Introduction
Grapes are one of the most economically important crops in the world (Khilare & Chavan, 2010; Lei, et al., 2016). In Colombia, the planted area is 2,500 ha and the production in 2016 was 30,156 t with an average yield of 10.51 t.ha-1. The department of Valle del Cauca, with a harvested area of 1,940 ha, produced that year 24,379 t of grape, which represents 80.84% of the national production (Agronet, 2018).
One of the pathologies of fungal origin affecting the crop is anthracnose, which has been reported in Japan (Shiraishi, et al., 2006), India (Khilare & Chavan, 2010; Shetty, et al., 2014), China (Lei, et al., 2016) and Australia (Greer, et al., 2011). The disease, caused by species of the genus Colletotrichum, affects a wide variety of crops and fruit trees including grapes (Vitis vinifera) (Lei, et al., 2016), especially in tropical and subtropical areas (Hyde, et al., 2009; Wikee, et al., 2011; Cannon, et al., 2012; Lei, et al., 2016); this genus has been designated as the eighth most important group of pathogenic plant fungi in the world for its economic and scientific importance (Dean, et al., 2012).
In Virginia, United States, the losses caused by the disease between 2010 and 2014 ranged between 15 and 30% significantly reducing the quality of the wine as well (Nita, 2015). In some provinces of China, the losses by insects and diseases, among which anthracnose stands out, reached 50% (Peng, et al., 2013). In Colombia, there is still no data on losses associated with this disease, although in the municipality of La Unión, Valle del Cauca, the disease has been present since 2016 where symptoms were initially detected in a batch of Red Globe table grape variety. From then on, farmers, without knowing its true etiology, have applied different chemical products with no success because the pathology is spread by foci within the same batch.
The disease occurs mainly on ripe fruits (Lei, et al., 2016), although infections are also present in developing fruits (Gil, et al., 2002; Jiang, et al., 2014) as has been observed at La Union vineyards (López-Zapata, et al., 2019). Grapes infected with Colletotrichum present lesions characterized by dark circular spots on the epidermis resulting in total damage to the berries that are then covered with masses of salmon-colored spores (Shiraishi, et al., 2006).
The etiology of the disease is complex: although in the United States it was initially identified as C. gloeosporioides (Daykin & Milholland, 1984), different species have been found in other wine-growing regions causing very similar symptoms. In China, for instance, C. viniferum, Colletotrichum sp. (Lei, et al., 2016), C. fruticola, and C. gloeosporioides (Peng, et al., 2013) have been identified. C. gloeosporioides and C. acutatum have been found in Australia and in the United States (Greer, et al., 2011; Nita, 2015). In India, the disease is attributed to Gloeosporium ampelophagum (Khilare & Chavan, 2010) and recently in Colombia, an isolate genetically close to C. aenigma and C. siamense, both belonging to the C. gloeosporioides complex (López-Zapata, et al., 2019), was identified as the agent causing anthracnose in grape fruits.
Chemical fungicides occupy the first place in the control of plant diseases (Gaviria, et al., 2013). In grapes, their applications must be frequent to ensure the quality for markets and consumers (Shiraishi, et al., 2006). However, Colletotrichum species present different responses to fungicides possibly due to continuous applications. For example, wild C. gloeosporioides isolates are sensitive to carbamate methyl-benzimidazole fungicides while C. acutatum is tolerant to this group (Han, et al., 2018). Likewise, different studies indicate that carbendazim, of the benzimidazoles group, has lost effectiveness on G. ampelophagum in grapes (Khilare & Chavan, 2010; Sawant, et al., 2014) and C. gloeosporioides in strawberries and yam (Han, et al., 2018). Similarly, C. gloeosporioides isolated from mango and strawberry in Taiwan showed resistance to benzimidazoles.
This behavior has led to suggest that the difference between resistant and moderately resistant isolates is due to the application of the product at doses higher than 100 μg.mL-1 (Chung, et al., 2010), especially benzimidazoles which have been historically used for the management of anthracnose in grape, strawberry, and mango crops (Xu, et al., 2014) resulting in resistant pathogens genotypes that can remain dominant in a field even after suspending the application of this group of fungicides (Hwang, et al., 2010). Given that the true etiology of this vine pathology is not well known in Colombia, knowledge of the in vitro effect of fungicides on C. gloeosporioides is of vital importance to establish efficient and lasting management measures to avoid production decreases and resistance problems that are common in the wine-growing regions of the world.
