Services on Demand
Journal
Article
Indicators
-
Cited by SciELO
-
Access statistics
Related links
-
Cited by Google
-
Similars in SciELO
-
Similars in Google
Share
Caldasia
Print version ISSN 0366-5232On-line version ISSN 2357-3759
Caldasia vol.30 no.1 Bogotá Jan./June 2008
CARLOS ALBERTO RIVERA-RONDÓN
SAÚL PRADA-PEDREROS
DIANA GALINDO
JAVIER A. MALDONADO-OCAMPO
Unidad de Ecología y Sistemática, Departamento de Biología, Pontificia Universidad Javeriana, Cra. 7 No. 40-82, Bogotá, Colombia. crivera@javeriana.edu.co; saul.prada@javeriana.edu.co; dianaguribe@yahoo.com
Programa Inventarios de Biodiversidad, Instituto Alexander von Humboldt, Claustro de San Agustín, Villa de Leyva, Boyacá, Colombia. jamaldonado@humboldt.org.co
ABSTRACT
G. bogotensis has a geographic distribution restricted to the Colombian Cundiboyacense plateau, it is listed as near threatened, and research on its autoecology is scarce. Three collections were made in 2006 in the Fúquene Lake, Cundinamarca, Colombia (5° 27' 55'' N, 75° 46' 19'' W) to describe the habitats occupied by G. bogotensis and to determine its vertical and horizontal distribution. Three sampling zones were selected according to the type of dominant macrophyte (Eichornia crassipes, Schoenoplectus sp. and Egeria densa). In each sampling zone two different cylindrical sampling traps (cloth and PVC) were placed at three depths: surface, mid-depth and bottom. Three replicates were used for each depth and type of trap. Traps were exposed for 24 hours and checked every 6 hours. In addition to the traps, sampling by electrofishing was conducted in each sampling zone during every month. To characterize the study area, physical and chemical variables were analyzed and the structure of phytoplankton, zooplankton, periphyton, and macroinvertebrate communities was studied. Results showed spatial differences on G. bogotensis habitat occupation and differences in captures at each depth, which depend on the dominant type of aquatic vegetation and size of individual. We conclude that only cylindrical cloth traps are suitable to conduct population studies of G. bogotensis.
Key words. Grundulus bogotensis, aquatic macrophytes, spatial distribution.
RESUMEN
Grundulus bogotensis tiene una distribución restringida al altiplano Cundiboyacense, se encuentra en la categoría "casi amenazada" y son escasos los trabajos realizados sobre su autoecología. Con el objeto de describir los hábitats ocupados por G. bogotensis y determinar su distribución vertical y horizontal en la laguna de Fúquene, Cundinamarca, Colombia (5° 27´ 55" N, 75° 46´ 19" O, 2560m), se realizaron tres muestreos durante el 2006 en tres zonas que se definieron en función del tipo de macrófita dominante (Eichhornia crassipes, Schoenoplectus sp. y Egeria densa). En cada uno de estos puntos se utilizaron dos tipos de trampas (cilíndricas de tela o de PVC) ubicadas en tres profundidades (superficie, medio, fondo); en cada profundidad y tipo de trampa se utilizaron tres réplicas. Las trampas estuvieron expuestas durante 24h y fueron revisadas cada 6h. Adicionalmente se realizaron muestreos con eletropesca en cada uno de los sitios de muestreo y durante cada mes. Para caracterizar las zonas de estudio, se analizaron variables físicas y químicas y se estudió la estructura de las comunidades de fitoplancton, zooplancton, perifiton y macroinvertebrados. De acuerdo con los resultados existen diferencias espaciales en la ocupación de G. bogotensis y diferencias en las capturas realizadas por profundidad que son dependientes del tipo de vegetación acuática dominante y de la talla del individuo. También se concluye que sólo las trampas cilíndricas de tela son adecuadas para realizar estudios poblacionales de G. bogotensis.
Palabras clave. Grundulus bogotensis, macrófitas acuáticas, distribución espacial.
INTRODUCTION
Plant communities are an important component of aquatic systems, creating a structurally complex environment for fish communities (Tonn & Magnuson 1982, Anderson 1984). Macrophytes provide adequate substrates for the development of aquatic organisms on which fish depend (Diehl 1993) and provoke modifications on physical and chemical water conditions, with the subsequent effect on structure of fish communities (Meerhoff et al. 2002, Petry et al. 2003, Soares et al. 2006).
The presence and type of macrophytes affect mobility and foraging strategies of fish (Baker & Ross 1981, Greenberg et al. 1995, Schindler & Scheuerell 2002). Differences on spatial distribution between juveniles and adults have been associated to habitat structural characteristics related to the presence of vegetation (Werner & Hall 1988, Lewin et al. 2004).
Large areas covered by macrophytes, reduction of water surface, loss of flooding pulses, and sedimentation due to industrial and agricultural activities are some of the major changes observed in lakes and wetlands in the Andean region (Donato 2004). The Bogotá plateau is one of the areas in the Andean region that has experienced more alterations, and open water surfaces of its aquatic systems have been replaced by dense covers of submerged, rooted and floating macrophytes (Chaparro 2003, van der Hammen 2003).
