Services on Demand
Journal
Article
Indicators
-
Cited by SciELO
-
Access statistics
Related links
-
Cited by Google
-
Similars in SciELO
-
Similars in Google
Share
Revista de la Academia Colombiana de Ciencias Exactas, Físicas y Naturales
Print version ISSN 0370-3908
Rev. acad. colomb. cienc. exact. fis. nat. vol.40 no.155 Bogotá Apr./June 2016
https://doi.org/10.18257/raccefyn.286
doi: http://dx.doi.org/10.18257/raccefyn.286
Universidad del Valle, Ciudad Universitaria Meléndez, Cali, Colombia. Corresponding author: Jaime R. Cantera Kintz, jaime.cantera@correounivalle.edu.co
Inaugural article by full member of the Colombian Academy of Exact, Physical and Natural Sciences in July 8, 2015
Received: October 07, 2015. Accepted: May 19, 2016
Abstract
The degree of internal Bioerosion of five tertiary sedimentary cliffs was examined in three tidal levels (high, medium and low) located in two bays of the Central region of the Pacific coast of Colombia, Tropical Eastern Pacific. The objective was to estimate bioerosion patterns and understand the principal factors determining spatial and temporal variations. The cliffs are composed by layers of soft sedimentary rocks (limestone) alternated with hard sedimentary rocks (shale, sandstones). Plate tectonics, high seismic risk, and processes occurring in the coastline heavily influence geology and geomorphology of the central region of the Pacific coast of Colombia. The region consists of Quaternary alluvial sediments of Pliocene and tertiary cliffs of sedimentary rocks (sandstones, mudstones and shales) of the Mayorquín and the Naya formations. Due to the high slopes and the high fracturing of the rock, non-biologic erosion is caused primarily by runoff and waves, which produces planar mass movements in different scales. It is also common the occurrence of falling blocks, mainly in the coastal cliffs. Main borers of soft rocks were bivalves of the family Pholadidae: Cyrtopleura crucigera, Pholadidea spp. and the crustacean Upogebia spp. Hard rocks were bored by mytilids species: Lithophaga aristata, L. plumula, and by the sipunculid Phascolosoma sp.
Major factors controlling bioerosion are in order of importance: hardness and nature of rocks, tidal level, abundance and distribution patterns (zonation) of benthic organisms associated to cliff fauna, and wave action. Total internal bioerosion displayed high values in low tidal levels and soft rocks of cliffs with moderate wave action. Bioerosion declines in externally located hard rocks of the two studied bays despite their exposure to higher hydrodynamics. Higher population densities of borer species were recorded in cliffs located in the internal parts of the bays and in the lower tidal levels. Bored volume was significantly different among tidal levels but not among stations. This was positively correlated with number of species and individuals.
Measurements of rocky material loss during the 12-month study period showed that cliff retreat varied between 4.2 cm y-1, in a hard-rock cliff located on an island in the outer region of the Malaga Bay to 13.2 cm y-1 in a mixed-rock cliff of the outer region of the same bay. These rates are high compared to other estimates based on erosion by organisms but are far lower than rates obtained in broad scale studies. Temporal variations would be attributed to local oceanographic conditions, particularly extreme tidal range, strong sea currents and intensity of wave action.
Key words: Benthic fauna, bioerosion, rocky cliff retreat, Tropical Eastern Pacific, zonation.
Resumen
El grado de Bioerosión interna de cinco acantilados sedimentarios terciarios fue estudiado en tres niveles de altura con respecto a la marea (alta, media y baja), ubicados en dos bahías de la región central de la costa del Pacífico de Colombia, en el Pacífico Oriental Tropical. El objetivo de la investigación fue estimar los patrones de bioerosión y comprender los principales factores que determinan las variaciones espaciales y temporales en sus tasas. Los acantilados están compuestos por capas de rocas sedimentarias blandas (limolitas, lodolitas) alternando con rocas sedimentarias duras (esquistos, areniscas). La tectónica de placas, el alto riesgo sísmico, y los procesos de sedimentación y erosión que ocurren en el litoral influyen fuertemente en la geología y la geomorfología de la región central de la costa del Pacífico de Colombia. Esta región está formada por sedimentos aluviales cuaternarios del Plioceno y acantilados terciarios de rocas sedimentarias (lodolitas, areniscas, lutitas y pizarras) de las formaciones Mayorquín y Naya. Debido a las altas pendientes y a la alta fracturación de la roca por movimientos sísmicos, los procesos de erosión son causados principalmente por la escorrentía y las olas que producen movimientos de masas planas con caída de bloques en diferentes escalas. Los principales perforadores de rocas blandas fueron bivalvos de la familia Pholadidae: Cyrtopleura crucigera, Pholadidea spp. y los crustáceos Upogebia spp. Las rocas duras son perforadas por especies de mitílidos: Lithophaga aristata, L. plumula y por el sipuncúlidoPhascolosoma sp.
Los principales factores que determinan los patrones de bioerosión en orden de importancia son, la dureza y naturaleza de las rocas, el nivel de las mareas, la abundancia y los patrones de distribución (zonificación) de organismos, y la acción de las olas. Las tasas de bioerosión interna muestran valores altos en los niveles bajos de marea y en las rocas blandas de acantilados con una acción moderada del oleaje. La bioerosión es menor en las rocas duras situadas en la parte externa de las dos bahías, a pesar de la mayor exposición a las olas. Las densidades de población más altas de las especies de perforadores se registraron en los acantilados ubicados en las partes internas de las bahías y en los niveles de las mareas más bajas. El volumen removido fue significativamente diferente entre los niveles de marea, pero no entre las estaciones, estando correlacionado positivamente con el número de especies y de individuos. Las mediciones de pérdida de material rocoso durante el período de estudio de 12 meses mostraron que el retroceso del acantilado varió entre 4,2 cm año-1, en un acantilado de roca dura situada en una isla en la región exterior de la bahía de Málaga, a 13,2 cm año-1 en un acantilado de rocas mixtas de la región externa de la misma bahía. Estas tasas son altas en comparación con otras estimaciones sobre erosión por organismos, pero son mucho más bajas que las obtenidas en estudios de amplia escala. Las variaciones temporales se pueden atribuir a las condiciones oceanográficas locales, a la amplitud de la marea, a fuertes corrientes marinas y la intensidad de la acción de las olas.