Materials and methods
Fungal isolates
We collected 200 fruits showing the symptoms of the initial stages of the disease, such as the reddish-brown discoloration, and those of the most advanced stages, i.e., sunken and necrotic Red Globe berries, following a rectangle sampling pattern in an eight-year-old vineyard of approximately three ha in physiological stage 79 (Lorenz, et al., 1994) located in the municipality of La Unión, Valle del Cauca. The berries were stored in transparent plastic bags and taken to the plant pathology laboratory of the Facultad de Ciencias Agrícolas at Universidad de Caldas for their analysis.
Tissue sections of approximately 5 mm2 including healthy and diseased tissue were cut and subsequently disinfected with a 1% sodium hypochlorite solution for 1 min; consecutively, they were transferred to 70% alcohol for 50 s, washed three times with sterile distilled water, and then placed on sterile paper towels to remove excess moisture. The disinfected tissue was placed into Petri dishes containing Merck potato-dextrose-agar (PDA) (39 g.L-1) culture medium and they were incubated at 28°C for 3 to 7 days with periodic observations. After incubation, agar sections taken from the active growth site of the colonies were aseptically transferred to new Petri dishes with PDA.
We obtained an isolate identified as Colletotrichum sp. for its macroscopic and micro scopic morphology. The pathogenicity of grapes in physiological stage 79 (Lorenz, et al., 1994) was subsequently tested and Koch's postulates were verified. Later, we determined that it belonged to the C. gloesporioides species complex using molecular tools (López-Zapata, et al., 2019) and with it, we carried out the sensitivity tests of the four fungicides.
In vitro sensitivity bioassay
We determined the efficiency of two systemic fungicides, benomyl (Benlate®50 WP) and carbendazim (Bélico® 500 SC), and two protectants, chlorothalonil (Odeon® 720 SC) and dodine (Syllit®400 SC) with the following three concentrations of each product corresponding to the commercial dose, half the commercial dose, and twice the com mercial dose expressed in grams per liter or milliliters per liter: benomyl, 0.5, 0.25, and 1 g.L-1; carbendazim, 0.6, 0.3, and 1.2 mL.L-1; chlorothalonil, 2.4, 1.2, and 4.8 mL.L-1, and dodine, 1.6, 0.8, and 3.2 mL.L-1. Product effectiveness was measured by the inhibition of mycelial growth and the sporulation.
Inhibition of mycelial growth
We used dilutions on Petri dishes containing PDA and added each of the concentrations when the medium reached a temperature of 45°C. Once solidified, a 5 mm portion of the active growth site of the fungus in a five-day culture was placed in the center of the Petri dish with mycelial growth making direct contact with the medium supplemented with the respective fungicide (Han, et al., 2018). As a control we used a seeding under similarconditions on PDA but no addition of fungicide. The dishes were incubated at 28°C until the control reached the maximum growth and the percentage of inhibition was calculated using the formula:
where I is the percentage of reduction in pathogen growth, c, the growth of the colony (cm) in the control, and t, the growth of the colony (cm) in the treatment.
Inhibition of the sporulation
Once the control reached the maximum growth, circular portions of 1 cm in diameter were taken from the active growth site of each treatment and the corresponding repetitions and then placed on Petri dishes containing 5 mL of sterile distilled water; with the help of a handle, the mycelium was removed gently and the spores were counted by product, treatment, and repetition with a hemocytometer. The same process was carried out with the control.
Effect of fungicides on the mycelial morphology
We observed portions of mycelium from the cultures corresponding to the commercial doses of each of the four fungicides using an environmental scanning electron microscope (ESEM) and we processed the images obtained with the ImageJ program (Ferreira & Rasband, 2012).
Statistical analysis
We used a completely randomized statistical design with thirteen treatments and five repetitions. Experimental units corresponded to the Petri dishes. We created an Excel database, entered the information from the laboratory, and analyzed it using the Statistical Analysis System (SAS) v.9.0. We used an analysis of variance to evaluate the variables and averages were compared with a 5% probability Tukey test. We used the following mathematical model:
where γij equals the value of the response variable of the experimental unit associated with the i-th treatment, j-th repetition; μ corresponds to the general average of the response variable in the experiment; ti is the effect of the i-th treatment; εij is the error of the experimental unit associated with the i-th treatment, j-th repetition; i equals 1, 2, 3,4...13, and j equals 1, 2, 3, 4, 5.