Grundulus bogotensis is one of the three endemic species that still occur in the Bogotá plateau (Eigenmann 1920) and is currently listed as ‘near threatened' because of its occurrence in one of the most transformed zones of Colombia (Mojica et al. 2002). This species used to be an important food source for local people and is still exploited by some communities.
Studies on the regional distribution of G. bogotensis are scarce. Eigenmann (1920), Miles (1947), Dahl (1971) and Maldonado-Ocampo et al. (2005) made comments on its distribution. The species has been introduced into other Andean aquatic environments (Alvarado & Gutierrez 2002, Maldonado-Ocampo et al. 2005). Biology and taxonomy of G. bogotensis were studied by Forero & Garzón (1974), González-Acosta (1992), Mora et al. (1992), and Román-Valencia et al. (2003, 2005). There are no studies on habitat occupation carried out with appropriate fishing techniques.
Considering the few studies published on the autoecology of this fish and the recent changes of the environments where the species lives, the following objectives were proposed in our study: (1) identifying and describing habitats occupied by G. bogotensis in the Fúquene lake, (2) finding relationships between its vertical and horizontal distributions and habitat structure, (3) determining any differential spatial occupation depending on the size of individuals, and (4) comparing the efficiency of two fishing techniques.
Study area
The Fúquene lake is located in the Cundiboyacense plateau (5° 27' 55'' N, 75° 46' 19'' W, 2,560 m in altitude), has an approximate area of 1,300 ha, a maximum depth of 6 m and an average depth of 2 m. The lake's principal inflow source is the Ubaté stream and its outflow source is the Suárez stream.
The lake has experienced a progressive deterioration over the last years as a consequence of an increased nutrient load from the drainage basin (Donato 1998). Studies conducted by JICA-CAR (2000) show that livestock farming accounts for 80% of the polluting load discharged into the lake and that 60% of its surface has been lost in the last 60 years. The agricultural and livestock farming land use has caused the drying of the lake during the last years (Donato 2004). Eutrophication has led to an increased cover of floating plants (Eichhornia crassipes), rooted plants (Schoenoplectus sp) and submerged rooted plants (Egeria densa), which have covered almost the entire lake.
Materials and methods
Three samplings were conducted between July and September 2006 to study the spatial distribution of G. bogotensis. Three different zones were selected and defined according to the dominant macrophyte type: E. crassipes (buchón), Schoenoplectus sp. (junco) or E. densa (elodea) (Figure 1). The selected zones show a varying depth of 1.5-2.2 m. Two types of traps were located in each of the three zones, at three different depths (surface, mid-depth, and bottom). Three replicates were set at each depth and for each type of trap.
Type A trap was a cylinder 50 cm long and 15 cm wide, of 0.7 mm-pore white cloth, with two funnels (one at each end of the cylinder) with a 4-cm wide inner opening. Type B trap was a dull-coloured PVC cylinder 50 cm long and 15 cm wide, having one funnel in one end of the cylinder, with a 4-cm wide inner opening, and the opposite end closed with nylon mesh. Traps were exposed without any bait during 24 hours and checked every 6 hours.
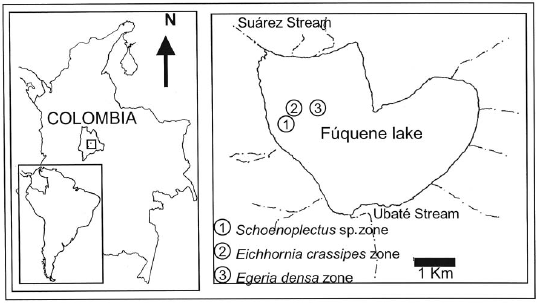
Study of the horizontal distribution was complemented with samplings using electrofishing equipment for 30 minutes at each of the sampling zones. We followed the recommendations by Maldonado-Ocampo et al. (2005) on methodology and equipment specifications.
Collected specimens were preserved in 10 % formalin for 72 h and then transferred to 70 % ethanol. Weight and length (standard and total) were recorded in all specimens prior to their deposition into the Fish Collection of the Museo Javeriano de Historia Natural (MPUJ) in Bogotá and the collection of the Instituto Alexander von Humboldt (IAvH-P) in Villa de Leyva, Boyacá.
In order to have a limnological characterization of the lake, pH, conductivity (Oakton pHCond10), oxygen and surface water temperature (Oakton oxymeter) were measured during sampling times. Samples of surface phytoplankton, periphyton, zooplankton and macroinvertebrates were collected. Physical and chemical vertical profiles were done during the September sampling.
Water samples of 200 ml were collected from the sampling zones with free water to study phytoplankton. Samples were preserved in 1% Lugol's solution. Subsurface water samples (1-10 L) were filtered with a 20 µm-pore mesh and samples were preserved in Transeau solution to study zooplankton. Periphyton was sampled by squeezing marshy vegetation and collecting sediments accumulated on plants. Periphyton samples were preserved with 1% formalin. Macroinvertebrate communities associated to macrophytes were sampled with a 900-cm2 hand mesh and samples were preserved in 70 % ethanol.