Palabras clave: fauna bentónica, bioerosión, regresión de acantilados rocosos, Pacífico Oriental Tropical.
Introduction
The shape and landscape of coastlines are products of geological history, dominated by erosion processes in cliff areas (Naylor, et al., 2012) and sedimentation processes in depositional areas (sand beaches and mudflats areas) (Naylor, et al., 2010). Several authors believe physical factors (erosion by waves and weathering) play the main role in the destruction of rocky cliffs (Russell, 1962; Stephenson and Kirk, 2000a, 2000b; Trenhaile, 2002), particularly when they are located in areas with high hydrodynamics. This explanation has been based in the relationship between rock hardness and resistance, and the hydraulic action caused by the magnitude of wave energy (impact on the rock) and mechanical action of weathering. Others attribute the main action to hydrochemical processes (Kaye, 1959). The action of borers should not be disregarded for it accelerates physical erosion of rocky coastlines, by destroying sea-front cliffs, changing bay sizes and limits, and transforming old peninsulas into islands (Warme, 1970; Fischer, 1981a; Cantera, et al., 1998; Naylor, et al., 2012). On the shores near the cliffs, this process induces the formation of new sedimentary substrates (Cruz-Colin and Curul-Magaña, 1997) and may be responsible for the appearance of rocks on sandy beaches, mud flats and soft bottoms. Bioerosion may also result in structural changes in biological communities such as rock dweller loss or recruitment and changes in the proportion of various groups of organisms (Cantera, 1991).
The role of organisms in erosion processes has been studied extensively in some areas of the world. Bioerosion of rocky and sedimentary cliffs has been known since the beginning of the XXth century as an important agent of destruction of rocky coasts and one of the main processes that contributes to modify coastal geomorphology (Jehu, 1918; Lamy, 1921). Several papers have shown the role of different types of organisms in rock bioerosion. Microorganisms can cause important initial bioerosion (Viles, 2012; Schneider and Lecampion-Alsumard, 1999). Intertidal rocks are bored by grazing gastropods and echinoids (Kaye, 1959; MacLean, 1972). Erosion by sponges has been studied in calcareous substrates (Neumann, 1966) and there are many papers dealing with erosion on coral skeletons. Some papers deal with boring bivalve species (Kleemann, 1973; Cantera and Contreras, 1988; Cantera, et al., 1998); others cite crusta-ceans (Thiestle, 1973; Williams, 1986; Holthius, 1980) and Sipunculans (Rice, 1969). Comprehensive studies have been published by Hodgkin (1970), Schneider (1976), Torunski (1979), Fischer (1981a, b), Trenhaile (1987), Sunamura (1994), Radtke, et al. (1997), Naylor and Viles (2002), Spencer and Viles (2002), Naylor, et al. (2002, 2010 and 2012). Sunamura (1994) has developed a conceptual model of rock coast erosion, but this author underestimates the role of biological agents and gives more importance to the relation force of wave/resistance of rocks. As was demonstrated by Naylor, et al. (2012) most of geomorphologic processes are affected by organism, resulting in a new conceptual model that includes biological agents as important reducers of the resisting force of the rock.
Most authors point out that rock bioerosion is controlled by factors such as type of rocks (geological composition and hardness), wave intensity, tidal exposure, speed of currents (physical), cliff biotic community structure, abundance of grazing organisms, density of boring species and mechanisms of boring (biological). Bioerosion rates have been measured by Robinson (1977), Torunski (1979), Fischer (1981b), and Cantera, et al. (1998). Historically, most literature emphasizes on coral bioerosion and pays less attention to the destruction of rocky cliffs by boring organisms.
In the Pacific coast of Colombia, rock bioerosion has been described as an important cause of geomorphological instability of the coastline, frequently affecting areas occupied by human settlements. The appearance of several "arches" or "lines" of islands in different parts of the Colombian Pacific coast has been attributed to coastal erosion with important participation of organisms (Martinez, 1993). These organisms and their patterns of abundance and distribution have been only recently studied and mainly in a descriptive or preliminary way (Ricaurte, 1995; Cantera, et al. 1998). Therefore, quantitative data on rates and patterns of rock destruction, their spatial and temporal variability and the environmental factors affecting them are scarce.
The main goals of this study were: (1) to estimate cliff wall loss (retreat) in 5 cliffs exposed to different environmental conditions in the central region of the Pacific coast of Colombia; (2) to compare them with relation to three tidal levels and to cliff rock composition; and (3) to determine the density and distribution of main boring species and their role in the loss of cliff rocky material.
Materials and Methods
Study area
The central zone of the Pacific Colombian coast has a high tectonic activity as result of the convergence of the Suramerican, Nazca and Caribe plates. The geomorphology of this area is diverse and the coastline presents Tertiary consolidated sedimentary rocks forming cliffs and shores, bordered by Quaternary mobile sediment platforms (Martinez, 1993; Cantera and Blanco, 2001). This coast has been highly influenced by historic high tectonic activity depending of movements and shocks (clashes) of South American, Nazca and Caribbean Plates and consequents frequent seismic movements. A smaller scale, geomorphology of the coast of the central region of Pacific coast of Colombia has been changed by subsidence, erosion and sedimentation processes. At present, the coast consists of consolidated Quaternary alluvial sediments and Tertiary cliffs of sedimentary rocks (mudstones, sandstones, and shales) of the Mayorquín and the Naya formations (Cardona, et al, 2015). Due to the high slopes and the high fracturing of the rock, erosion processes are caused primarily by seismic movements, runoff and waves, which produces planar mass movements and fall of the rocks in different scales leaving blocks on the feet of cliffs. Structurally, there are different sedimentary stratified layers with evidence of displacement (Malaga bay) and without these evidences (Buenaventura bay) and abundant fractures along the cliff (Montoya, 2003).