Results
Effects on the development of the colony, mycelial growth, and sporulation of the fungus
Our results indicated that all the treatments showed highly significant statistical differences (Pr> F = <0.0001) between doses as to the diameter of the colony, the percentage of inhibition, and the number of spores, as well as to the interaction active ingredient per dose at a 95% reliability level (Table 1).
All the fungicides inhibited C. gloeosporioides mycelial growth compared to the control (Table 1). However, benomyl was the best fungicide, with significant statistical differences compared to the other products and doses, totally inhibiting the fungal growth and sporulation at twice the commercial dose (1g.L-1) (Figure 1). Although carbendazim at double the commercial dose (1.2 g.L-1) showed a lower effect on mycelial growth, it completely inhibited the sporulation of the fungus as did benomyl. Both benzimidazole-group products had similar effects on sporulation, which demonstrates the high effectiveness of the systemic fungicides on this fungus (Table 1).
Table 1 In vitro effectiveness of four fungicides on the diameter of the colony, mycelial growth, and sporulation of Colletotrichum gloeosporioides
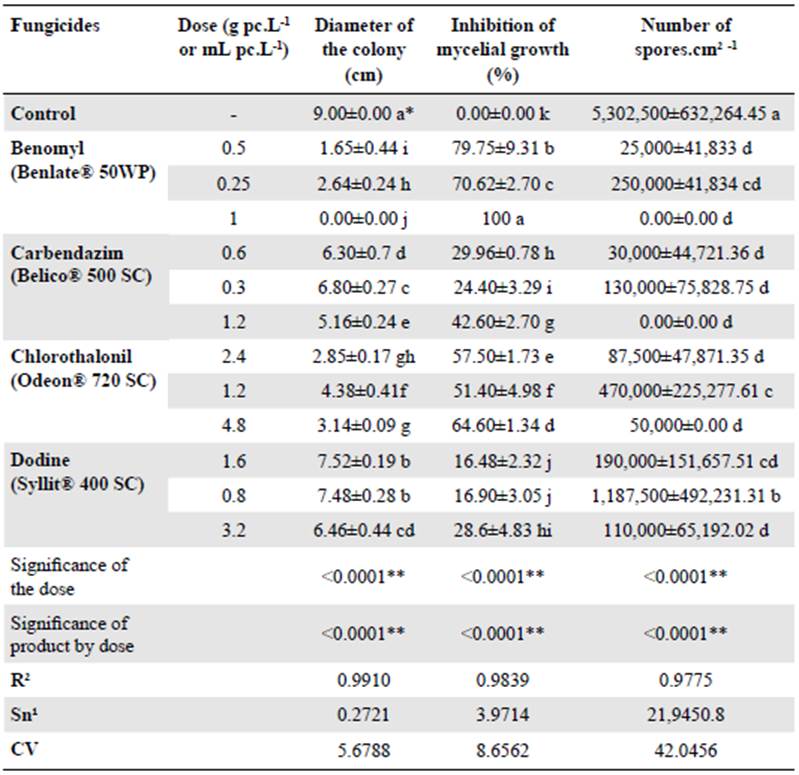
Numbers followed by the same letters are not significant at 5% probability.
** Highly significant
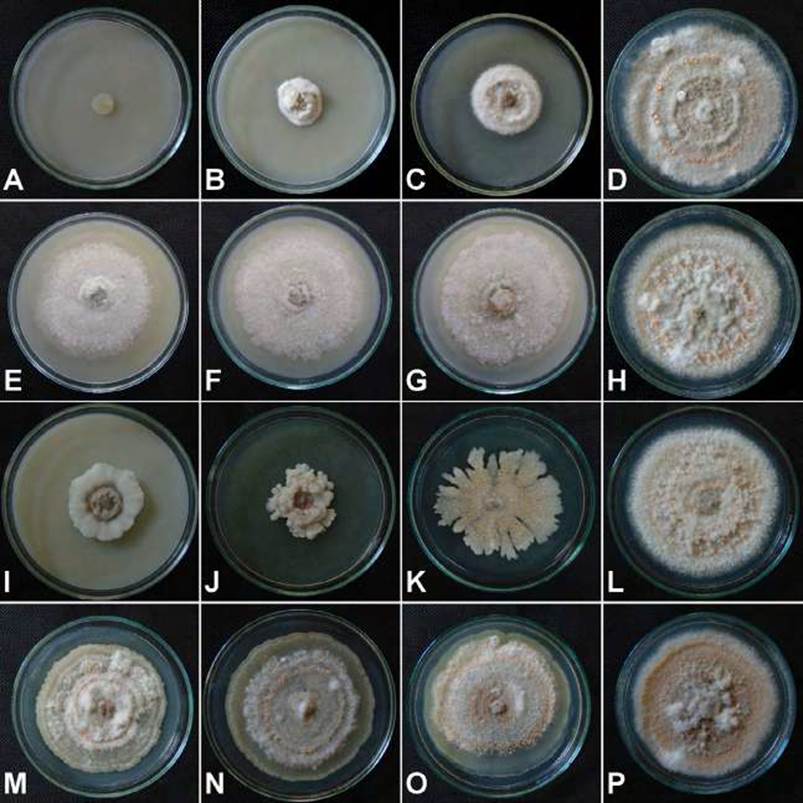
Figure 1 Effect in vitro of four fungicides on the diameter of Colletotrichum gloeosporioides colony from grape (Vitis vinifera). A-D. Benomyl in doses of 1, 0.50, andd 0.25 g.L-1 and control, respectively. E-H. Carbendazim in doses of 1.2, 0.6, and 0.3 mL.L-1 and control, respectively. I-L. Chlorothalonil in doses of 4.8, 2.4, and 1.2 mL.L-1 and control, respectively. M-P. Dodine in doses of 3.2, 1.6, and 0.8 mL.L-1 and control, respectively.