Phytoplankton was quantified using the sedimentation method and counting under an inverted microscope (Wetzel & Likens 2000). Periphyton was quantified by counting 400 cells of the dominant morph type under an optical microscope. Zooplankton samples were analysed under an optical microscope and a stereoscope with 1 ml chambers. Macroinvertebrate samples were cleaned and observed under a stereoscope for their identification. Specimens from each community were determined down to the lowest possible level using specialized taxonomical keys.
Results
Limnological characterization. Zones dominated by Schoenoplectus sp and E. crassipes showed a tendency to have water pHs from weakly acid to neutral, while zones dominated by E. densa showed alkaline water pHs (Table 1). Zones dominated by E. densa had oxygen values > 70 % in saturation and values for the other two zones were < 30%. Temperature records were highest during the September sampling, mainly in the E. densa zone.
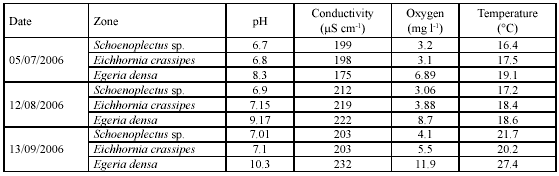
Vertical oxygen profiles exhibited spatial differences. In the zone with Schoenoplectus sp. dominance, values were < 5 mg l-1 within the entire water column; in the zone dominated by E. crassipes, values ranged between 4 and 7 mg l-1 within the first meter in depth; and values in the zone dominated by E. densa showed a variation between 4 and 12 mg l-1 within the first meter in depth (Figure 2).
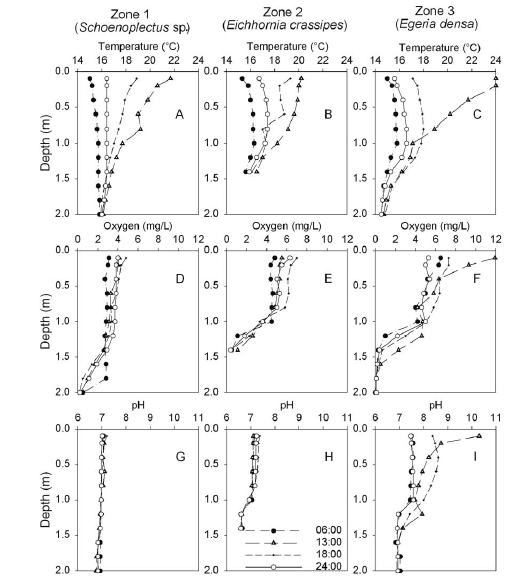
Conductivity values were relatively homogeneous in the entire water column, while pH, temperature and dissolved oxygen showed a vertical gradient that varied during the day/night cycle (Figure 2). During the daytime, the water column showed a progressive increase in temperature in the three zones. Additionally, we observed an increase of pH and oxygen values in the zone dominated by E. densa in the daytime.
Phytoplankton revealed a large variation in density of the main groups between zones and samplings but always with a dominance tendency of Bacillariophyceae and Cyanophyceae (Figure 3). Algal density varied between 400 and 2,400 cell ml-1 and zooplanktonic density ranged from 10 to 200 ind. l-1. Rotifers were the dominant group followed by cladocerans and copepods as codominant groups. An increase of the zooplankton density was recorded in August.
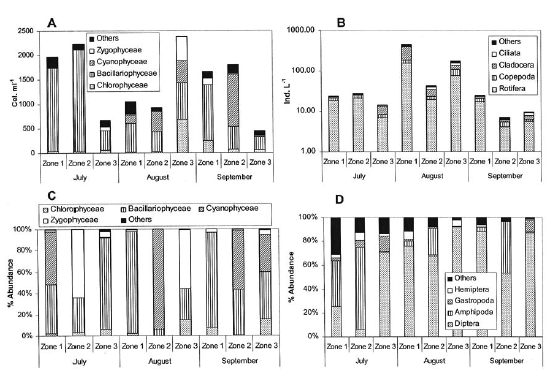
The periphytic algal community was dominated by Bacillariophyceae, Cyanophyceae and Zygophyceae. In general, no pattern of temporal or spatial variation was observed in the dominance by these groups. The benthonic macroinvertebrate community was dominated by dipterous, mostly of the family Chironomidae. In the zone dominated by E. crassipes we observed Hyalella sp (Amphipoda) as the most important macroinvertebrate, while the zone dominated by Schoenoplectus sp contained a higher diversity of groups and, in general, a higher richness of morph type. Structural differences of the dominant vegetation in each zone did not allow an adequate estimation of macroinvertebrate density; however, total specimens collected in the zone dominated by Schoenoplectus sp were twice lower than those collected in the other zones.
Efficiency of the fishing techniques. Captures of G. bogotensis with type A trap were significantly higher than captures with type B trap in all the three samplings (Table 2, Figure 4). Total specimens captured with type A trap were 472 and with type B trap 63 (after 72h of exposure). Electrofishing allowed capturing 436 specimens after a 4.5-hour effort.
