Introduction
Despite the advances (Eslava, 1974; Snow, 1976; Mesa, et al., 1997; Poveda & Mesa, 2000; Pabón-Caycedo, et al., 2001; Urrea, et al., 2019), there is no satisfactory theory to explain the climate of Colombia and the causes of its regional, seasonal, and inter-annual variability. The present work contributes to the development of such a theory considering the circulation induced by the latent heat released from the copious rainfall over the country, particularly, the Pacific coast. The theory of the linear equatorial waves provided the main elements for explaining the general circulation and the seasonal and inter-annual variability.
The low-level lands on the Pacific coast of Colombia (northwest of South America) are one of the most significant places on Earth where air raises. It is also the rainiest region on the planet (López, 1966; Arnett & Steadman, 1970; Snow, 1975; Nieuwolt, 1977; Eslava, 1993; Poveda & Mesa, 1997; Poveda & Mesa, 2000; Mapes, et al., 2003; Sakamoto, et al., 2011; Alvarez-Villa, et al., 2011; Durán-Quesada, et al., 2012) and one of the largest diabatic heat sources (Zhang, et al., 2017). The existence of a low-level westerly jet, known as Chorro del Occidente Colombiano or CHOCO jet, has been the traditional explanation for such a high average annual precipitation (over 13,000 mm). This jet interacts with topography and evapotranspiration from the tropical rainforests of western Colombia (Poveda & Mesa, 1997; Poveda, et al., 2006; Poveda, et al., 2014).
Poveda & Mesa (2000) associated the dynamics and strength of the CHOCO jet with the sea surface temperature gradient between the cold tongue of Equator-Perú and the warmer Pacific in front of Colombia. The change of direction of the cross-equatorial trade winds favors the development of the jet (Poveda & Mesa, 2000; Poveda, et al., 2002; Poveda, et al., 2006; Kessler & Gourdeau, 2006; Amador, et al., 2006). The corresponding change in the sign of the Coriolis acceleration, the predominant north-south coast orientation, as well as the land-sea temperature gradient and the friction gradients, contribute to establishing a westerly jet centered at 5°N. For these reasons, the traditional CHOCO jet explanation is the so-called Stensrud's mechanisms (Stensrud, 1996). Furthermore, the latent heat release reinforces the CHOCO jet due to the high amounts of condensation and rainfall. Most of this condensation occurs in mesoscale convective systems (MCS), which, in their turn, favor the wind surface convergence (Velasco & Fritsch, 1987; Poveda & Mesa, 2000; Mapes, et al., 2003; Zuluaga & Poveda, 2004; Mejía & Poveda, 2005; Poveda, et al., 2006).
Another essential feature of the regional circulation is the so-called Caribbean jet, located between the north of South America and the Greater Antilles (Amador & Magana, 1999; Poveda & Mesa, 1999; Magaña, et al., 1999). This other jet also transports large amounts of moisture from the Caribbean Sea to the Eastern Pacific, northern South America, and Central America (Xie, et al., 2005; Mestas-Núñez, et al., 2007; Wang, 2007; Wang & Lee, 2007; Whyte, et al, 2008; Amador, et al, 2006; Amador, 2008; Cook & Vizy, 2010). During most of the year, it is possible to observe how the winds coming from the Caribbean are recurved towards the south crossing the Papagayo Gulf and the Panama isthmus (Chelton, et al., 2000; Xie, et al., 2005; Romero-Centeno, et al., 2007; Serra, et al., 2010). Besides, sometimes the winds cross over Tehuantepec (Romero-Centeno, et al., 2007) to merge with those winds from the CHOCO jet and turn to the west to reach the Colombian coast (Poveda, et al., 2014). None of these mechanisms explaining the curvature of the CHOCO jet can explain the turn of the winds coming from the Caribbean jet to Colombia. Additionally, there is a low-level pressure in Panamá (Called the "Panamá Low"), which could explain the main features of wind recurving in that area (Poveda, 1998). Poveda, et al. (2014) also postulated the existence of the biotic pump mechanism in western Colombia following the Makarieva and Gorshkov hypothesis (Makarieva & Gorshkov, 2006; Makarieva & Gorshkov, 2010).
