Introduction
Paravandellia phaneronema (Miles, 1943) (Siluriformes: Trichomycteridae) is an endemic fish parasite from the basins of the Magdalen and Cauca rivers and is the only species of the genus in Colombia (DoNascimiento, et al., 2017). As is usual with temporary hematophagous parasites of large fish species, P. phaneronema is often found with its intestines full of blood in the gills of the streaky catfish (Pseudoplatystoma magdaleniatum) (Buitrago, et al., 2007), hence its common name in English: “lancet, bloodsucker” or “son of streaky catfish” (Miles, 1947; Dahl, 1971).
The Trichomycteridae includes some of the most remarkable feeding specializations found among fishes. All the species of the subfamily Vandelliinae are exclusively hematophagous, sucking blood from the gills of other fishes (Kelley & Atz, 1964; Machado & Sazima, 1983; Zuanon & Sazima, 2005). Stegophilines and Vandelliines are popularly known in the Amazon as “candirus” and their accidental penetration of the urethras of humans and other mammals has been reported several times (Gudger, 1930). A detailed account of that remarkable phenomenon, however, has not yet been published. Their most conspic- uous synapomorphies lie in the structure of the opercular apparatus. The interopercle is modified into a compact structure with a large ventroposterior platform that supports a patch of odontodes. The opercle is also highly modified as an odontode-bearing structure. The parasitic forms also use the opercular apparatus for penetrating and anchoring themselves to their hosts (de Pinna, 1998).
By means of micrographs obtained from material examined under a scanning electronic microscope (SEM), we attempted to clarify the external morphologic adaptations of this species. We used repeated magnifications to study the evolutionary processes underlying their detection habits with neuromasts (mechanical receptors), the reduction and elongation of the body for penetration of the gill chambers, as well as some adaptations of morphologic character used for their anchor on the gills and the modification of teeth for the incision of the gill filaments and lamellae arteries of the host.
The biology of this fish has not been studied in detail. It is only known to inhabit muddy river beds (Román-Valencia, 1998; Maldonado-Ocampo, et al. , 2005). Our study was based on the examination of its external morphological adaptations using SEM images.
Methods
Using the scanning electron microscope at the University of Giessen (Germany), we obtained images from three of the eighteen specimens of P. phaneronema collected in February 1965 (Díaz del Basto, 1970) during an expedition conducted along the Cesar River, a tributary of the Magdalena River in Colombia. The samples were taken in the area known as Boca de La Miel (9º47´37” N; 73º37´12” W). They were swimming freely in a shoal near the water surface at the end of the dry period when the sediments of decomposing organic material from minor tributaries caused by the first rains had already appeared. The sediment load was inducing the fish to rise to the surface due to water eutrophication and oxygen insufficiency. The specimens were fixed in 3% formalin and later preserved in 70% ethanol.
Preparation of the specimens for scanning electron microscopy. The preparation of the three specimens (total length: 17 mm, 18 mm, and 20 mm each) for their observation under the SEM was carried out after a medium-sized dorsal-ventral dissection for assembly on the specimen mount. Specimens were immersed in 2% glutaraldehyde and 1% formalin (buffered in 0.1 M sodium cacodylate adjusted to a pH of 7.2) for two hours at room temperature. They were stored overnight (15-20 hours) in the fixative in a refrigerator (4-8 ºC). Specimens were chilled in 0.5 ml of tap water in a refrigerator (4-8 ºC) for 30 minutes. They were sequentially fixed by adding four drops of cold 4% glutaraldehyde and 2% formalin (buffered with 0.1 M sodium cacodylate, pH 7.2) every half hour until the final concentration of fixative reached 2% glutaraldehyde and 1% formalin. Fixation continued overnight (15-20 hours) in the cold. After fixation, all specimens were postfixed in 2% osmium tetroxide (buffered in 0.1 M sodium cacodylate, pH 7.2) for eight hours in the cold (4 ºC), dehydrated in a sevenstep acetone, and dried by the critical point method with CO using standard procedures (Eisenback, 1985). The samples were then sputter-coated with a thin layer (200nm) of gold to enhance conductivity, mounted onto aluminium stubs, and viewed with a Philips SEM XL20 scanning electron microscope at 10kV. The sturdiness of the sample was evaluated creating a 5 x 5 cm focusing window in the image display at 1000x for one minute and then reduced to lower magnification 4000x to determine whether any depression, changes or edges were left in the focusing window or could be seen by full frame observation.