The dominant rocks are soft stones such as dark grey mudstones and siltstones, alternating with sandstones and conglomerates (Galvis and Mojica, 1993). The layers of soft rocks are well defined in stratified and homogeneous lawyers and are mainly composed of grey limestone deeply weathered, burrowed and with a wrinkled surface. Hard rocks are consolidated sandstones and shale dark and irregular.
The region is located within the Inter-Tropical Convergence Zone (ITCZ). This low-pressure belt and the orographic rain caused by the presence of the Western Chain of the Cordillera, influence the climate of the Pacific coast of Colombia. This area one of the most humid lowlands of the world, with a precipitation average of 6,500 mm y-1, 228- 298 days of precipitation by year, and a relative humidity of 80-95 % (Lobo-Guerrero, 1993).
Experimental design
1. Selection of stations
Five stations with rocky cliffs were selected in the estuarine bays of Buenaventura (3° 48' - 3° 54' N and 77° 05' - 77°20' W) and Malaga (3° 56' - 4° 05' N and 77° 19' - 77° 21' W) (Figure 1). Both bays present two rainy periods, with precipitations exceeding 567 mm mo-1, interrupted by low precipitation periods with 374 mm mo-1 (Eslava, 1993). As a result of high precipitations there are many freshwater sources and both bays present estuarine salinities varying depending of tides (Malaga: ranging from 20 to 33 (salinities in psu) at the mouth to 10-15 in the inner part; Buenaventura: ranging from 15 to 25 at the mouth to 4-5 in the inner part). Both bays have wide range (macrotidal), semidiurnal tides (3.5 to 4.2 m) generating strong surface currents, and variable action of waves on the cliffs, ranging from "exposed" in outer cliffs to "sheltered" in inner ones.
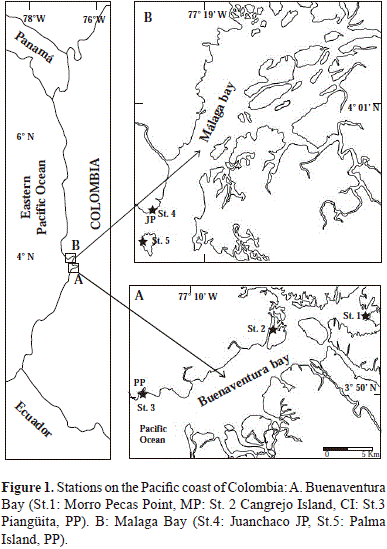
Selection of stations (Table 1) was made to obtain a representative diversity in rock composition and hardness, exposure to waves and tidal action, salinity of surrounding waters, cliff slope, and geographical location. Three stations were selected in Buenaventura Bay, two (St. 1 and 2) in inner parts of the bay (shelter cliffs) and one (St. 3) in outer part (mid-exposed) and two in Malaga Bay (St. 4 and 5) both in exposed areas. The order in hardness of the rocks (St. 1 to St. 5) was the same that in cliff exposition to waves.
2. Field and laboratory techniques
Different studies on bioerosion processes in Tertiary rocky cliffs of Malaga and Buenaventura Bays were conducted by two years in order to estimate densities of macroborers and patterns of loss of rocky material.
2.1. Sediment grain size distribution
We cut 5 cm cubic fragments of soft rocks at the five stations, disaggregated them with a press in laboratory, weighed the product and sieved it through meshes of 2, 1, 0.5, 0.250, 0.100, 0.063 and 0.050 mm. The fractions retained on each sieve were weighed to the closest milligram and these weights were expressed as percentage (%) of total weight. Grain size distribution was analyzed including determination of silt, clay, sand and their relative proportion (Mahieu, 1984).
2.2. Density of perforations and boring species
For each tidal level we selected three 0.0625 m2 quadrats and counted the perforations appearing in them in two different manners. First, we introduced a stick inside each bore, and then counted the sticks. Secondly, we took a picture, counted the perforations and determined their total area using a program for digital image processing. For the quantification of boring species, we took an X-ray picture which was used to determine the species associated to a given type of cavity. A sample was then collected from each quadrat and crushed using a press. Boring macrofauna was extracted and all individuals of each species were counted. Volume was calculated multiplying estimated area by the length of the perforation.
The percentage of perforation was determined using X-ray picture and digital image processing of the rock section under study. For each sample, the % of erosion was then calculated by dividing the volume of perforations by the total volume of the sample.
2.3. Determination of Bioerosion patterns
In order to estimate cliff material loss by the combined action of organisms and physical conditions, a 0.80 m (mm-graded) iron bar was stuck into the rocky wall of the five studied cliffs, trying to avoid loss of surface material. Wall retreat was determined every month during 12 months by measuring the exposed portion of the iron bar. Where it was not possible to introduce the whole iron bar, material loss was measured as from the initial surface mark. Three iron bar replicates were used for each of the three tidal levels.
3. Data analysis
3.1. Sediment grain-size distribution
Collected data allowed studying rock composition and hardness, and relationship between borers' density and bioerosion rate. Similarities between samples were analyzed using different similarity indices and aggregation methods for cluster analysis, calculated by STATISTICA© software package. Since similar results came out of all methods, percent disagreement was chosen as distance index, and unweighted pair-group average as cluster procedure.
3.2. Density of perforations and boring species
Data on perforation density, abundance of individuals and number of boring species were tested for normality using the probability plot procedure, and for homogeneity of variances using the Levene's and Bartlett's procedures. Results indicated that data on perforation density did not require transformation. For number of species and individuals, an Ln transformation was necessary. A two-way ANOVA test was performed to check for differences between stations and tidal levels. Post ANOVA Newman-Keuls test was also performed to check for differences between stations.