Wang (2002, 2004) analyzed the existence of a Walker cell in northern South America. His work describes the existence of an east-west circulation with rising air in the west (Northern South America) diverging to the east in the high troposphere to sink over the colder eastern Atlantic and return to the west, which is directly associated with diabatic heating and Hadley's circulation cell. Poveda & Mesa (1999) and Poveda, et al. (2006) also described the presence of strong easterly winds in the middle equatorial troposphere traveling like a jet from the Atlantic Ocean to the Andes.
The moisture advection associated with all these winds produces copious orographic precipitation in both flanks of the Andes, although more abundant in western Colombia. Where precipitation largely exceeds evaporation in specific regions, a significant amount of latent heat becomes available to influence the atmospheric circulation. Recently, Cruz (2017) considered the dynamic and thermodynamic structure of the atmosphere heating and its effects over Colombian climatology using the vertically-integrated diabatic heating from NCEP-NCAR and ERA-interim reanalysis. She assumed that the latent heating associated with condensation controls the longitudinal variation of the radiative fluxes (Webster, 1972). One of the most relevant results of Cruz's work is the annual cycle of vertically integrated diabatic heating. These observations confirmed that over northern South America, specifically in western Colombia, there is one of the most prominent regions of diabatic heating on Earth. These observations are also consistent with the dynamics of the outgoing longwave radiation in the region studied by Zhang, et al. (2017) and the analysis of the thermodynamic structure of the atmosphere from ERA-40 atlas reported by Chan & Nigam (2009). From mid-March to the end of November, this heat source is concentrated in a specific area and has a small size in comparison with the circulation around.
It is clear, then, that there is a sizeable diabatic heating source concentrated over western Colombia whose presence is due to the convergence of low-level moisture toward the Andes producing large amounts of orographic precipitation and the consequent large amount of latent heat release from the beginning of March until the end of November. On the west side of the heating source, there are two curvatures in the wind circulation responsible for strong westerly winds, one in the northwest branch associated with the Caribbean jet and the other in the southwest known as the CHOCÓ jet. Besides, there is a Walker cell on the east side of the heat source, which generates easterly winds. Figure 1 shows a conceptual scheme of the pathways in the low-level circulation as described in the literature; these pathways are similar to those proposed by Poveda, et al. (2014) and Arias, et al. (2015).
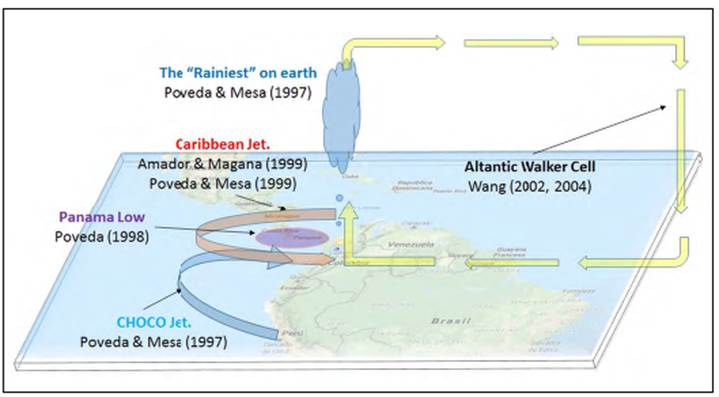
Figure 1 Schematic diagram of the general circulation of the atmosphere in northern South America and Mesomérica based on the review of relevant literature
Xie, et al. (2006) considered the strong westerly winds associated with the orographic precipitation and the high latent heat release. Also, Holton (2012) argued that the existence of diabatic heating sources can induce large-scale circulation. Some examples are the Walker cell and its variations (El Niño-Southern Oscillation), the monsoon circulation, the Madden-Julian Oscillation, and the equatorial waves (Zhang, et al., 2017). There are several models of this induced circulation. They are founded on the early work by Webster (1972) and Gill (1980) whose basis, in turn, comes from a still earlier original work on the linear equatorial wave theory (Matsuno, 1966). The main assumptions of those models are that: (i) heating associated with the condensation of water vapor in deep convection drives the surface winds, and (ii) linear dynamics is adequate to understand the surface wind response to this heating.