Among the protective fungicides, chlorothalonil at double the commercial dose was the best followed by dodine. Based on the recommended commercial dose, this product had the least effect. In general, as the dose of the four fungicides was reduced, the effectiveness decreased in the three variables evaluated (Table 1).
Effect on the development and morphology of hyphae
The effect of the four fungicides at commercial doses on the development and morphology of the hyphae and sporulation of C. gloeosporioides was corroborated through environmental scanning electron microscopy (ESEM) showing a marked inhibition of the development and pronounced deformation of the hyphae, septum formation, and little sporulation in comparison with the control (Figure 2).
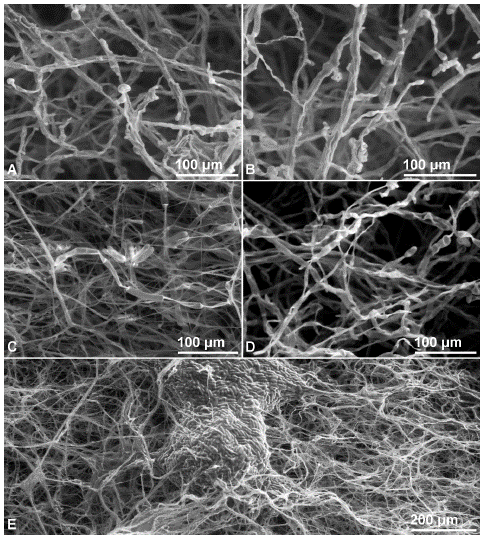
Figure 2 ESEM image of Colletotrichum gloeosporioides using the commercial doses of the evaluated products. A. Treated with 0.5 g. L-1 of benomyl. B. Treated with 0.6 g.L-1 of carbendazim. C. Treated with 2.4 mL.L-1 of chlorothalonil. D. Treated with 1.6 mL.L-1 of dodine. E. Absolute control, not treated.
With benomyl and carbendazim, distortion, deformation, excessive branching, and the collapse of the hyphae were evident, as well as their granular and swollen appearance; no spores were observed in the analyzed portions. The hyphae in formation were thinner presenting rough surfaces (Figure 2 A,B).
With chlorothalonil, the hyphae became weak, translucent, and empty with irregular morphology that included thickened sections with reduced sizes. Additionally, clusters of conidia were observed (Figure 2C). With dodine, hyphae were disrupted and appeared coiled, shrunken, and curled forming blisters while spores were apparently dehydrated and deformed (Figure 2D).
In contrast to the effect of the fungicides, the control grown on PDA produced long, dense, uniform, tubular, and smooth surface hyphae, as well as abundant sporulation (Figure 2E).
Discussion
With the increased doses of the four fungicides under study, the mycelial growth of the fungus decreased, although fungi toxicity varied notably depending on the fungicides and their concentrations; the systemic fungicides were more effective than the protectants (Table 1) but, as a general trend, the growth of the pathogen decreased with the products compared to the control. However, after 15 days of incubation of C. gloeosporioides at 28 ± 2°C, benomyl was the best product inhibiting completely the growth of the fungus.
