INTRODUCTION
Amphibians possess physiological and behavioral traits related to internal temperature control and skin permeability that shape vital processes and limit their distribution potential (Lillywhite, 2006). As ectotherms, their vital processes depend to a greater extent on their body temperature (BT), which is affected by the ambient temperature (Carey, 1978). BT acts as a controlling factor for many physiological processes such as oxygen consumption, heart rate, locomotion, water balance and digestion (Köhler et al., 2011). BT is also a controlling factor of behavioral processes such as movement in search of favorable environmental conditions of temperature and humidity (Kaufmann and Dohmen, 2016; Köhler et al., 2011).
BT in amphibians is determined by energy loss and gain by radiation, convection, conduction, as well as heat loss by evaporation (Maldonado-Castro, 2017; Navas, 1996a; Wygoda and Williams, 1991). Radiation, convection, and conduction depend on the environmental thermal gradient and evaporation depends on the vapor pressure that exists between the skin and the environment (Navas et al., 2008).
Many amphibians do not possess any type of resistance in their integument to water loss and therefore lose water by evapotranspiration in a manner similar to that of a body of water (Spotila and Berman, 1976). The permeability of frogs' skin confers to them an ability to carry out cutaneous respiration (Alveal-Riquelme, 2015), but also represents a risk of dehydration in terrestrial environments during some hours of the day under conditions of high solar radiation, temperature and low relative humidity (Carey, 1978). Therefore, water loss by cutaneous evaporation in amphibians is associated with a need for cooling, but thermoregulatory strategies also respond to the requirements of controlling their water content (Brattstrom, 1979; Wygoda and Williams, 1991; Navas et al., 2008). However, some terrestrial-arboreal species of the genus Phyllomedusa show low rates of evaporative water loss, which is considered a sign of adaptation in this genus (Shoemaker and McClanahan, 1975).
Understanding the thermoregulatory mechanisms of species is essential to understand the implications of microhabitat selection, as well as responses to disturbances in their thermal environment. However, study in this area is difficult to perform in living organisms and requires use of invasive techniques or non-invasive, but less precise techniques (Navas and Araujo, 2000).
An alternative is the use of agar models, from which it is possible to model water loss and body temperature both in field and laboratory conditions (Romero-Barreto, 2013; Navas and Araujo, 2000). In agar models, water evaporates at the same rate as a free surface water and therefore, represent a control in studies of water loss by evapotranspiration since they do not have any barrier to water loss (Romero-Barreto, 2013). These models simulate aspects such as the size and mass of living individuals, and therefore can be used to simulate the behavior of amphibian species in different environments (Spotila and Berman, 1976).
In this study, the Sabanera frog Dendropsophus molitor was used as a model. This hylid is endemic to Colombia, inhabiting the Andean region in the Eastern Cordillera from 1,900 to 3,600 meters above sea level. Dendropsophus molitor is commonly found in disturbed areas, such as pastures, roadsides, and populated centers primarily in the savannah of Cundinamarca (Navas, 2006). However, D. molitor currently faces challenges such as the presence of the fungus Batracochritium dendrobatidis, pressure from exotic species such as Lithobates catesbeianus (Guarnizo et al., 2014) and climate change (Kaufmann and Dohmen, 2016). The latter, is one of the causes of increased environmental temperature and climate variability, altering the thermal tolerance of amphibians, modifying activity patterns, dispersal and abundance (Corn, 2005; Méndez-Narváez, 2014; Navas et al., 2013).