3.3. Determination of Bioerosion rate
The a priori test revealed that the variable bored volume (in percentage), required transformation. After several attempts, this variable failed in the a priori tests and successive transformations. Therefore, the non-parametric Kruskal-Wallis ANOVA test was used to compare stations and zones of the cliff, using STATISTICA© software package. Relationships between bored volume and the main parameters were established by regression analysis.
Data obtained for cliff retreat with relation to time did not require transformation and were compared using two-way ANOVA and analyzed both for the instantaneous retreat rate per month and for the accumulated loss of material throughout successive months.
Results
Sediment grain size in soft cliff rocks
Grain size distribution of soft-rock sediments from the five cliffs showed that the two rock samples from the inner Buenaventura Bay (St. 1 and St. 2) were quite different from those of the outer Malaga Bay (Figure 2). All cliffs showed high percentages of 0.100 mm particles. Cluster analysis showed that there was no significant variation within the same cliff, except for St. 3, in which lower and medium zones were similar to hard-rock cliffs (St. 4 and St. 5) and the high zone was closer to soft-rock cliffs (St. 1 and St. 2).
Abundance and density of perforations and boring species
The number of perforations was significantly different among stations (F= 11.40, p< 0.001) as well as between tidal levels (F=25.97, p< 0.001). The interaction between stations and tidal levels was not significant (F= 1.88, p< 0.103). Newman-Keuls post ANOVA test showed differences between St. 2 and all other locations. St. 2 had significantly more perforations than the rest (Figure 3A).
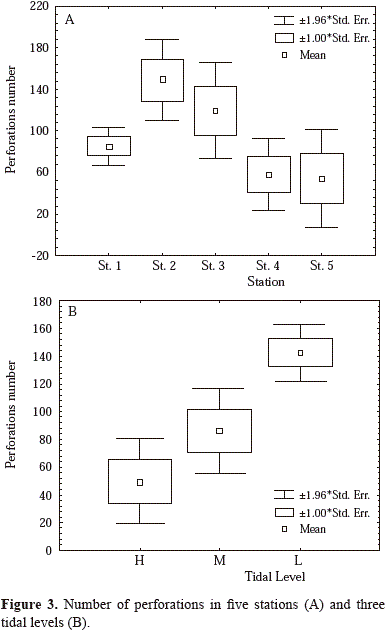
In all cliffs, the lowest tidal level (L) presented the highest number of perforations (Figure 3B) except in St. 2, where there was no significant difference between densities of perforations at high and low tidal levels. In general, there are more perforations in the predominantly soft-rocks cliffs of inner wind-protected stations (St. 1 and St. 2) which extend into the whole intertidal surface of the cliff. Perforations density is higher in the lower parts of the three cliffs exposed to more intense hydrodynamic action.
There is a negative relationship between density of perforaÂtions and height on the cliff (Figure 4). However, the relationÂship is not statistically significant (perforations=121.38-32.68 * height, r= -0.297, n=43, F=3.97, p=0.053).
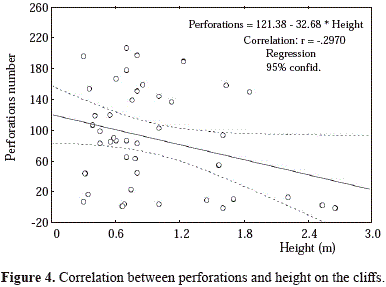
Spatial variations in erosion rates
Stations 3 and 4 presented the highest number of boring species and St. 5 the lowest (Figure 5A). Number of individuals was not significantly different between stations (F=4.19, p= 006) but was lower in both cliffs of the outer Malaga Bay (Figure 5B). Also, perforations volume was not significantly different between stations (H4,45 = 13.26, P= 0.010), the highest volume of St. 2 (Figure 5C) being similar to those of the other two Buenaventura Bay cliffs but higher than St. 4 and St. 5.
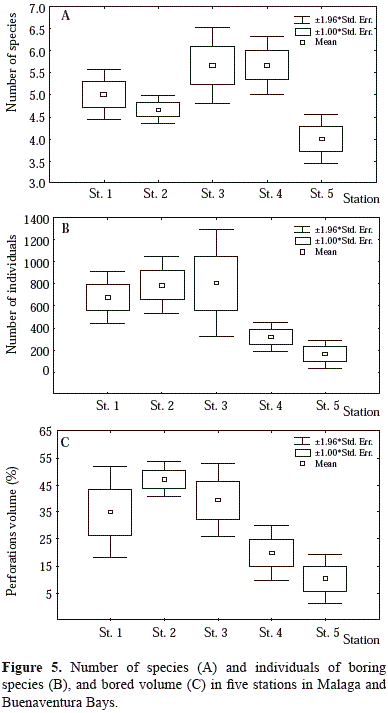
in boring species number, in individuals and in percentage of bored volume (Figure 6). In Stations 1 and 2, the high tidal level exhibits a greater bored volume compared with other stations, with a uniform rock loss in its three tidal levels (H, M and L). St. 5 showed the lowest percentage of bored rock, coinciding with rock hardness and low density of perforations despite the stronger hydrodynamic action.