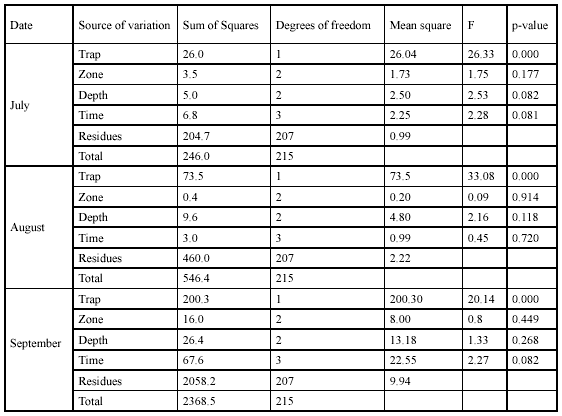
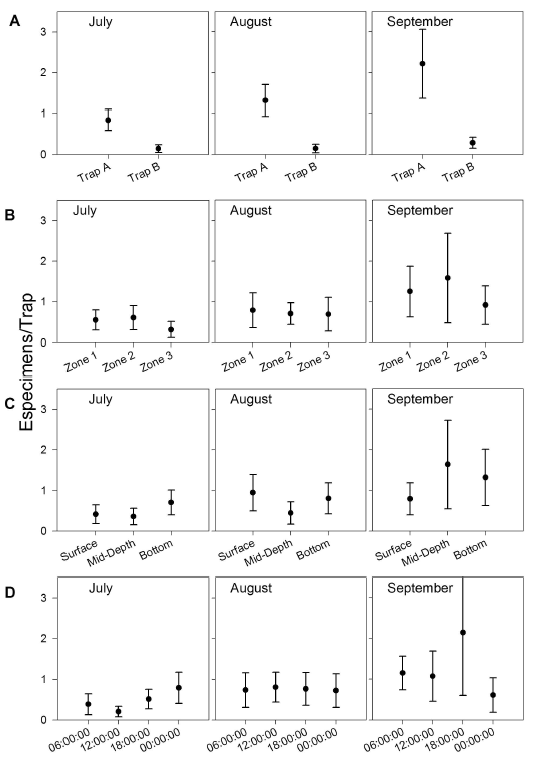
Spatial and temporal variation in G. bogotensis captures. An Analysis of Variance revealed no significant differences in captures between zones using traps (Table 2, Figure 4). However, total captures with traps was higher in zones dominated by Schoenoplectus sp and E. crassipes (Table 3). The highest capture scores with electrofishing were obtained in the zone dominated by E. densa (Table 4).
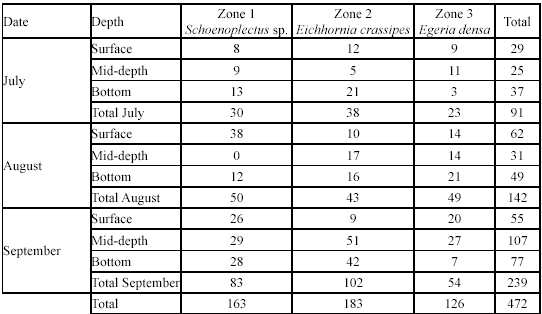
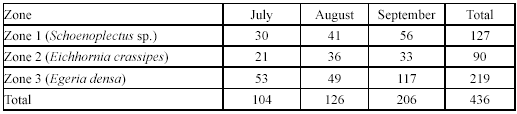
Although no significant differences were found either between captures at different depths, there was a numeric tendency to have higher capture scores in deep samples, particularly in the zone dominated by E. crassipes.
Type A traps and electrofishing showed the highest capture levels in September (Table 3 and table 4). There was a tendency to have higher captures at 6:00 h and 18:00 h and lower captures at 12:00 h and 24:00 h (Table 5), but no significant differences between hours were found.
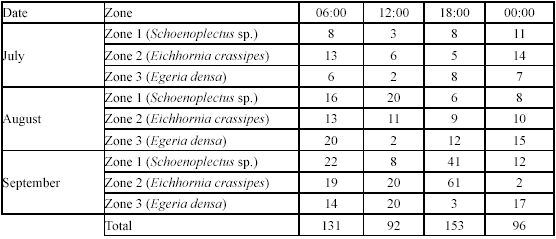
Spatial and temporal variations in G. bogotensis size. Size analysis of captured specimens revealed differences between traps and electrofishing. Traps showed higher numbers of captures of specimens between 25 and 40 mm in standard length, while electrofishing allowed capturing specimens mainly with one of two distinct sizes: 10-20 mm and 30-40 mm (Figure 5).