Dendropsophus molitor has been the subject of several studies, due to its variation in size, coloration patterns and physiological and behavioral mechanisms (Amézquita, 1999; Guarnizo et al., 2014; Navas, 2006). Furthermore, D. molitor is confronted by drastic changes in temperature between day and night in extreme conditions, which can be unpredictable (Navas, 1996b). Dendropsophus molitor is eurythermic, since it supports a wide variation in environmental temperature and presents a heliothermic thermoregulatory behavior that allows it to reach body temperatures above the ambient temperature through daytime basking (Navas, 1996a; Navas et al., 2008; Valdivieso and Tamsitt, 1974). In addition, D. molitor has variation in body color patterns throughout the day, which potentially represents a thermoregulatory strategy (Amézquita, 1999; Carey, 1978). Dark colorations have been suggested to increase absorption of solar radiation and increase the rate of body temperature, which has been associated with species at higher elevations that experience large variations in environmental temperature (Carey, 1978). It has also been suggested that the effect of coloration is mediated by body size, which increases and decreases cooling and warming rates, respectively, with larger individuals exhibiting lower rates (Carey, 1978).
The change in coloration is considered a diurnal thermoregulation strategy, and a heliothermic behavior of the species that confers an ability to carry out other vital processes such as reproduction and feeding despite low temperatures (Navas, 2006). However, D. molitor could also increase their rate of warming and their risk of dehydration by being exposed to high ultraviolet radiation levels, and to warmer and drier substrates (Withers, 1995; Carey, 1978; Navas, 2006). Additionally, habitat degradation can change temperature and relative humidity conditions and have a synergistic effect in the extreme conditions of these habitats.
In this sense, the objective of the present work was to determine the effect of six climatic variables and microhabitat temperature on the percentage of weight change and body temperature in agar models of D. molitor with different colorations, in two microhabitats and in two microclimatic conditions. We aim to increase the knowledge of the thermal ecology of D. molitor in the grasslands surrounding construction, and to understand the potential consequences of environmental variation and climatic conditions on body temperature and percent weight change of the savannah frog.
MATERIALS AND METHODS
Study area
The study was conducted on the campus of Universidad Militar Nueva Granada (UMNG), located in the municipality of Cajicá at 4°56'70.5'' N, 74°00'70.4'' W, with an average temperature of 12-18°C, an average relative humidity of 82%, and an altitude of 2,550 meters above sea level (Angarita-Cañón, 2014; Trujillo-Pérez, 2017). Two different zones were sampled within UMNG between June 17 until August 4, 2017. The first zone corresponds to reservoirs (artificial lakes) located at 4°56'38.2'' N, 74°0'36'' W and the second to a natural wetland, near the wastewater treatment plant (WWTP) located at 4°56'20.28'' N, 74°0'34.33'' W, sites with presence of the species (Figure 1).
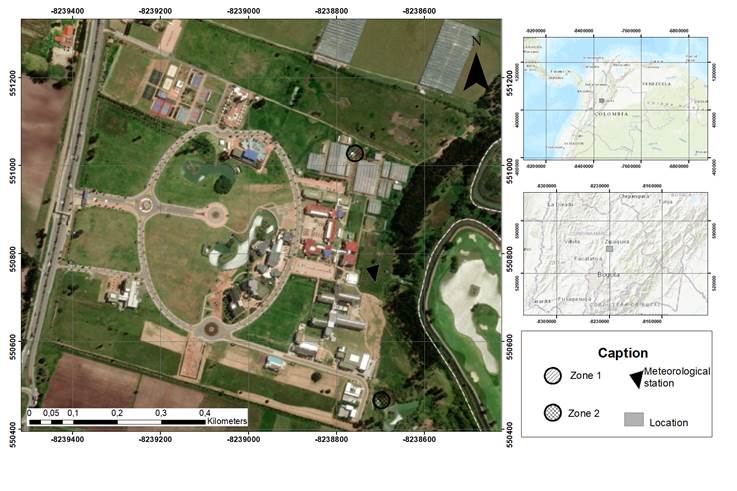
Figure 1 Geographical location of the areas sampled at the Universidad Militar Nueva Granada, Cajicá, Colombia. Zone 1: Reservoir; Zone 2: WWTP, and the location of the meteorological station.