Results
We obtained a series of back, side and ventral SEM images of the specimens of processed P. phaneronema which provided the details of the surface and external texture of dif- ferent morphologic structures of the anatomical adaptations for its parasitism. The SEM images are presented in eighteen micrographs as follows.
The general anterodorsal head view (Figure 1a) shows where the nostrils, the six cephalic pores and the operculum with a patch of odontodes are located. The head is spindle-shaped with a convex back profile and the egg-shaped snout has a distal disc whose function remains unknown. The eyes are only visible as circular nodes to the sides of the latter nostrils due to the SEM technology. The eyes in living specimens are rounded and entirely visible. A system of eight sensory cephalic pores spread obliquely from the snout back to the operculum presumed to be the mechanism for host location.
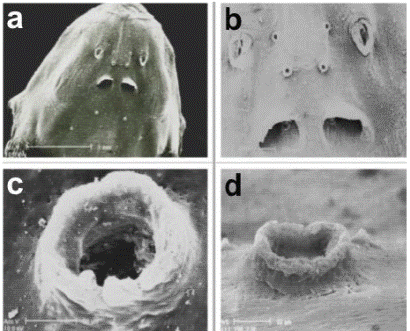
Figure 1 Head, dorsal view (a); two pairs of cephalic pores between the nostrils and a pair inside the posterior nostrils (b); detail of dorsal view of the cephalic pore (c); detail of a cephalic pore, side view (d)
The two pairs of nostrils and the first three pairs of cephalic pores are detailed in Figure 1b. The anterior nostrils have a spindle-shaped tubular structure with a frame of protuberant dermal flap. The distance between the anterior nostrils is wider than between the posterior nostrils, which are rounded and almost half covered by a proximal semicircular dermal fold. The first two pairs of cephalic pores form a square between the anterior nostrils. A third pair of cephalic pores is just visible inside the posterior nostrils.
The magnified frontal and side views (Figures 1c and 1d) show more details of these pores, which appear like structures in the shape of a chimney. Apparently, their function could be that of facilitating the water flow to the interior of the cephalic channels where the neuromasts are located, like a morphologic adaptation of the sensory system for orientation in the aquatic environment and for the reception of the hydrodynamic information produced by the host.
In the upper ventral torso (Figure 2a) the egg-shaped outline of the snout, the mouth cavity with numerous teeth, and two pairs of maxillary barbels can be observed: the longest and thinnest barbels do not reach the interopercular plate of odontodes while the slightly thicker internal barbels scarcely reach the labial rim. Nasal barbels are absent.
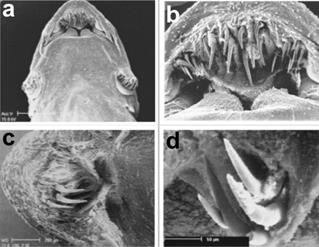
Figure 2 Head in ventral view (a); oral cavity (b); lower lip (c); two pairs of mandibular teeth under the lower lip (d)
From the ventral position, the mouth cavity is not as wide as the head, and the top lip forming a moderately sharp arch with straight sides can be seen (Figure 2b). The internal rims of the top lip form a sharp posterior margin covered with a crease of tegument. The premaxillary has two types of teeth: a) twelve central located canine teeth that are long and curved and rest on a semi-circular muscular lobe. These are probably the teeth adapted to anchor onto the gills of the host, an evolutionary mechanism that permits the parasite to perforate the arteries of the gills (phlebotomy); b) six shorter teeth are dispersed towards the flanks of the premaxillary teeth. The number of teeth can vary between individual specimens.
With another specimen we carried out a micro-dissection of the separated lobes of the lower lip to confirm the existence of teeth (Figure 2c). Two long and vaulted teeth under the tegument of every lobe can also be seen as a row of four vaulted teeth, all joined to the dentary, which are not functional (Figure 2d).