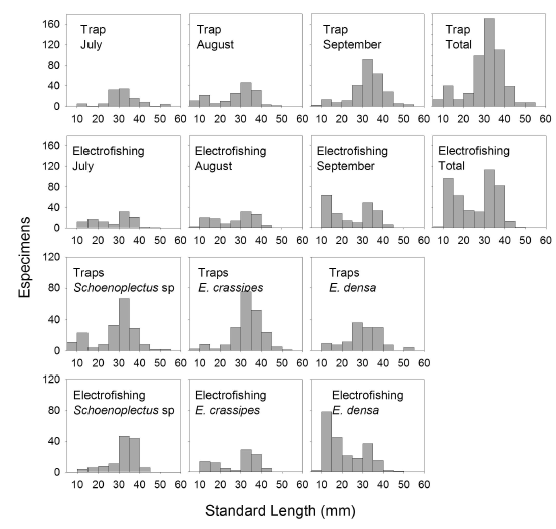
Differences on size selectivity between the two fishing techniques were observed also on a spatial basis. Electrofishing showed a tendency to higher captures of small specimens in the zone dominated by E. densa and the zone dominated by E. crassipes compared with capture results from traps. On the contrary, in the zone dominated by Schoenoplectus sp, captures by electrofishing were mainly of large specimens (30-40 mm in size), although small specimens (5-15 mm) were also captured with traps.
Size analysis of specimens captured with traps indicated that the highest numbers of small- sized specimens were obtained at 12:00 h and 18:00 h (Figure 6) and that the smallest specimens were captured mostly with surface traps.
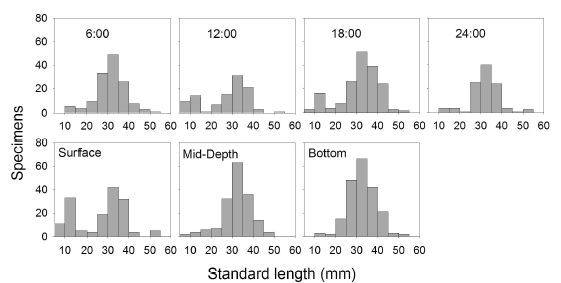
DISCUSSION
The limnological characterization shows that Fúquene is an eutrophic lake. Conductivity scores are high, indicating high levels of organic matter mineralization (Wetzel 2001). Dominance by macrophyte communities corroborates a high eutrophic status, in which nutrients accumulate in plant biomass and control by herbivory is poor (Carpenter et al. 1997). In general, there is a high variation between sampling zones and sampling months as a consequence of the system's trophic status and physical heterogeneity.
The pH scores were highly variable between depths and zones. The zone with E. densa showed very high values compared to other aquatic ecosystems in the Andean region (Donato et al. 1996, Donato 2001, CI-EAAB 2005), indicating an increased primary production.
Both the high eutrophic status of the lake and the presence of aquatic plants throughout its entire water mirror, create environmental conditions suitable for the development of periphytic communities and the maintenance of large amounts of particulate organic matter. Despite difficulty in calculating the percentage of contribution by periphytic algae to the total primary productivity of the ecosystem, it has been reported that algal communities may contribute with the largest proportion of fixed biomass in lentic aquatic systems (Vymazal 1995, Goldsborough & Robinson 1996).
Submerged plants such as E. densa favour the development of extensive sheets of periphyton by having a larger exposed surface area. Such communities are more efficient in nutrient retention and therefore exhibit a higher productivity and favour the development of collector and foraging invertebrate communities (Wetzel 1996). Sheets of periphytic algae are important components of food webs in aquatic systems of the Bogotá plateau; carbon and nutrient flows become faster in absence of herbivorous fish.
On the other hand, high organic matter production by emerging plants as Schoenoplectus sp. and floating plants as E. crassipes, creates anaerobic conditions favouring phosphorous availability (Esteves 1998) and subsequently an increase in the ecosystem productivity.
However, anoxic conditions in tropical aquatic systems may favour denitrification (Lewis 2002) and primary production controlled by cyanophyceous algae. Since these algae are poorly palatable (Margalef 1983) and the strong limitation of light due to macrophyte cover, trophic structure can be organized on a decomposer system. These conditions favour the development of dense communities of collector and detritivorous macroinvertebrates with the subsequent increase in food availability for G. bogotensis.
A shallow water column and the nictimeral nature of temperature suggest a polymictic circulation pattern and a tendency to anoxia in deep water layers (Lewis 1996). The eutrophic status of the water mass, the shallow depth of the basin and the high density of aquatic vegetation, allow an increase in oxygen concentrations in the epilimnion during the day and a fast reduction during the night. In general, tropical lakes tend to have oxygen deficits as a result of constant annual temperature and radiation and the higher activity of microbial communities (Lewis 2000). As a consequence, the presence of a large biomass of aquatic plants affects water chemistry by intervening on the sedimentation process and the carbon flow within the ecosystem (Esteves 1998).
Hypoxia in deep water layers represents an important factor for fish populations, considering that oxygen values were <1 mg l-1 near the bottom. G. bogotensis individuals were found dead in a few occasions, mainly inside traps placed on the bottom of the lake. Although no reports exist on G. bogotensis adaptations to hypoxia, the impossibility for fish to reach the more oxygenated surface water layers while they remained trapped can explain their death. Many fish species living in tropical waters with little oxygen exhibit adaptations in their morphology, physiology or behaviour (Soares & Junk 2000). Laboratory experiments have revealed that lethal concentrations for some tropical fish are below 0.6 mg l-1 when exposed to a deficit of several hours (Junk et al. 1997, Soares et al. 2006). However, there are no data on G. bogotensis ability to withstand anoxia.