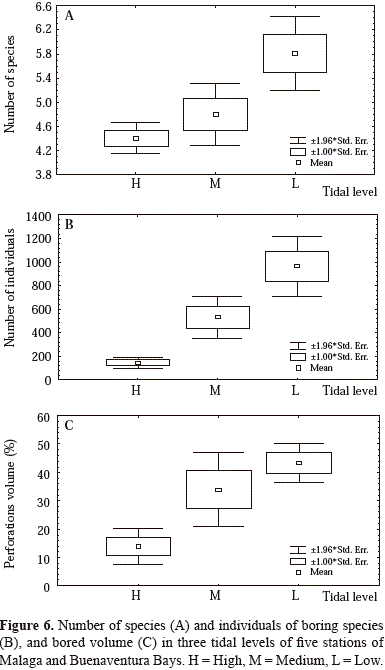
Species and number of individuals are significantly and positively correlated (higher for number of individuals) with bored volume (Figure 7A and 7B). In contrast, bored volume is negatively correlated with the height of the sample (Figure 7C). There is also a significant positive correlation between bored volume and perforation density. The species with the highest percentage of bored volume in the lower zone were Lithophaga aristata (St. 5: 45.2+1.7, St. 3: 35.2+2.4, St. 4: 33.1+2.6 %; %; St. 2: 21.6+5.3 %, St. 1: 15.8+3.8 %) and Upogebia spp. (St. 1: 62.3+3.6 %, St. 3: 32.8+2.8 %; St. 5: 21.3+1.7 %; St. 2: 48.2+6.9 %, St. 4: 17.2+1.2 %). In the medium zone, Cyrtopleura crucigera (St. 2: 72.8+27.2 %; St. 1: 85.7+31.8 %, St. 3: 76.7+12.5 %) is the species with highest percentage of bored volume. The remaining species are responsible for a small proportion of bored volume.
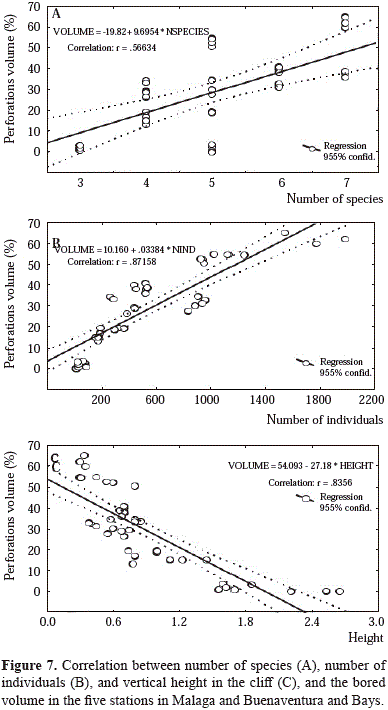
Temporal changes in average cliff retreat rate
The rate of cliff retreat varied significantly not only throughout the study (F= 12.78, p< 0.001) but also among stations (F= 9.52, p< 0.001). Interaction between months and stations was also significant (F= 2.48, p< 0.001). Newman- Keuls Post Hoc test showed significant differences between St. 5 and all the other stations. The analysis of retreat rate with relation to tidal levels showed no significant differences (F= 0.622, p= 0.538). Absolute retreat rate was very variable throughout the 12 months of the study (Figure 8). The three outer stations of the bays (St. 4, St. 5 and St. 3) had the highest rates during the first part of the study, reaching a maximum in September (St. 3: 4.5 cm; St. 4: 6.3 cm; St. 5: 4.2 cm) and strongly decreasing afterwards, particularly St. 4 and St. 5. St. 3 presented irregular variations. The inner cliffs of the Buenaventura Bay had their highest retreat in October (St. 1: 4.2 cm; St. 2: 5.8 cm) with a secondary peak in January.
Cumulative values of cliff retreat at the end of the study period were very different among localities, varying between 4.2 cm y-1 (St. 5) and 13.2 cm y-1 (St. 4). Other values were St. 1: 9.7 cm y-1; St. 2: 10.5 cm y-1; and St. 3: 10.8 cm y-1 (Figure 9).
Discussion
A positive relationship was observed between number of perforations, and cliff surface retreat with lithology, species richness, macrofauna zonation and density of boring species.
A negative correlation was observed with rock hardness and tidal level. Interaction between nature of substrate, tidal level and wave exposure were the main factors controlling bioerosion in the studied cliffs. As expected, stations with high proportion of soft rocks and fine sediments located in the lower zones of the most exposed cliffs showed the highest cliff retreat. Hard rock cliffs located at very exposed areas exhibited a small number of perforations and a low cliff retreat rate. Bored volume and number of perforations were higher in sheltered soft muddy cliffs, although cliff retreat was intermediate. Furthermore, there is a relationship between observed cliff retreat and the time-period of the study. Accordingly, we can reject the two null hypotheses that there are no significant spatial differences and temporal changes in number of perforations, bored volume and cliff retreat rate among the three selected tidal levels of the five studied cliffs in Málaga and Buenaventura Bays.
Cluster analysis separates the inner Buenaventura Bay rocks (softer, alluvium-rich and less basaltic and magmatic) from those of Málaga Bay (harder and mainly volcanic). The main characteristics of rocks which favor the bioerosion process are: chemical composition, texture, lithology, stratification and slabbing (Fisher, 1981a; Cruz-Colin and Curul- Magaña, 1997). Colombian Pacific sedimentary rocks, rich in silicified limestone and basaltic components (Martinez, 1993; Galvis and Mojica, 1993) facilitate colonization by Lithophaga. Conversely, their colonization by sponges and pholadid bivalves is limited by their low calcareous contents, and by sipunculans and polychaetes, due to their high basalt content (Fischer, 1981b). High percentages of fine sediments (less than 0.3 mm) in most cliff rocks facilitate the action by Lithophaga and pholadid bivalves, whereas the sediments between 0.3 and 0.8 mm found in higher proportions at PP, JP and PI favor crustaceans and sipunculans. Coarser fractions found mainly at PI limit all types of internal borers and are only subject to external abrasion by sea urchins. Except for some occasional individuals of Hespedocidaris asteriscus in low zones of PI, no sea urchins were observed in the studied cliffs.
Slab orientation inhibits deep boring by Lithophaga (in all cliffs) and Upogebia spp. (in MP and CI cliffs) but pholadids (Cyrtopleura crucigera) at MP, CI and PP are able to change the orientation of their boring to follow the main stratification axis.