The left side of the trunk has a relatively curved back profile. The under part of the head is flat and continues obliquely from the pectoral fins to the posterior part of the body (Figure 3a). The image shows the hook-shaped teeth relatively parallel to the snout line. The maxillary barbels, the operculum, and the interoperculum with the odontodes plates, the operculum, and the pectoral fin can also be seen.
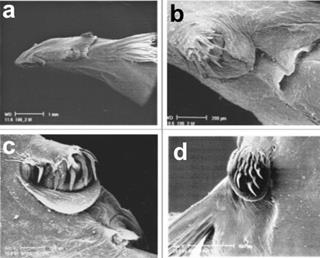
Figure 3 Head, left side view (a); interoperculum with straight odontodes and membranous fold (b); interoperculum with curved odontodes and membranous fold (c); details of opercular plate, dorsal view, and lateral line pores 7, 8 and 9 (d)
The interopercular odontodes varied between the analysed specimens, from eight long and pointed ones, barely curved (Figure 3b), up to twelve with an extremely hooked form (Figure 3c), possibly retractable to adhere to the gill plates of the host. The dermal retractable fold perfectly surrounds the odontodes in a semicircle to cover them and, hence, facilitate its mobilization inside the gills.
The operculum plate has 14 curved odontodes and a circular dermal flange, possibly for the recovery of the thorn- like odontodes (Figure 3d). Behind the five pores previously seen in Figure 1, there are other three pairs of cephalic pores: the sixth is located in the forward base of the operculum plate, the seventh in the posterior base, and the eighth, more distally at the end level of the pectoral fin. Channels between the pores and the lateral line connect these pores.
With an increased magnification of the operculum plate (Figure 4a), eighteen curved odontodes are visible, as well as the retractable odontode on the dermal crease and the seventh and eighth cephalic pores referred to above. Further magnification of the operculum plate (Figure 4b) shows the odontodes lightly raised from the individual fusiform-shaped dermal chamber. This proves that the odontodes are not rigid but retractable structures that possibly act as part of the active system of adhesion to hosts gills.
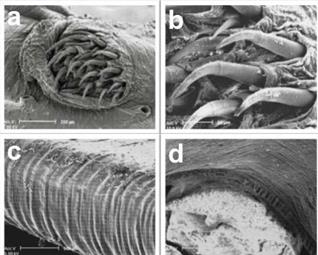
Figure 4 Detail of opercular plate, dorsal view, and lateral line pores 7 and 8 (a); detail of retractable odontodes on a fusiform base (b); striated abdominal musculature for blood storage (c); cutting of striated abdominal muscles for blood storage (d)
The details of the external striated abdominal musculature developed to satisfy the enormous dilatation potential are shown in Figure 4c. As soon as the blood sucking ends, the specimens release themselves from the gills with the abdomen full of blood and they continue the digestive process in their habitat.
With a transversal cut of the abdominal musculature, highly flexible muscular fibres can be observed with very elastic connections to the abdominal cavity. These enable the storage of the enormous quantity of blood extracted from the host (Figure 4d). Figures 5a and b could be superficial neuromasts. Often the cupula is lost in the preparation and so the hair bundles of the sensory cells.
Discussion
Parasite and host relationship. After highlighting the dynamics of the interrelation between parasite and host, it is time to consider the complex physiological osmoregulation and the function of the mechanoreceptor organs of the teleost freshwater fish. There appear to be two possible operating modes of this parasitic fish for detecting its host: the commonly accepted chemical attraction to the ammonia waste of the host and the hypothesis we present in our study, i.e., the remote perception of the host by means of the parasites’ canal or superficial neuromasts. Our results offer a possible solution to this question, but further experimental research on the sensory system of this genus is required.
The function of neuromasts in remote detection of hosts. By means of the SEM images we were able to detect alternative or complementary mechanical reception centers on the structures viewed. The lateral line is a hydrodynamic sensory system and fish use its information in many of their behaviors including spatial orientation, rheotaxis, schooling, prey detection, predator avoidance, and intraspecific communication (Bleckmann, 1994).