Oxygen concentrations in the Fúquene lake were > 2 mg l-1 above 1-m depth and averaged 5 mg l-1 within that water layer. This finding suggests that the permanency of G. bogotensis in deep layers may be restricted to short time intervals and individuals go to upper layers to avoid remaining exposed too long to hypoxia. Verification of this hypothesis requires sampling with cloth traps of larger pore size that facilitate water exchange and eliminate any influence by the trap design.
Currently there is no fishing device properly designed to determine distribution patterns and habitat preference by G. bogotensis. Efficiency of conventional fishing techniques may involve considerable habitat damage or poorly selective biotope samplings. Cloth traps were more effective than PVC traps due probably to their two openings instead of one. However, other characteristics such as their white colour and less rigid structure might have favoured their efficiency.
Electric fishing was a very efficient method since it produced a number of captures similar to those of traps but with much less effort. Despite electric fishing data provided valuable information on size and horizontal distribution of G. bogotensis, they did not provide information on vertical distribution and activity changes at different times in the day. Electric fishing has been regarded a much more robust method in fish community studies due to its low selectivity by electric current affecting all individuals. However, electrofishing, as well as traps, was poorly effective in capturing other fish species that occur in the lake according to field observations and local people, suggesting that differences in fleeing strategies and location within the water column strongly affect the effectiveness of that method.
Only two Eremophilus mutisii individuals were captured by electrofishing and none with traps. Pinilla & Abril (1996) experienced difficulties in capturing this species contained in artificial ponds where it had been introduced. They tried with no success different nets and bottom traps and finally decided to drain the ponds.
Although no significant differences were found between zones, times and depths, there was a tendency to find more individuals in deep traps with a higher activity at 6:00 h and 18:00 h and a lower activity at 12:00 h and 24:00 h. This result indicates temporal differences in space occupation by G. bogotensis.
Absence of statistically significant differences is related to the differential response of fish according to size. Based on the assumption that electric fishing is less selective, results suggest that smaller fish are found in zones with E. densa dominance as well as being active during the daytime and located mainly in the surface. Differential distribution of sizes is related to variation in oxygen profiles between zones. According to the results obtained by electrofishing, the zones having higher concentrations of oxygen (E. densa and E. crassipes zones) showed more captures of individuals < 20 mm in standard length.
However, differences in spatial distribution may be related to differences in the type of dominant resource in each different plant cover. Higher structural complexity provided by submerged macrophytes favours higher densities of benthonic macroinvertebrates. Little foraging efficiency in more complex environments as those with submerged macrophytes is compensated by a higher productivity of preys (Diehl 1993). Another explanation is related to differences in predation risk posed by fish size. Smaller fish can be more easily predated in free waters (Werner & Hall 1988); therefore submerged macrophytes offer a well sheltered environment (Lewin et al. 2004).
In conclusion, G. bogotensis exhibits temporal and spatial differences in abundance, which in turn are related to the size of individuals. Smaller specimens occupying the zone dominated by E. densa suggest that submerged macrophytes in wetlands can play a very important role in providing shelter, high oxygen concentrations and food availability. Further studies covering wider sampling windows and including more replicates will serve to test our hypothesis.
The large physical, chemical, and resource variations of the environment where G. bogotensis lives, offer an opportunity to deepen our knowledge on the species tolerance to extreme pH and oxygen conditions. We need also to assess the direct relationships between habitat complexity, resource availability and space use. Understanding these relationships will acquire importance for future habitat design and rehabilitation plans to promote the maintenance of viable G. bogotensis populations.
ACKNOWLEDGMENTS
Financial support was provided by the Colombian Research Grants Program Jorge Ignacio Hernández Camacho-Initiative for Threatened Species (IEA-CO157) and the Pontificia Universidad Javeriana (Id399-No.1922). Authors are grateful to: Instituto Alexander von Humboldt for providing logistic assistance; Wilson and Julio Pachón, Vladimir Paez, Angélica Pérez, Emmanuela Daza, Tatiana Romero, Carlos Ávila, Ricardo Álvarez, Camilo Roa, Diana Pérez, Marisol Beltrán, and Lina Ortíz for their valuable help in building the traps, carrying out the samplings, and laboratory work; Mauricio and Jairo Valderrama (Fundación Humedales) for their kind hospitality and assistance during field work; and Sandra Constantino for translating the manuscript.