Our results confirmed other findings regarding the antifungal activity of benomyl on Colletotrichum species: C. capsici from peppers whose growth was 100% inhibited (Subhani, 2015); C. gloeosporioides from mango with an inhibition percentage ranging between 75% and 90% (Kumar, et al., 2007), and C. gloeosporioides and C. acutatum from citrus whose growth was inhibited at a concentration of 1.0 μ a.i. L-1 (Peres, et al., 2004).
The percentage of inhibition of C. gloeosporioides mycelial growth with benomyl and carbendazim differed statistically, although they belong to the same chemical group, which agrees with Gaviria, et al. (2013), who found inhibition percentages for C. acutatum ranging from 8.25% to 46.50% using the commercial doses.
Although strictly speaking no resistance to carbendazim was found in our study, given that sporulation was similar to that obtained with benomyl, it is necessary to consider that its loss of effectiveness has been reported in different crops and species of the pathogen: C. gloeosporioides isolated from strawberry (Fragaria sp.) and grape (Han, et al, 2018; Lin, et al., 2016), C. siamense and C.fructicola from strawberry and yam (Dioscorea sp.), respectively, whose level of resistance to the fungicide was different depending on the region of origin of the isolate (Han, et al, 2018).
In the particular case of anthracnose, the continuous use of carbendazim for managing the pathogen has reduced its sensitivity (Deokate, et al, 2012) and it has been documented that highly resistant isolates have not been controlled even at concentrations of 100 |ig.mL-1 (Narkar, et al, 2012). In the case of grapes, more than 1% of the active ingredient was needed to obtain effective control.
As we mentioned already, the effect of carbendazim was comparable to that of benomyl on the sporulation of C. gloeosporiodes, which has been previously demonstrated on this pathogen in grapes, with very similar effectiveness to that of the quinone inhibitors and demethylation (Shetty, et al, 2014), as well as on C. capsicum in chili pepper (Capsicum annuum) (Kumar, et al, 2016).
The effect of carbendazim on Colletotrichum species is differential: it is more effective for the control of species of the C. gloeosporioides complex than for C. acutatum (Cao, et al., 2017). This difference in the efficacy of fungicides according to the fungus species has also been documented for benomyl.
According to Peres, et al. (2005), doses of 0.1 to 1.0 μL.mL-1 inhibit the growth of some species of Colletotrichum while in C. acutatum, the inhibition ranges between 20% and 50%, which is consistent with the results reported by Greer, et al. (2011) who observed that benomyl inhibited mycelial growth by 97% and 53% in C. gloeosporioides and C. acutatum isolates, respectively. Likewise, it inhibited the mycelial growth of C. gloeosporioides in strawberry (Rubus glaucus) by 74% to 83% and between 7% and 16% in C. acutatum while with carbendazim it was 85% and 33%, respectively (Gaviria, et al., 2013). However, Santamaría, et al. (2011) in their study on the in vitro effect of benomyl on C. gloeosporioides and C. dematium isolated from papaya did not observe any effect on the development of these fungal species even at concentrations of 4 g pc.L-1 of water.
These studies show once again the versatility of pathogens to overcome the active ingredients and their mechanisms of action, which in the case of benzimidazoles has been widely known and reported on species such as C. gloeosporioides, C. acutatum, C. cereale, and C. siamense (Hu, et al., 2015; Peres, et al., 2004; Wong, et al., 2008).
In this sense, the Fungicide Resistance Action Committee - FRAC (2020) stated that the benzimidazole group fungicides (including carbendazim) are highly conducive to the development of resistance in many fungal species, which has already been widely demonstrated for benomyl. Although it is not yet the case, this may alert grape growers about the implications of ignoring the loss of effectiveness of active ingredients, as C. gloeosporioides isolates resistant to benomyl have been recovered after more than 10 years of ceasing its application and even in places where its use was not known (Peres, et al., 2004).
Resistance to these products in grape crops has resulted from the excessive use of chemicals to control anthracnose in recent years because, until the 1980s, studies conducted on muscadine grapes showed the effectiveness of benomyl at a dose of 100 μg a.i. mL-1 (Daykin & Milholland, 1984). Likewise, the loss of efficacy of benzimidazoles has been explained by the continuous use of this group of fungicides for the control of Botrytis cinerea, causing pathogenic strains of C. acutatum to be less sensitive to benomyl and damage the bunch (Greer, et al., 2011), also reducing the effect of the fungicide when it is applied near the time of grape sprouting (Shiraishi, et al., 2006).