Density of boring organisms was positively correlated with bored volume. Lithophaga species (Lithophaga aristata, L. plumula, L. spatiosa and L. hancocki) present in all stations in medium and low levels are particularly abundant in JP and PP, where there is volcanic material mixed with mudstones. Average Lithophaga density varies between 3.15 Ind m-2 (MP medium tidal level) and 93.2 Ind m-2 (JP low tidal level). These figures are high compared to other cliffs of the world. At the Pacific coast of Costa Rica Lithophaga is restricted to calcareous substrata (Fischer, 1981a), and in the northern Adriatic to the subtidal zone of rocky cliffs where its bioerosion is insignificant compared to endolithic micro-organisms and grazers (Torunski, 1979). Lithophaga plays an important role in hard rocks, where petricolid bivalves (Petricola paralela and P. denticulata) are present, but less abundant (densities between 0.08 Ind/m-2 in JP's medium tidal level and 0.83 Ind m-2 in JP's low tidal level). Instead, Pholadidae (Cyrtopleura crucigera, Pholas chiloensis, Pholadidea spp. and Diplothyra curta) are abundant in the medium tidal level of MP, CI and PP. Sipunculans (Phascolosoma sp.) are evenly distributed in all stations with about one Ind m-2 in the low tidal level. The boring crustacean Upogebia spp. is also present in all stations and is also more abundant in the low tidal level (11 to 53.8 Ind m-2). Fischer (1981b) recorded Alpheus saxidomus as the most important decapod in the bioerosion process of the Pacific coast of Costa Rica.
Temporal changes observed in this research could be attributed to local oceanographic conditions. In the coastal erosion literature, wave action and tidal range and action are considered the two oceanographic agents that cause retreat of rocky cliffs (Schneider, 1976; Torunski, 1979; Fischer, 1981b). Both factors varied during the study period as follows: between January 1997 and April 1998, eleven very high tides were recorded at the Pacific coast of Colombia, the highest corresponding to February 1997 and March 1998. Winds and wave action were stronger between August and November. We observed that the combination of very high tides ("extreme spring tides") and strong waves favor the deposition of sand at the bottom of the cliffs in St. 3, 4 and 5, thus changing the accessibility of these sections of the cliff due to the action of the sea. Accumulated sand covered cavities, killing established borers while preventing hydrodynamic action and colonization by new borers.
Few published data on cliff retreat as a consequence of bioerosion are available for comparison. Previous studies are of very different nature and mainly refer to: (1) measurements related to superficial abrasion by gastropods (in their home scars), by sea urchins and by sponges; (2) different types of rocky material in cliffs or in rocky shores with little slope; (3) different borers' species or taxonomic groups: and (4) measurements made directly on cliffs, but through different methods regardless of the presence of organisms.
Studies related to abrasion by mollusks in home scars showed variable bioerosion rates: Littorina spp. in calcareous sandstone from Southern California (USA), 0.07 mm y-1 (Emery, 1946) and 0.6 mm y-1 (North, 1955). Nerita tesselata, in beachrock of reef areas in the Caribbean, 0.1 mm chitons in the same places 8-13 mm y-1 (MacLean, 1972). Superficial erosion by sea urchins has been studied by Fischer (1981b) estimating a boring rate for Diadema mexicana of 0.005-0.16 mm y-1 in basaltic cliffs of the Pacific coast of Costa Rica. Finally, Torunski (1979) reported a bioerosion rate of 0.02-0.98 mm y-1 by sea urchins, and of 0.10-1.11 mm y-1 caused by the benthic community at the Gulf of Trieste (Adriatic Sea). Sponges were studied by Neumann (1966) who determined boring rates of 1.4 cm y-1 for Cliona lampa, in laboratory controlled experiments with submerged carbonate substrate from Bermuda (USA).
In geological studies using aerial photography and scales at the basis of cliffs, Andrade, et al., (2002) estimated a cliff retreat of 0.14-8.2 cm y-1 for the west limestone coast of Portugal. Dias and Neal (1992) recorded retreats of 1 to 2 m y-1 (with a maximum value of 6.3 m) in southern Portugal Miocene-Quaternary friable cliffs resulting from joint environmental and anthropogenic factors. With aerial photography, Cruz-Colin and Curul-Magaña(1997) estimated a retreat rate of 0.8-0.97 m y-1 for sandstone and shale cliffs and of 0.7 m y-1 for basaltic cliff in Baja California (Mexico). None of these studies is any reference to the boring species participating in the process.
Specific data on bioerosion rates and retreat in cliffs with abundance of bivalves are scarce, outdated and not appropriate for comparisons. Limestone cliffs of the Pacific coast of USA were studied by Evans (1968), who estimated a bioerosion rate of 1.2 cm y-1 caused by Penitella penita (Pholadidae). Pioneer studies (Jehu, 1918) reported a bioerosion rate of 2.5 and 1.3 cm y-1 respectively for Lithophaga, in chalky subtidal benches of the coast of Norfolk in England. Kleemann (1973) reported a bioerosion rate of 0.016-0.16 mm y-1 also by Lithophaga sp. Finally, in the Gulf of Oman Vita-Finzi and Cornelius (1973) reported a bioerosion rate of 0.25-0.9 cm y-1 derived from the length of the cavities of Lithophaga obesa and L. cumingiana.
Despite the considerable variability in data, methods, species and material, we can hypothesize that our average rate of 4.2 cm y-1 and maximum rate of 13.2 cm y-1 are indicative of a bioerosion process, which is more intense in the Colombian Pacific tertiary cliffs than in most other cliffs. However it is significantly less intense than that of the basaltic (0.70 m y-1) and sandstone and shale (0.8-0.97 m y-1) cliffs of Baja California and of the Miocene-Quaternary friable cliffs of southern Portugal (1 to 2 m y-1).