LITERATURE CITED
1. AGENCIA DE COOPERACIÓN INTERNACIONAL DEL JAPÓN-JICA & CORPORACIÓN AUTÓNOMA REGIONAL DE CUNDINAMARCA COLOMBIA-CAR. 2000. El estudio sobre plan de mejoramiento ambiental regional para la cuenca de la laguna Fúquene. Informe Final. CTI Engineering international co., Ltda., Bogotá. [ Links ]
2. ALVARADO, H. & F. GUTIÉRREZ. 2002. Especies hidrobiológicas continentales introducidas y transplantadas y su distribución en Colombia. Ministerio de Medio Ambiente, Bogotá. [ Links ]
3. ANDERSON, O. 1984. Optimal foraging by largemouth bass in structured environments. Ecology 65(3): 851-861. [ Links ]
4. BAKER, J. & S. T. ROSS. 1981. Spatial and temporal resource utilization by southeastern Cyprinids. Copeia 1981(1): 178-189. [ Links ]
5.CARPENTER, S. R.& K. L.COTTINGHAM. 1997. Resilience and restoration of lakes.Conservation Ecology online1(1):2.http://www.consecol.org/vol1/iss1/art2. [ Links ]
6. CHAPARRO, B. 2003. Reseña de la vegetación en los humedales de la Sabana de Bogotá. Tomo I. Págs. 71-89 en: A. Guarnizo A. & B. Calvachi (eds). Los humedales de Bogotá y la Sabana. Acueducto de Bogotá - Conservación Internacional Colombia, Bogotá. [ Links ]
7. CONSERVACIÓN INTERNACIONAL COLOMBIA-ACUEDUCTO DE BOGOTÁ. 2005. Convenio de investigación aplicada a la restauración ecológica del Humedal Juan Amarillo. Informe final. [ Links ]
8. DAHL, G. 1971. Los peces del norte de Colombia. Ministerio de Agricultura. INDERENA. Talleres Litografía Arco, Bogotá. [ Links ]
9. DIEHL, S. 1993. Effects of habitat structure on resource availability, diet, and growth of benthivorous perch, Perca fluviatilis. Oikos 67(3):413-414. [ Links ]
10. DONATO, J. 2004. Consideraciones generales sobre el estado de los ambientes acuáticos de agua dulce en Colombia. Págs. 226-236 en: J. J. Neiff (ed.). Humedales de Iberoamérica. Programa Iberoamericano de Ciencia y Tecn. para el Desarrollo - Subprograma XVIII, CYTED, La Habana. [ Links ]
11. DONATO, J. 2001. Fitoplancton de los lagos andinos del norte de sudamérica (Colombia). composición y factores de distribución. Academia Colombiana de Ciencias Exactas, Físicas y Naturales. Universitat de Barcelona. Colección Jorge Álvarez Lleras No. 19, Bogotá. [ Links ]
12. DONATO, J. 1998. Los sistemas acuáticos de Colombia: Síntesis y revisión. Págs. 31-47 en: Guerrero, E. (ed.) Una aproximación a los humedales en Colombia. UICN. Fondo FEN, Bogotá. [ Links ]
13. DONATO, J., L. GONZÁLEZ & C. RODRÍGUEZ. 1996. Ecología de dos sistemas acuáticos de páramo. Academia Colombiana de Ciencias Exactas, Físicas y Naturales. Colección Jorge Álvarez. No. 9, Bogotá. [ Links ]
14. EIGENMANN, C.H. 1920. The fish fauna of the Cordillera of Bogotá. Journal of the Washington Academy of Sciences 10:460-468. [ Links ]
15. ESTEVES, F. 1998. Fundamentos de Limnología. Editora Interciencia, Río de Janeiro. [ Links ]
16. FORERO, J. E & M. R. GARZÓN. 1974. Ciclo Biológico de la Guapucha Grundulus bogotensis (Humboldt 1821) (Pisces: Characidae) de la Sabana de Bogotá. Trabajo de grado Biólogo. Universidad Nacional de Colombia, Bogotá. [ Links ]
17. GOLDSBOROUGH, G. & G. ROBINSON. 1996. Pattern in wetlands. Págs. 77-117 en: R. J. Stevenson, M. L. Bothwell & R. L. Lowe (eds.). Algal Ecology. Academic press, INC. San Diego, California. [ Links ]
18. GONZÁLEZ-ACOSTA, J.A. 1992. Caracterización cromosómica en dos especies ícticas nativas, Guapucha Grundulus bogotensis y el capitán de la Sabana Eremophilus mutisii en la Sabana de Bogotá. Trabajo de grado Biólogo. Universidad Nacional de Colombia, Bogotá. [ Links ]
19. GREENBERG, L. A., C. A. PASZKOWSKI & W. TONN. 1995. Effects of prey species composition and habitat on foraging of two functionally distinct piscivores. Oikos 74(3):522-532. [ Links ]
20. JUNK, W. J., M. G. M. SOARES & U. SAINT-PAUL. 1997. The fish. 126: 385-408 en: W. J. Junk (ed.), The Central Amazon Floodplain: Ecology of a Pulsing System. Springer, Ecological Studies, Berlin. [ Links ]
21. LEWIN, W-C., N. OKUN & T. MEHNER. 2004. Determinants of the distribution of juvenile fish in the littoral area of a shallow lake. Freshwater Biology 49: 410-424. [ Links ]
22. LEWIS, W. JR. 1996. Tropical lakes: how latitude makes a difference. Págs. 43-64 en: Schiemer, F. & K. T. Boland (eds.), Perspectives in tropical Limnology. SPB Academic Publishers, Amsterdam. [ Links ]