The qualitative results of this kind of model and the documented observations on the atmosphere's general circulation over northern South America are consistent enough to affirm that a set of equatorially trapped waves induced by a heating source explains the circulation around Colombia. Developing this hypothesis is an essential step for a better understanding of the Colombian hydroclimatology, as well as Northern South America and Mesoamérica circulation. The present paper is organized as follows: Section 2 revisits the rainiest place on Earth using diverse gauging stations in western Colombia; in section 3 we present the Matsuno -Webster Gill Model to propose a linear mechanism to explain how the diabatic heating due to intense precipitation determines surrounding circulation in response to exited equatorially trapped waves; section 4 discusses the model and how it describes the main features of the general atmosphere circulation around Colombia and compares the reanalysis with the simple model results; section 5 extends the linear model to some numerical experiments on the seasonal variability of the diabatic heating; finally, we make a brief summary and present our conclusions in section 6.
The rainiest place on Earth revisited
The town of Lloró, in the department of Chocó, Colombia, received 12,717 mm of average annual rainfall from 1952 to 1958 as registered in the Lloró La Granja station (Snow, 1976) while the nearby town of Vigía de Curvaradó received 26,871 mm in 1974 (Eslava, 1974). These records surpass or are very close to those in Cherrapunji's, India, and Mr. Waileale's, Hawaii, widely accepted as the rainiest locations on Earth (see Ahrens, 1998, p. 355), which led Poveda & Mesa (1998) to present Lloró as the rainiest locality on Earth.
Lloró La Granja station has records only for seven years and the gauge station is no longer working. Another station located to replace it only registered 8,240 mm of average annual rainfall during the period 1983-2018. Therefore, to select Colombia's rainiest recording gauge, we considered only active stations with sufficient years of registration. Table 1 presents the annual average precipitation (P), as well as the total maximum annual rainfall (Pmax) for some stations located in the low lands of the Colombian Pacific coast using the official data of the Colombian Institute of Hydrology, Meteorology, and Environmental Studies (IDEAM). We also present the first year of records (year) and the geographic coordinates of each station. The rainiest gauge in Colombia, and probably on Earth, is Puerto López (77°14'56.3"W, 2°50'43.0"N) in the department of Cauca with 13,159 mm/year. In the same town but in La Concha location, we have the second rainiest gauge with 12,069 mm/year while the rain gauge of San Antonio de Yurumanguí (77°15'33.3"W, 3°15'38.4"N), in the nearby city of Buenaventura, received 26,987 mm in 2018.
Table 1 Mean annual rainfall and maximum annual records at different gauging stations in western Colombia
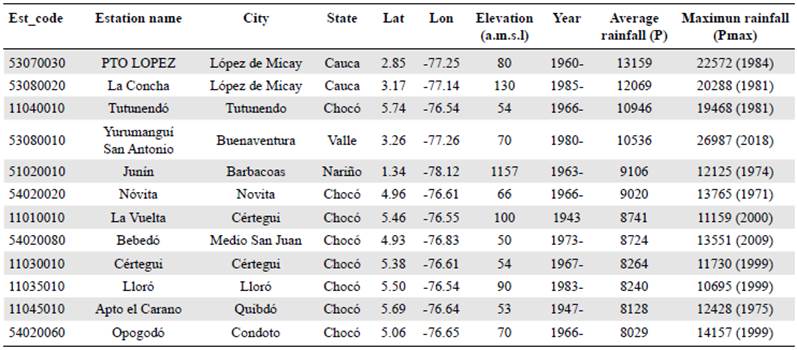
The rainiest place on Earth is not only a record; it is also a noteworthy hot spot in the tropical circulation. A large amount of rainfall also means a large amount of latent heat release. This heating source is originated in the convergence of low-level moisture toward the Andes. Condensation heating appears to be the primary energy source in the tropics as the diabatic heating associated with tropical precipitation not only drives a local response in the atmospheric circulation but also an excitation of equatorial waves which induce remote responses and large scale circulation (Holton & Hakim, 2012). For this reason, the three-dimensional structure of the diabatic heating closely relates to the atmospheric circulation because it not only drives the circulation, it also receives feedback from it.