The smallest functional unit of the fish lateral line is the neuromast, a sensory structure that can stand out on the skin (superficial neuromast) or in the fluid-filled canals (canal neuromast) that usually open to the environment through a series of pores (Puzdrowski, 1989; Schmitz, et al., 2008; Bleckmann, et al., 2010).
The organs responsible for this lateral line system consist of units of gelatine provided with nerve connections. These neuromasts form groups of cells covered by a gelatinous dome moved by the water which, in its turn, moves the cilia of the hair cells in charge of sending messages to the brain. The neuromasts of the body are supplied by branches of the vagus nerve (X) and those of the head, by the facial nerve (VII) and the glossopharyngeal nerve (IX). The nerves send signals to the ciliated cells for their activation in the brain and these locate and identify possible sources of the changes detected in the movement of the water (Norman, 1966).
The hair cell is the main sensory cell of the lateral line system. Like all hair cells, these have a hair bundle at their apical surface comprised of up to 150 stereovilli that grow longer from one edge of the bundle to the other. A single true kinocilium always occurs eccentrically at the tall edge of the hair bundle. Within the sensory epithelium of a neuromast, the hair cells are oriented into two opposing directions that define the most sensitive axis of a neuromast (Flock & Wersäll, 1962). The hair bundles protrude into a cupula that connects the bundles with the water surrounding the fish or with canal fluid. Lateral line neuromasts are innervated by afferent and efferent nerve fibers. Morphological and physiological studies indicate that a single afferent fiber most likely innervates only hair cells with the same orientation (Münz, 1985; Bleckmann, 1994).
The hydrodynamic information provides the basis for many behavioural decisions. There is evidence that fish use it for prey detection, predator avoidance, intraspecific communication, schooling, object discrimination, entrainment, and rheotaxis (Bleckmann, 1993, 1994; Montgomery, et al., 1997).
As mentioned, canal neuromasts are pressure gradient detectors. One of the stimulus parameters that is clearly encoded in the central lateral line pathway is the direction of the motion of an object, i.e., some central lateral line units require a certain temporal and spatial pattern of water motions (Müller, et al., 1996; Wojtenek, et al ., 1998; Plachta, et al ., 2003; Bleckmann & Zelick, 2009).
Some authors have suggested that the Vandellia species in the Amazon basin are unable to actively absorb the blood of their host, therefore, they make an incision in the main gill arteries and use the blood pressure of the host to pump the blood into their intestines (Zuanon & Sazima, 2004).
The concept that nitrogen waste products from the host attract the parasite is questionable. On the basis of published values for teleost fish plasma ionic concentrations (Keys, 1931; Smith, 1932; Krogh, 1939) and the knowledge that freshwater teleosts have significantly lower plasma salt contents, we can conclude that they are still distinctly hyperosmotic in relation to their environment (~300 mOsm/l vs ~1 mOsm/l), Na and Cl¯ being the dominant ions in the plasma. Because of these osmotic and ionic gradients, freshwater teleosts are overhydrated. These authors also knew that, as a consequence of these osmotic and ionic gradients, freshwater teleosts produce large volumes of dilute urine (Evans, 2008).
The salts are removed from the blood of bony fish in two ways: through the kidneys (mainly bivalent ions) or else through the gills (monovalent ions). As noted above, freshwater fish internal fluids are hypertonic to the external medium. In freshwater bony fish the main osmoregulatory function does not lead to the retention but to the excretion of surplus quantities of the water which has entered osmotically (Nikolsky, 1963).
The teleost fish gill is a multi-purpose organ, specialized in respiratory gas exchanges, the clearance of waste products from the nitrogenous metabolism, and the maintenance of acid-base and mineral balances. Structural studies reveal a complex epithelium. The chloride cells are almost certainly the site of ion exchange in relation to salt balance. Functional studies show that the gill is responsible for the net absorption of Na+ and Cl- present in fresh water and that there is a coupling between endogenous NH4 +, or H+ and HCO3 - excretion and Na+ and Cl- absorption. Biochemical studies have revealed two enzymes that play important roles in the ionic pumps: carbonic anhydrase and Na-K activated ATPase (Maetz, 1971).