In conclusion, the complete process of rocky cliff destruction involves four main phases that interact with each other and the participation of different organisms, chemical corrosion and physical factors: (1) Micro-algae generating micro cavities, (2) Bio-abrasion by gastropods and crustaceans while feeding upon endolithic micro-algae. (3) Perforations by bivalves, sipunculans and crustaceans weaken the structure. (4) The mechanic action of breaking waves forms an abrasion cavity penetrating the lower part of the cliffs and the protruding upper cliff wall would then collapse, thus completing the falling of the rock blocks. This process is facilitated by vertical, subvertical and horizontal geologic fractures in the cliffs that cause the falling of the rock blocks acting combined or independently of the impact of material removed by the organisms.
Mudstones would continue their destruction on the sandy or muddy surface and the resulting tiny sediment particles would be washed away by currents. This process will contribute to the formation of mud planes in the inner side of bays or else to the muddy enrichment of sandy beaches. The coarser or thicker sedimentary material, such as sandstones, conglomerates and stone slabs will form intertidal or subtidal rocky substrates or hard rocky substrates in edge mangroves.
The whole process has also a major impact on the ecological structure of the biological communities associated to the Tertiary cliffs in the Colombian Pacific coast. Their populations present quantitative variations resulting from availability of new substrates for colonization, because of material loss. Specific cliff richness, which is close to zero immediately after the collapse of rocks, increases gradually as the colonization progresses and a new process of abrasion, boring and loss of material, begins. In the surrounding mudflats and sandy beaches diversity increases thanks to the species that colonize the new rocky substrates of various size disposed on the surface.
Acknowledgments
This study was conducted thanks to different research projects supported and funded by the Department of Biology of the Universidad del Valle, Biology Department, Marine Biology Section, and partially by internal funding of the Research Vice-rectory of the Universidad del Valle (CI 7980) "Biological erosion in cliffs of the Central part of the Colombian Pacific". The author thanks Henry von Prahl, Raul Neira O., Constanza Ricaurte and Alba Marina Cobo-Viveros, who have collaborated in some parts along the research on bioerosion. Likewise, the author thanks Dr. Iván Correa (Department of Environmental Geology of EAFIT University), Daniela Cardona, Alejandra Diaz and Camila León for providing information and advice in geological aspects.
Conflict of interests
The authors declare no conflicts of interest of any kind.
Bibliography
Andrade C., Marques F., Freitas M.C., Cardoso R. & Madeureira P. (2002) Shore platform downwearing and cliff retreat in the Portuguese west coast. In: EUROCOAST Littoral 2002, the changing coast, Eurocoast / EUCC, Portugal, 423-431. [ Links ]
Cantera J.R. (1991) Etude structurale des mangroves et des peuplements littoraux des deux baies du Pacifique colombien (Málaga et Buenaventura). Rapport avec les conditions du milieu et les perturbations anthropiques. Thèsed'Etat Sciences. Universitéd'Aix-Marseille II. Marseille, France, 429 p. [ Links ]
Cantera J.R. & Blanco J.F. (2001) The estuary ecosystem of Buenaventura bay, Colombia. In: Seeliger, U., Kjerve, B. (Eds.). Coastal marine ecosystems of Latin America. Ecological Studies. 144: 265-280 [ Links ]
Cantera J.R. & Contreras R. (1988) Bivalvos perforadores de corales escleractiniarios en la Isla de Gorgona, Colombia. Revista Biología Tropical. 36: 151-158. [ Links ]
Cantera J.R., Neira R. & Ricaurte C. (1998) Bioerosión en acantilados del Pacifico colombiano. Santa fe de Bogotá, Fondo FEN, 160 p. [ Links ]
Cardona D., Díaz A., León M.C. (2015). Bioerosion en acantilados de la costa central del Pacifico colombiano. Informe Geología, Universidad Eafit, 23 p. [ Links ]
Cruz-Colin M.E. & Curul-Magaña L.A. (1997) Erosion and sediment supply of sea cliffs of Todos Santos, Baja California, from 1970 to 1991. Ciencias Marinas. 23: 303-315. [ Links ]
Dias J.M. & Neal W.J. (1992) Sea cliff retreat in southern Portugal: profiles, processes and problems. Journal of Coastal Research. 8: 641-654. [ Links ]
Emery K.O. (1946) Marine solution basins. Journal of Geology. 54: 209-228. [ Links ]
Eslava J. (1993) Climatología. In: Leyva, P. (Ed.) Colombia Pacífico. Santafé de Bogotá, Fondo FEN-Proyecto Biopacífico, Tomo 1: 137-147. [ Links ]
Evans J.W. (1968) The role of Penitella penita (Conrad, 1837) (Family Pholadidae) as eroders along the Pacific coast of North America. Ecology. 49: 156-159. [ Links ]
Fischer R. (1981a). La bioerosion de la costa pacífica de Costa Rica. Anais II Congresso Latinoamericano Paleontología, Porto Alegre, 907-918. [ Links ]
Fisher R. (1981b) Bioerosion of basalt of the Pacific coast of Costa Rica. Seckenbergiana maritima. 13: 1-41. [ Links ]
Galvis J. & Mojica J. (1993) Geología. In: Leyva, P. (Ed.) Colombia Pacífico. Santafé de Bogotá, Fondo FEN-Proyecto Biopacífico. Tomo I: 80-96. [ Links ]
Hodgkin E.P. (1970) Geomorphology and biological erosion of limestone coast in Malaysia. Geological Society Malaysia Bulletin. 3: 27-51. [ Links ]
Holthuis L.B. (1980) Alpheus saxidomus new species; a rock boring snapping shrimp from the Pacific coast of Costa Rica, with notes on Alpheus simus Guérin-Méneville, 1856. Zoologische Mededelingen. 55: 47-58. [ Links ]
Jehu T.J. (1918) Rock-Boring organisms as agents in coastal erosion. Scottish Geographical Magazine. 34: 1-10. [ Links ]
Kaye C.A. (1959). Shore line features and Quaternary shoreline changes, Puerto Rico. U.S. Geological Survey professional paper. 317-B, 140 p. [ Links ]
Kleemann K.H. (1973) Lithophaga lithophaga (L) (Bivalvia) in different limestone. Malacologia. 14: 345-347. [ Links ]
Lamy E. (1921) Les théoriesexplicatives de la perforation par les mollusques lithophages et xylophages. Revue scientifique. 59: 423-432. [ Links ]
Lobo-Guerrero A. (1993) Hidrología e hidrogeología. In: Leyva, P. (Ed.) Colombia Pacífico. Santafé de Bogotá, Fondo FEN-Proyecto Biopacífico. Tomo 1: 121-134. [ Links ]
MacLean R.F. (1972) Nomenclature for rock destroying organisms. Nature. 240: 490. [ Links ]
Mahieu G. (1984) Milieu et peuplements macrobenthiques littoraux du golfo Triste (Venezuela). Etudes expérimentalessur sa pollution. ThèseDoctorat Sciences Université Aix- Marseille 2, Marseille, France, 333 p. [ Links ]
Martínez J.O. (1993) Geomorfología. In: Leyva, P. (Ed): Colombia: Pacifico. Santafé de Bogotá, Fondo FEN-Proyecto Biopacífico. Tomo 1: 111-119. [ Links ]
Montoya, D.M. (2003). Geología de las planchas 240 Pichimá, 241 Cucurrupí, 259 Malaguita y 260 Aguas Claras. Ingeominas, Bogotá [ Links ].