The heat-induced circulation model
TheMatsuno-Webster-Gill (MWG) Model
Matsuno (1966) studied the linearized shallow water equations applied to an equatorial β-plane:
where u and v are the velocities in the x and y directions, respectively, and h is the small deviation of the elevation on the top surface, the mean value of which is denoted by H. f is the Coriolis parameter and g is the acceleration of gravity. As shown in figure S1,https://www.raccefyn.co/index.php/racceiyn/article/view/899/2837; the x-axis is along the equator directing eastward and the y-axis is northward.
Here f= βy, where y is the meridional length measured from y=0 at the equator and β=df/dy is the so-called Rossby parameter and is approximated to a constant. One can rewrite the set of equations using the geo-potential height ϕ instead of the geometrical height h.
where c 2 = gH which is the square of the velocity of pure gravity waves.
Webster (1972) developed a theoretical analysis of the large-scale, stationary, zonally asymmetric motions that result from heating and orographic effects in the tropical atmosphere. Then, Gill (1980) using also the Matsuno (1966) equations proposed a simple analytical model, which explains the main characteristics of the response of the atmosphere to concentrated diabatic heating in the tropics over a finite area. Both Webster and Gill found that there is a significant asymmetry in the circulation at the east and west sides of the heat source explained by solutions of the shallow waters equations in steady-state with a forcing equivalent to a concentrated heat source.
Suppose an atmosphere at rest is perturbed by adding an amount of heat Q and the perturbation is small enough to apply the linear theory, in that case, equatorially trapped waves describe well the response of the atmosphere. If the heating was switched on at some initial time, Kelvin waves would carry information rapidly to the east, thereby creating easterly winds in that region; also, winds will rise over the heat region generating a Walker-type circulation cell. If dissipative processes were significant, the easterly winds would reduce their speed gradually. On the other hand, switching on the heat source can also generate a half planetary wave (or half Rossby wave) which carries information quickly to the west. These planetary waves are much faster, three times as fast as the Kelvin waves, so the planetary wave size is one third of the Kelvin wave, resulting in two low-level cyclones with strong westerly winds.
When the movement occurs in the tropical region, reducing the problem to the non-dimensional form of a shallow water equations system with thermal forcing is useful. To that end, the units of time and length should be taken as:
where α is the equatorial Rossby radius and T is a length of time. The following equation is used to write the shallow water equations in a non-dimensional form introducing the non-dimensional quantifiers denoted by an asterisk (Gill & Clarke, 1974):
x* = x/a y* = y/a u* = u/c v* = v/c φ* = c 2 φ Q* = c 2 Q/T.
Finally, the non-dimensional equations (dropping the asterisk) are:
In these equations, the independent variables (x, y) represent the non-dimensional distances with x varying eastward and y along the north with y=0 at the equator. Both u and v are proportional to the components of horizontal velocity, and Φ is proportional to the geo-potential disturbance. Q is proportional to the heating rate and the signs imply that a positive Q represents warming. Variables u, v, Φ are measured on the surface. Equations [9] and [10] represent the momentum equations while [11] is the continuity.
Dissipative processes need to be introduced in some way to study the response to a stationary forcing. The most convenient ones are the "Rayleigh friction" and the "Newtonian cooling," in which the operator ∂/∂t is replaced by ∂/∂t + ε. An even more considerable mathematical simplification comes from assuming that ε adopts the same values in all the equations; this means that ε represents friction in the momentum equation and cooling in the continuity equation. Under steady flow with dissipation, the system of equations above becomes:
Assuming a small ε«1 and that the forcing function is such that on the y-axis perturbations have an order of magnitude close to one with wavelength k small, then 2εk«1. This relationship implies that the east-west forcing scale (k) is much larger than to 2ε. Therefore the term εv could be omitted. Then,
This simplification implies that the horizontal velocity u is in geostrophic balance with the pressure gradient, a simplification equivalent to the long wave approximation when analyzing the system of equations in the transitory regime. Finally, the set of equations of the MWG model are:
Besides, the vertical velocity w comes from the buoyancy equation:
The analytical solution of the MWG model for symmetrical heating to the equator
Assuming that the heat source is located symmetrically to the equator as shown in figure 2a, there is a neat analytical solution proposed by Gill in terms of parabolic cylindrical functions. Since the parabolic function D(y) = exp is symmetric to the equator, one can use it to define the symmetric heating source as:
In this case, the wave number k is
When n = 0, the atmospheric response to an asymmetric heat source to the equator generates a Kelvin wave that travels to the east as shown in the following solution:
Figure 2b shows Gill's solution for n = 0 and a heating source over the equator for L=2 and ε=0.1. The previous solution represents a Walker circulation to the east (x>L) with easterly winds (q 0 <0, u<0) which travel parallel to the equator towards the heat source (|x|≤ L) where the winds rise (w>0 with a maximum x=0) and then return towards the east in the high troposphere. The wave moves with unit celerity and dissipative rate ε. On the equator, there is always low pressure. However, it decreases towards the west until reaching its minimum on the heat source. The Kelvin wave does not carry information to the west of the heat source, so the solution for x<-L is zero.