Six climate variables derived from the UMNG IMETOS IMT280 weather station (Station No. 000008B8) were used (Figure 1). Weather station variables recorded were relative humidity (%), solar radiation (W/m2), precipitation (mm), ambient temperature (°C), wind speed (m/sec), and dew point (°C). Substrate temperature (°C) was taken at each sampling site with an infrared thermometer digital- Raytek DT-810 (Fixed emissivity 0.95; Accuracy( 0.4%).
Validation of agar models
To evaluate the effect of environmental variables on body temperature and percent weight change in the savannah frog D. molitor, agar models with the species mold were used (Navas and Araujo, 2000), following the protocol proposed by Sinervo et al. (2012) (Annex 1).
Evaluation of temperature acquisition and percent weight change in the models
Each agar model was placed in two contrasting microhabitats and at each sampling site, 2 brown molds and 2 green molds were used (Figure 2), which were changed during the day. The microhabitats were categorized under two conditions, a dry microhabitat 10 meters from the body of water, and a wet microhabitat 50 cm from the body of water (artificial lake or wetland). In each microhabitat, sites with direct sun exposure and without direct sun exposure (shade) were selected. These environments were chosen because active individuals have been observed at these sites during the day. To record the temperature of the agar models and the microhabitat (substrate temperature at 50 cm from the agar model), data were collected every four hours using a Raytek infrared thermometer and an OHAUS analytical balance. The four-hourly sampling was carried out based on a pilot test, which showed that the agar models did not lose their properties in terms of water loss capacity and thermal behavior.
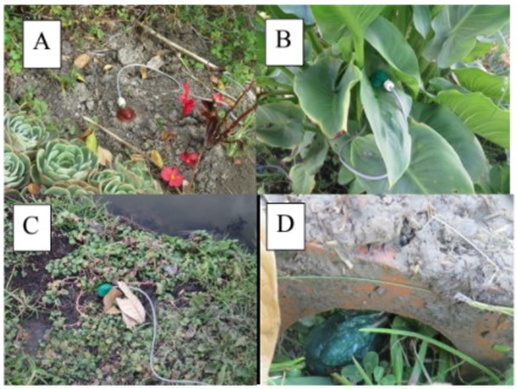
Figure 2 Location of hobos and agar molds in the four microhabitats chosen for Dendropsophus monitor. A and B: dry environments, C and D: environments near a body of water, A and C: sun, B and D: shade.
When evaluating water loss by skin evaporation and body temperature, the agar models were replaced by other models with the same molds. Four records were taken during the day for the first 15 days (6:00, 10:00, 14:00, 18:00) and then three records (8:00, 12:00, 16:00) for the following nine days, a total of 24 days. For the analysis of the percentage weight change (% weight change = (initial weight - final weight / initial weight) *100%)) in the models, the initial weight was recorded at the zero hour, and the final weight three or four hours later with an OHAUS balance of 0.001 accuracy.
Data analysis
For the validation of the agar model, temperature and percent weight change were measured simultaneously for three specimens of D. molitor and the agar models (Annexes 2 and 3). A Pearson correlation test was performed between the values obtained from the live individuals and the agar models; high values of association between the variables indicated similar thermal behavior between the agar model and the live individuals. In order to analyze the effect of agar model color (green vs. brown) on temperature and percent weight change of the model, a one-way ANOVA was used. All analyses were performed in R v 4.0.5 2020 (R Core Team, 2021).
A multivariate normality (MVN) test was performed on all variables and residuals, as well as a Mauchly test to test for sphericity with the MVN (Korkmaz et al., 2014) and Stats (R Core Team, 2021) packages, respectively. To test for differences in dispersal between microhabitats (dry and wet), a test for homogeneity of multivariate dispersal through permutations (PERMDISP) was performed with a Euclidean distance matrix, using the vegan package (Oksanen et al., 2013).