23. LEWIS, W. JR. 2000. Basis for the protection and management of tropical lakes. Lakes & Reservoirs: Research and Management 5:35-48. [ Links ]
24. LEWIS, W. JR. 2002. Causes for the high frecuency of nitrogen limitation in tropical lakes. Internationale Vereinigung Für Theoretiche Und Angewandte Limnologie 28:210-213. [ Links ]
25. MALDONADO-OCAMPO, J., A. ORTEGA-LARA, J. USMA, G. GALVIS, F. VILLA-NAVARRO, L. VÁSQUEZ, S. PRADA-PEDREROS & C. ARDILA. 2005. Peces de los Andes de Colombia. Guía de Campo. Instituto de Investigaciones de Recursos Biológicos Alexander von Humboldt, Bogotá, D. C. [ Links ]
26. MARGALEF, R. 1983. Limnología. Editorial Omega. Barcelona. [ Links ]
27. MILES, C. 1947. Los peces del Río Magdalena. Bogotá, Colombia, editorial el Gráfico, 214 p. [ Links ]
28. MEERHOFF M., L. RODRÍGUEZ-GALLEGO & N. MAZZEO. 2002. Potencialidades y limitaciones del uso de Eichhornia crassipes (Mart.) Solms en la restauración de lagos hipereutróficos subtropicales. Págs. 61-74 en: A. Fernández & G. Chalar (eds.), Agua en Iberoamérica: de la limnología a la gestión en Sudamérica. CYTED XVII, Buenos Aires. [ Links ]
29. MOJICA, J.I., C. CASTELLANOS, S. USMA & R. ALVAREZ (Eds.) 2002. Libro rojo de peces dulceacuícolas de Colombia. La serie Libros rojos de especies amenazadas de Colombia. Ministerio del Medio Ambiente, Bogotá. [ Links ]
30. PETRY, P., P. B. BAYLEY & D. F. MARKLE. 2003. Relationships between fish assemblages, macrophytes and environmental gradients in the Amazon River floodplain. Journal of Fish Biology 63: 547-579. [ Links ]
31. PINILLA, G. & M. ABRIL. 1996. Crecimiento y desarrollo gonadal del Capitán de la Sabana Eremophilus mutisii, Humbolt 1805, (Pisces: Trichomycteridae) bajo diferentes densidades de siembra en pequeños lagos artificiales. Informe Final Fondo para la Protección del Medio Ambiente José Celestino Mutis FEN Colombia - Fundación Universidad de Bogotá Jorge Tadeo Lozano Centro de Investigaciones Científicas, Bogotá. [ Links ]
32. ROMÁN-VALENCIA, C., R. RUIZ C & R. BARRIGA. 2005. Una nueva especie ecuatoriana del género de peces andinos Grundulus (Characiformes: Characidae). Revista de Biología Tropical 53(3-4): 537-544. [ Links ]
33. ROMÁN-VALENCIA, C., HANS-JOACHIM PAEPKE & F. PANTOJA. 2003 ("2001"). Una especie nueva de Grundulus (Teleostei: Ostariophysi: Characidae) para Colombia y redescripción de Grundulus bogotensis (Humboldt y Valenciennes, 1833). Memorias de la Fundación la Salle de Ciencias Naturales 155: 51-72. [ Links ]
34. SCHINDLER, D. E. & M. D. SCHEUERELL. 2002. Habitat coupling in lake ecosystems. Oikos 98: 177-189. [ Links ]
35. SOARES, M., N.A. MENEZES & W.J. JUNK. 2006. Adaptations of fish species to oxygen depletion in a central Amazonian floodplain lake. Hydrobiologia 568:353-367. [ Links ]
36. SOARES, M. G. M. & W. J. JUNK. 2000. Respiratory adaptations of five curimatid species (Teleostei, Curimatidae) to oxygen depletion in an Amazonian floodplain lake. Internationale Vereinigung Für Theoretiche Und Angewandte Limnologie 27: 1063-1069. [ Links ]
37. TONN, W. M. & J. J. MAGNUSON. 1982. Patterns in the species composition and richness of fish assemblages in northern Wisconsin lakes. Ecology 63(4): 1149-1166. [ Links ]
38. VAN DER HAMMEN, T. 2003. Los humedales de la Sabana: Origen, evolución, degradación, y restauración. Págs. 19-52 en: A. Guarnizo & B. Calvachi (eds). Los humedales de Bogotá y la Sabana. Acueducto de Bogotá - Conservación Internacional Colombia, Bogotá. [ Links ]
39. VYMAZAL, J. 1995. ALGAE AND ELEMENT CYCLING IN WETLAND. Lewis Publishers, Boca Ratón, Florida. [ Links ]
40. WERNER, E. & D. J. HALL. 1988. Ontogenetic Habitat Shifts in Bluegill: The Foraging Rate-Predation Risk Trade-off Ecology 69(5): 1352-1366. [ Links ]
41. WETZEL, R. 1996. Benthic algae and nutrient cycling in lentic freshwater ecosystems. Págs. 641-667 en: R. J. Stevenson, M. L. Bothwell & R. L. Lowe (eds.). Algal Ecology. Academic press, INC. San Diego, California. [ Links ]
42. WETZEL, R. 2001. Limnology: Lake and river ecosystems.3a Edición. Academic press. San Diego. USA. pp. 142-150. [ Links ]
43. WETZEL, R. & G. LIKENS. 2000. Limnological Analyses. Springer-Verlag, New York. [ Links ]
Recibido: 08/02/2007
Aceptado: 14/04/2008