Naylor L.A. & Viles H.A. (2002) A new technique for evaluating short-term rates of coastal bioerosion and bioprotection, Geomorphology. 47 (1): 31-44, http://dx.doi.org/10.1016/S0169-555X(02)00139-3. No está citado.
Naylor, L.A., Viles, H.A. and Carter, N.E.A., 2002. Bio-geomorphology revisited: Looking towards the future. Geomorphology. 47: 3-14. [ Links ]
Naylor L.A., Stephenson W.J. and Trenhaile A.S. (2010) Rock coast geomorphology: recent advances and future research directions, Geomorphology. 114 (1-2): 3-11, http://dx.doi.org/10.1016/j.geomorph.2009.02.004.
Naylor L.A. Coombes M.A. and Viles H.A. (2012) Reconceptualising the role of organisms in the erosion of rock coasts: a new model, Geomorphology. 157-158: 17-30, http://dx.doi.org/10.1016/j.
Neumann C. (1966) Observations on coastal erosion in Bermuda and measurements of the boring rates of the sponge Cliona. Limnology and Oceanography. 11: 92-108. [ Links ]
Radtke G., Hofmann K. & Golubic S. (1997) A bibliographic overview of micro- and macroscopic bioerosion. Courier Forschungsinstitut Senckenberg. 201: 307-340. [ Links ]
Ricaurte C. (1995) Bioerosión en las bahías de Málaga y Buenaventura, costa Pacífica colombiana. Tesis de grado. Universidad del Valle, Departamento de Biología, 85 p. [ Links ]
Rice M.E. (1969) Possible boring structures of Sipunculids. American Zoologist. 9: 803-812. [ Links ]
Robinson L.A. (1977) Erosive processes on the shore platform of northeast Yorkshire, England. Marine Geology. 23: 339- 361. [ Links ]
Russell R.J. (1962) Origin of beach rock. Zeitschrift fürGeomorphologie. 6: 1-16. [ Links ]
Schneider J. (1976) Biological and inorganic factors in the destruction of limestone coasts. Contributions to Sedi-mentology. 6: 112. [ Links ]
Schneider J. & Le Campion-Alsumard T. (1999) Construction and destruction of carbonates by marine and freshwater cyanobacteria. European Journal of Phycology. 34: 417-426. [ Links ]
Spencer T. & Viles H. (2002) Bioconstruction, bioerosion and disturbance on tropical coasts: coral reefs and rocky limestone shores, Geomorphology. 48 (1-3): 23-50, http://dx.doi.org/10.1016/S0169-555X(02)00174-5.
Stephenson W.J. and Kirk R.M. (2000a) Development of Shore Platforms on Kaikoura Peninsula, South Island, New Zealand, Part I The Role of Waves. Geomorphology. 32: 21-41. [ Links ]
Stephenson W.J. and Kirk R.M. (2000b) Development of Shore Platforms on Kaikoura Peninsula, South Island, New Zealand, Part II The Role of Subaerial Weathering. Geomorphology. 32: 43-56. [ Links ]
Sunamura T. (1994) Rock control in coastal geomorphic processes. Transactions Japanese Geomorphological Union. 15 (3): 253-272. [ Links ]
Thiestle D. (1973) A taxonomic comparison of the American Upogebia (Decapoda: Thalassinidea) including to new species from the Caribbean. Breviora. 408: 1-23. [ Links ]
Torunski H. (1979) Biological erosion and its significance for the morphogenesis of limestone coasts and for nearshore sedimentation (Northern Adriatic). Senckenbergiana maritima. 11: 195-265. [ Links ]
Trenhaile, A. S. (1987) The geomorphology of rocky coasts. Clarendon Press, Oxford, 384 p. [ Links ]
Trenhaile, A. S. (2002): Rocky coasts, with particular emphasis on shore platforms. Geomorphology. 48: 7-22. [ Links ]
Viles H. A. (2012) Microbial geomorphology: a neglected link between life and landscape. Geomorphology. 157-158: 6-16. [ Links ]
Vita-Finzi, C. & Cornelius P.F.S. (1973) Cliff sapping by mollusks in Oman. Journal of Sedimentary Petrology. 43: 31-32. [ Links ]
Warme J.E. (1970) Traces and significances of marine rock borers, In: Crimes, T.P., Harper, J.C. (Eds.) Trace fossils. Seel House Press, Liverpool, 515-525. [ Links ]
Williams A.B. (1986) Mud shrimp, Upogebia from the eastern Pacific (Thalassinoidea: Upogebiidae). Memoirs of the San Diego Society of Natural History. 14: 1-60. [ Links ]