Since environmental variables may be correlated with each other, and to avoid multicollinearity in the model, the variance inflation factor (VIF) method was used, which implies that the standard errors of the parameters are inflated by the square root of 1/(1-Rj 2 ); a threshold of 3 VIF was established (Zuur et al., 2010). The variable that generated high collinearity in the dataset was removed (e.g., air temperature) and the remaining variables were used for data analysis. In order to identify whether there was a relationship between environmental variables (annex 4), microhabitat (dry vs. wet) and sunlight condition (sun vs. shade) on water loss by evapotranspiration and temperature of the agar model over time, a linear mixed effects model was performed using the lme4 package (Bates et al., 2019). This model allows for the consideration of repeated measurements over time, avoiding pseudoreplication and the inflation of the type I error rate (Whitlock and Schluter, 2015; Zuur et al., 2009). Moreover, it is a flexible model against violation of normality and sphericity assumptions. All analyses were performed in R v 4.0.5 software (R Core Team, 2021).
RESULTS
Validation of the agar models
In the validation of the agar models, it was observed that D. molitor and the models present a significant correlation for body temperature (p < 0.0001; CPearson = 0.931). With respect to the variable weight change percentage, being at the significance limit, it was assumed that a similar evapotranspiration behavior is presented between the agar model and D. molitor (p = 0.051, CPearson = 0.949). This corroborates that the agar model has a similar thermal behavior and percentage weight change relative to the D. molitor specimen.
Effect of agar model staining
Regarding the effect of the color of the agar models (green vs. brown), there was no significant effect on body temperature (ANOVA, F = 0.094; p = 0.761) and percent weight change of the models (F = 0.468; p = 0.506). Therefore, color was not considered to be an informative variable for the analyses.
Effect of climatic variables on agar models
The variables dew point, relative humidity, solar radiation, substrate temperature, condition (shade vs. sun) and interaction (microhabitat * condition) have a significant effect (Table 1) on weight loss and temperature of the agar model (wet-sun; wet-shade; dry-sun; dry-shade) over time (LMMs, p = 1.30 x 10-6 ; 0.001; 0.006; 4.25 x 10-7 ; 0.022 and 0.001, respectively).
Table 1 Results of the linear mixed effects model
Standard error | Df | t value | Pr (> |t|) | |
---|---|---|---|---|
Intercept | 0.137 | 10.012 | -0.120 | 0.907 |
Microhabitat dry. humid | 0.118 | 260.169 | -0.455 | 0.649 |
Shade and sun condition | 0.115 | 260.219 | 2.302 | 0.022* |
Dew point | 0.064 | 78.046 | 5.245 | 1.30e-06*** |
Precipitation | 0.043 | 262.921 | 1.049 | 0.295 |
Solar radiation | 0.105 | 198.465 | 2.769 | 0.006** |
Wind speed | 0.051 | 263.420 | -1.239 | 0.217 |
Substrate temperature | 0.082 | 264.178 | 5.187 | 4.25e-07*** |
Relative humidity | 0.091 | 77.663 | 3.538 | 0.001*** |
Microhabitat * Condition | 0.164 | 260.025 | -3.433 | 0.001*** |
Asterisk* refers to the significance of the test.
The increase in agar temperature is consistent with the increase in daytime temperature for both microhabitats, reaching maximum values at midday (Figure 3). Water loss and body temperature of the agar model is significantly higher in the sun condition and the dry microhabitat (Figure 4A), although high water loss was observed in the shade condition for the dry microhabitat (Figure 4B). Water loss and temperature change is consistent between days and hours sampled, with the highest water loss for both microhabitats (dry vs. wet) occurring between 12:00 and 16:00 hours, the hours of highest temperature increase of the study area (Figure 5).
