2C12 cells induced with rhBMP-2 could synthesize calcium nodules (figure 4) mineralizing the surface 84% and 73%, respectively. This demonstrates that our rhBMP-2 can differentiate C2C12 cells into functional osteoblasts.
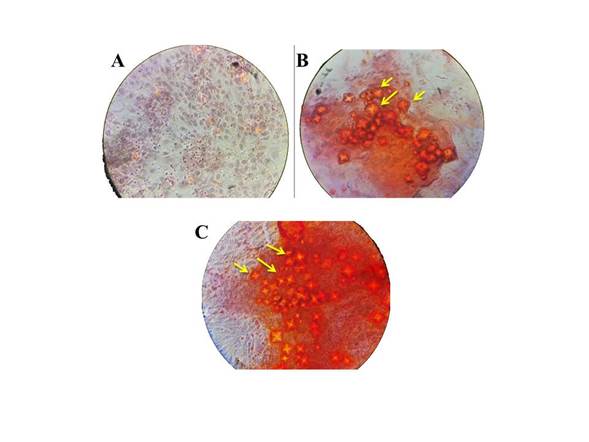
Figure 4 Effect of increased intracellular calcium nodules by rhBMP-2 treatment for 21 days on C2C12 and Saos-2 cells. Mineralized nodules formed by differentiated cells after incubation with rhBMP-2 were stained with Alizarin red (yellow arrows). (A and B) C2C12 cells in mineralization media with and without 3000 ng/mL of hBMP-2, respectively. (C) Saos-2 cells in mineralization media (positive control). 40 X magnification.
DISCUSSION
BMPs play a central role in bone formation and fracture healing. The FDA (food drugs administration, USA) has commercially approved two recombinant BMPs for clinical applications, among them rhBMP-2 (Durham et al, 2018; Krishnakumar et al, 2017). Our goal was to find a suitable protocol for cloning, expressing, and purifying rhBMP-2; which could serve as a basis for large-scale production processes. PCR-based synthesis of the human BMP-2 CDS, corresponding to the 283-396 amino acids of the mature region of the protein, proved here to be a low-cost, reliable and fast strategy (Park et al, 2019). The rhBMP-2 protein was efficiently expressed in E. coli under the control of the T7 promoter. The dimerization of the protein was achieved using the same refolding and the purification conditions employed by Ruppert and colleagues, except that we used an SP-sepharose column, instead of a CM-sepharose column to obtain the rhBMP-2 (Ruppert et al, 1996). Our results are in agreement with previous data, which reported efficient rhBMP-2 production in bacteria (Sharapova et al, 2010; Von Einem et al, 2010). The accumulation of rhBMP-2 in IB made necessary a refolding procedure, which is time-consuming, complicated and the yield is low. However, the interactions between all atoms in the amino acids sequence of BMP-2 and the buffer conditions supplemented with a redox system allowed that the majority of BMP-2 monomers reached the native structure of the protein and its corresponding free energy minimum (Figure 5) (Basak et al, 2019; Englander and Mayne, 2014). As a result, we obtained an active rhBMP-2 with 70% or more of purity. The rhBMP-2 could induce osteoblast differentiation of C2C12 cells at 3000 ng/mL (Figure 3 and Table 1). Although we needed higher doses of synthetic BMP-2 to induce the osteogenic differentiation of C2C12 cells, they are in line with reported doses in other studies (Fung et al, 2019; Ihm et al, 2008; Kashiwagi et al, 2009). When we compared the ALP activity of the commercial and our rhBMP-2 at 300 ng/mL, we found that our BMP-2, unlike the commercial, was not statistically different from the control. While at 3000 ng/mL we did not find statistical differences between the two. It is possible that impurities left after protein dimerization and purification using SP-sepharose cation exchanger column might be interfering with our protein; reducing its activity (Figure 3). Other studies use heparin chromatography to purify BMP-2 (Hettiaratchi et al, 2020; Xiong et al, 2008). In heparin chromatography purification, heparin acts as an affinity ligand for both the monomer and dimer of BMP-2. It also acts as an ion exchanger column that under increasing NaCl concentrations elutes either the monomer or the dimmer (Xiong et al, 2008). This dual action of the heparin column increases specificity of the process, obtaining a purer BMP-2. Considering this, in the future, we will use a heparin column and optimize the purification process to obtain the rhBMP-2 with a greater purity that needs lower doses to induce the osteogenic differentiation of C2C12 cells.
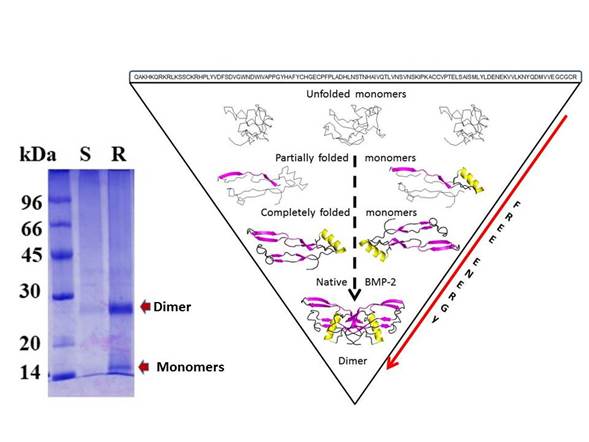
Figure 5 BMP-2 dimer. The SDS-PAGE for the monomers and dimer BMP-2 after chromatography purification (left side). A brief schematic for BMP-2 folding is shown (right side). All monomers started in the denatured state (amino acid sequence) (upper). After that, some monomers present secondary structures such as α-helix or β-sheets (medium). Finally, correctly folded monomers bound to form the BMP-2 dimer (bottom). These structures were visualized with PyMOL. The red arrow indicates a decrease in the free energy of monomers to reach the native state.
Despite these issues, the resulting rhBMP-2 is clearly functional and induces ALP activity in C2C12 cells, which can further differentiate into functional mineralizing osteoblasts. This means that the dimer of rhBMP-2 protein was folded correctly and can bind to its specific receptors, receptors type I (BMPR-Ia) and type II (ActRII) (Mueller & Nickel, 2012; Nickel & Mueller, 2019; Tabisz et al, 2017). Nevertheless, in the future, we will continue optimizing the protein purification procedure to obtain a purer BMP-2.
This work opens the possibility of scaling up the BMP-2 production to facilitate the study of bone tissue regeneration strategies. It also opens the possibility of expanding our biotechnology industry by synthetizing a functional protein which could be used to generate polyclonal antibodies for western blot applications, enzyme-linked immunosorbent assays, etc.