The large electrochemical gradients for both Na+ and Cl- between fish plasma and the medium in freshwater dictate that there should be mechanisms for the extraction of both of these ions from extremely diluted environments. Given that Na+ or Cl- can be taken up independently by fishes, Krogh (1938) suggested that the extrusion of internal counterions, such as NH4 +, H+, or HCO3 -, was probably chemically coupled to Na+ and Cl- uptake in order to maintain reasonable electrical gradients across the gills. The gill epithelium of fish possesses a suite of transport steps for Na+ and Cl- that also functions in the excretion of acid vs base and ammonium ions. The fish gill is also the site for the excretion of excess nitrogen in the form of ammonia. The multistring nature of the junctions between adjacent cells in the freshwater fish gill presumably hinder paracellular diffusion of NH4 + and apical H+-ATPase will not extrude NH3. Given these limitations, it is generally assumed that the dominant and possibly sole mode of ammonia extrusion across the freshwater fish gill is via the paracellular diffusion of NH3 (e.g., Wilkie, 1997).
Interestingly acidification of the apical unstirred layer by either proton extrusion or carbonic anhydrase-mediated hydration of expelled CO2 would facilitate NH3 diffusion by reducing the NH3 to NH4 +. This would maintain the partial pressure gradient for NH3 diffusion across the gill (Evans, et al., 1999).
These considerations on the regulation and the excretion of teleost freshwater fish lead us to question the supposed attraction of the parasite for nitrogenous metabolic products originating from the host. Considering the urea concentrations expelled in the urine or that of the ammonia (toxicant) that has to be dissolved by the gills and eliminated in the river currents in the form of non-poisonous ammonium, it is unlikely that they work as the only stimulus adapted for the attraction of these parasites. These suggestions have not been experimentally verified. Likewise, the anecdotes on the penetration of the hematophagus “candirú” into human orifices when attracted by urine have not been scientifically checked either. Experiments carried out on candirú behaviour in aquaria, together with Carassius aureus and Cichlasoma auratus, throw doubts on their preference for this fish species and on their reaction to attractive chemicals (aminoacids, ammonia, mucilaginous textile of fresh fish and human urine) when added to the aquarium. Instead, a visual interaction between parasite and host seems a more likely explanation for the parasitic attack (Spotte, et al ., 2001).
Conclusions
The crucial question for our study was to try to explore the adaptations that provide P. phaneronema with the ability to reach the nutritive source of the gills once it leaves its habitat on the muddy and sandy river beds using SEM images.
Some of the results of basic research on the lateral line sensory system and for osmoregulation in teleost freshwater fish have been useful in the discussion regarding the dynamics of the interrelation between parasite and host. A review of published studies on the structure and function of the neuromasts supported our hypothesis on the remote sensing of the host through mechanoreceptors (canal and superficial neuromasts) of the lateral line system and the skin. The opinion that the traces of nitrogenous waste products of the host are the attractants for the parasite seems uncertain in view of the papers published on osmoregulation and, therefore, it seems necessary to conduct new relevant investigations in this context.
According to the osmoregulation mechanisms of dilute urine, the ionic interchange at the gill epithelium, and the extrusion of nitrogen wastes as non-poisonous ammonium by fresh-water teleost fish, we question whether the attraction of the parasite responds merely to a chemical nature, as claimed by a variety of studies.
The SEM micrographs show details of the external structures of the species never before seen at such magnification and they verify the optimized adaptations for the parasitic way of life. Detailed analyses lead to the supposition that the geometrical arrangement of cephalic pores that connect the channels of the lateral line system with the surrounding aquatic environment are the key components of the sensory mechanical reception system.
Therefore, we suggest that in order to carry out the parasitic attack, P. phaneronema needs two neurosensory components. First, they utilise the canal neuromasts within the cephalic pores, as well as the superficial neuromasts on the skin for remote detection and subsequent tracking of the host. The second step is carried out on or near the host and consists of the location of the gills, the penetration of the gill camera, the incision of the arteries and the blood sucking, undoubtedly as a result of the cooperation, coordination, and synergy with other sensory organs.