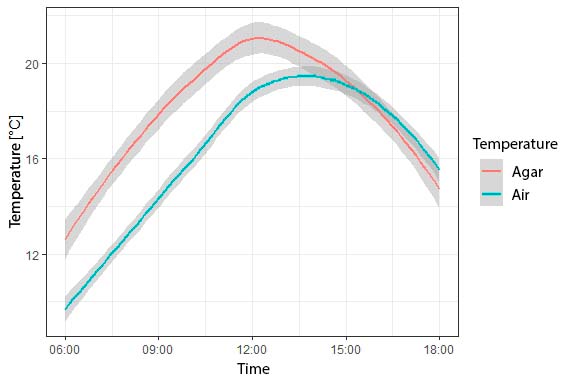
Figure 3 Air temperature and temperature of Dendropsophus molitor agar models in relation to time of day in the areas sampled at the Universidad Militar Nueva Granada, Cajicá, Colombia.
DISCUSSION
Effect of agar model staining
The results show that the two colors used in the agar models do not significantly affect the temperature and percent weight change. This result could be due to the fact that the concentration and range of the dye (payasite) used in the agar model did not allow to adequately simulate the color patterns of D. molitor, that the number of models for each color type in the microhabitats was low, and finally, the physical characteristics of the dye, such as the emissivity, is different from the frogs' skin, and the absorption of light of the agar models differs from the study organisms.
In contrast to our results, changes in skin color has been observed as a thermal regulation strategy in some species (Navas et al., 2008), especially for lowland species. An example of this is recorded in the gray tree frog (Chiromantis xerampelina), which changes to lighter colors when the environmental temperature increases, resulting in a lower rate of water loss, a mechanism to prevent overheating (Withers, 1995). In amphibians, when the environmental temperature decreases, they change to a dark coloration, resulting in a body temperature close to the environmental temperature (Withers, 1995). The above shows that skin color changes are temperature dependent, and result in solar absorption at low temperatures and solar reflection at high temperatures (Navas et al., 2008; Withers, 1995). Therefore, BT and water loss are influenced by skin color patterns.
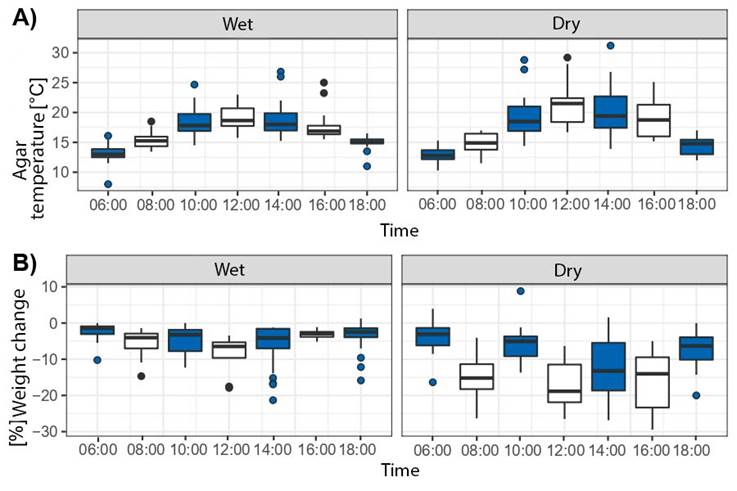
Figure 5 Variation of temperature (A) and percent weight change (B) of the agar models with respect to time of day, discriminated between wet and dry microhabitats at the sampling sites at the Universidad Militar Nueva Granada, Cajicá, Colombia. The first and second sampling is highlighted in blue and white, respectively.
In addition to color, altitude is important, as shown by Percino-Daniel et al. (2021) in a multispecies approach in the genus Craugastor, where lowland populations were compared to highland populations. Elevation was found to play a fundamental role in regulating BT and water loss; lowland populations were more susceptible to temperature fluctuations. In the case of D. molitor, an interesting question to address would be: in medium and high elevation species, if color has a fundamental role in regulating body temperature. To address this, it would be necessary to increase the number of agar models and test different concentrations and color ranges to simulate the color patterns of this species in agar models (Guarnizo et al., 2014; Tattersall et al., 2006).