After benomyl and carbendazim, chlorothalonil (chloronitriles group) was the active ingredient inhibiting the mycelial growth of C. gloeosporioides and with similar effects on sporulation using the commercial dose. This broad-spectrum protectant fungicide is widely used in agriculture and it has shown significant effects against anthracnose reducing vine leafs damage caused by Elsinoe ampeliana (Magarey, et al., 1993), as well as the incidence of anthracnose in pepper (Capsicum annuum) (Kumar, et al., 2016) and of C. acutatum sporulation in cherry (Prunus cerasus) (Tóth, et al., 2012).
The promising results of chlorothalonil allow for suggesting it as an alternative for the management of the disease and as prevention against the resistance induced by the continuous, excessive, and undue use of benzimidazoles. Considering the time of appearance of the first symptoms of the disease, preventive applications would be advisable. Besides, the product can also be effective against other vine pathogens such as Phomopsis viticola inhibiting the viability of the spores and its mycelial growth (Krol, 2005). However, although chlorothalonil showed more than a 50 % inhibition effect in this study, it should be used carefully as a mild activity has been documented on C. gloeosporioides and C. dematium (Santamaría, et al., 2011) and resistance in other Colletotrichum species (Andriani, et al., 2017).
Dodine is a fungicide used to control diseases in apples, pears, nuts, peaches, cherries, and strawberries, as well as a biocide and preservative (Çördük, et al., 2016). In Colombia, it is registered for the control of flowers, bananas, and coffee pathogens; in other countries, its bactericidal activity has been studied mainly for the disruption of the cytoplasmic membrane and cell death, and on fungi for the loss of amino acids and materials absorbing UV rays (Cabral, 1991).
The results obtained using dodine against C. gloeosporioides sporulation had no statis tical difference compared to chlorothalonil at twice the commercial dose, which corrobo rates its point and mechanism of action. It is also worth noting that, although the diameter of the colony was not noticeably affected in comparison with the other products, we observed a decrease in the volume of the mycelium. However, since it is not recommended for this fungal species in the country, laboratory tests to corroborate the average lethal dose and subsequent field evaluations to analyze possible side effects are necessary, as studies have shown that it can cause oxidative stress by inhibiting protein synthesis and degradation, although it can also stimulate plant defense systems (Çördük, et al., 2016).
The applications of chemical synthesis products are essential for disease control and to ensure the quality that markets and consumers expect (Shiraishi, et al., 2006), which is not alien to the vine crops at La Union, Valle del Cauca, where the use of fungicides is a frequent practice resorting to benzimidazoles among the products used. This would partially explain the behavior of benomyl and carbendazim in this study and constitutes an alert on the implications of an eventual resistance of C. gloeosporioides to this type of product.
Given the possible resistance to benzimidazoles, the quinone outside (QoI) and demethylation inhibitors could be an alternative for the management of C. gloeosporioides resist ant isolates in grapes (Shetty, et al., 2014). However, this hypothesis should be validated on isolates from a specific region as in some areas of the United States, for example, several isolates of this fungus have already shown resistance to QoI fungicides (Nita, 2015).
The mixture of benzimidazoles with dietofencarb is usually an alternative to handle resistant C. gloeosporioides isolates. However, this is not recommended in grapes without taking strict measures to avoid the development of resistance (Lin, et al., 2016), even more so when it has been demonstrated that there is no specificity between pathogen and host as C. acutatum isolates from different crops can infect grape crops further evidencing possible cross-infection (Whitelaw-Weckert, et al., 2007), which very possibly may be happening in the study area.
Additionally, it is important to consider other aspects recently elucidated regarding the role of temperature in the effectiveness of fungicides on resistant isolates through its inhibitory effect on them, which underlines the need to have information on the sensitivity of resistant isolates since this can have an impact on the strategies for applying fungicides (Ma & Michailides, 2005).
Conclusions
Benomyl was the most efficient fungicide in in vitro conditions for the control of C. gloeosporioides causing anthracnose in grapefruits inhibiting mycelial growth and the production of spores at twice the commercial dose.
Although the effectiveness of dodine on the mycelial growth and sporulation of the fungus was the lowest in comparison with the other fungicides, it deserves more detailed laboratory and field studies on its effectiveness as an alternative against C. gloeosporioides.
Considering that fruit infections occur in the early physiological stages, apparently from the flowering phase itself, it is important to apply a preventive fungicide at this time followed by applications to protect the berries during their development.
Using various strategies such as the collection and destruction of diseased fruits and the combination of systemic products with protectants would help to mitigate the effects of the pathogen.