Climate variables and agar models
Our results show that the temperature of the agar models was higher than the air temperature (Figure 3). Therefore, it can be corroborated that D. molitor has a heliothermic behavior previously described by Navas (1996a), since it basks in the sun to raise its body temperature. Amphibians that exhibit this behavior dehydrate quickly and must adopt a posture to conserve water when exposed to the sun (Mitchell and Bergmann, 2016), a posture where the ventral skin comes in contact with the substrate, the limbs are kept under the body and the head in a low position (Withers, 1995). This behavior has been observed in D. molitor individuals from the UMNG campus in Cajicá.
On the other hand, exposure to solar radiation, substrate temperature, dew point, relative humidity (Annexes 4 and 5) and microhabitat type each affect the percentage of weight change related to body temperature, especially in microhabitats with exposure to solar radiation (Figure 4) (Maldonado-Castro, 2017; Navas, 1996a). Solar radiation is related to substrate temperature (Annex 6) and these variables in turn have a direct or indirect effect on body temperature and water loss by evapotranspiration on a temporal scale (Angilletta et al., 2010).
In this study, the microhabitat substrate temperature affected the BT of the agar model (annex 4), which is concordant with what was found in the species of the family bufonidae Anaxyrus compactilis and Incilius occidentalis, in the species of the family Hylidae Dryophytes eximius and D. arenicolor, and in the species of the family Ranidae Lithobates neovolcanicus (Leyte-Manrique et al., 2018). The above shows that both in the experimentation of agar models and that performed directly with specimens, these models behave as thermoconformists given that they depend on the conditions offered by the environment.
Regarding the sampling schedule, the hours with the highest values of body temperature and the highest dehydration of the agar molds of D. molitor are 12:00 and 16:00 hours (Figures 3 and 5). The water loss is possibly due to a cooling strategy by evapotranspiration during the hours of highest solar radiation and therefore with the highest environmental temperatures (Sanabria et al., 2003). However, evaporative cooling by the amphibian causes a high metabolic cost in exchange for maintaining an optimal temperature (Alveal-Riquelme, 2015; Maldonado-Castro, 2017; Sanabria et al., 2003), the time dedicated to thermoregulation is important to tolerate water loss (Alveal-Riquelme, 2015; Sanabria et al., 2003).
The results show that several variables, such as microhabitat temperature or ambient temperature, solar radiation and relative humidity, have effects on water loss and body temperature of the models. These results are similar to those observed in the work of Sanabria et al. (2003), where the variables environmental temperature, solar radiation and wind speed showed a significant effect on body temperature. In contrast, the studies of Navas et al. (2013) and Rueda-Solano et al. (2016) found an association between body temperature and altitude gradient.
On the other hand, dew point variable was found to be related to body temperature and the percentage of weight change of D. molitor (Annexes 4 and 5). This may be due to the fact that in these Andean frogs, dew is a very important variable to compensate for the high temperature, since it is one of the available forms of water in the environment (Martines and Lira, 2008).
This study suggests the need to incorporate more variables in addition to environmental temperature in ecophysiological studies, such as solar radiation, substrate temperature, dew point, relative humidity, precipitation, wind speed and microhabitat, because they can have a strong effect on the thermal behavior of the species to be studied (Alveal-Riquelme, 2015; Maldonado-Castro, 2017).
CONCLUSIONS AND RECOMMENDATIONS
Although D. molitor presents different coloration patterns which can be affected by environmental temperature, where variation in coloration depends on environmental temperature (Guarnizo et al., 2014; Tattersall et al., 2006), in our agar models, coloration variation was not significant for BT or water loss. Dendropsophus molitor has a heliothermic behavior and is therefore susceptible to dehydration. The importance of including additional variables to the environmental temperature is evident, because they can be better descriptors of the thermal behavior and percentage of weight change of the species. This work highlights the need to monitor these populations over time to evaluate the behavior of abiotic variables on campus, and their effect on body temperature and the percentage of weight change of the savannah frog. This knowledge allows us to think in the long term about conservation strategies for the species on the campus, such as planting shrubs and trees that favor the niches that the species use.