When n = 1, the system of equations has a solution described by a half planetary wave to the west of the heat source whose equations are:
Figure 2c. shows the circulation to the west of the heat source described by the half planetary wave.
There are some important observations about the properties of this solution:
u = -
q 2 (x) when y=0 and q 2(x) <0for x<-L. Therefore, the winds are from the west over the equator on the west side of the heating source.
u=0 for y=±√3, which means that westerly winds vanish at a distance √3 above and below the equator. When y>√3 y and <-√3, the winds are from the east. In other words, winds recurve to the west of the heating source, as much to the north as to the south, generating two cyclones.
The above inferences imply that the air is descending everywhere in the region x<-L and is equatorward.
Pressure decreases from the west to the east and the flow over the equator follows the pressure differences. By thermal wind and pure baroclinic mode in the upper troposphere, the fluxes are easterly.
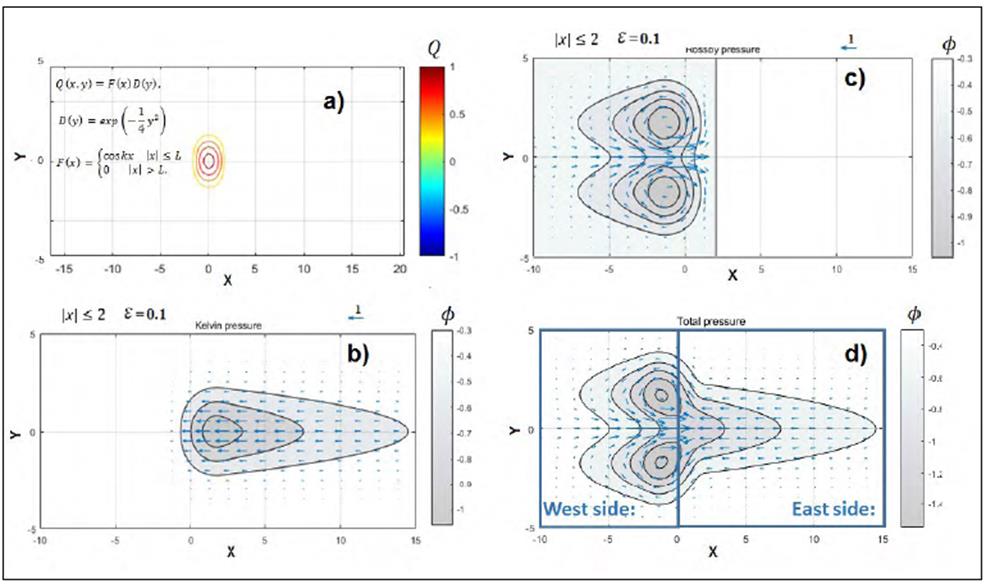
Figure 2 Solution for heating symmetric about the equator for decay factor ε = 0.1 and L=2 in the lower troposphere (Gill, 1980); contours show perturbation in pressure, which is negative everywhere. a) Heat located symmetrically to the equator. b) Gill's solution for n = 0 - Kelvin wave. c) Gill's solution for n = 1 - Rossby wave. d) Total MWG solution for the system response to a heat source symmetric to the equator
The system of governing equations in the MWG model is linear, so the principle of superposition of the solutions is applicable. The sum of the solutions associated with the equatorial Kelvin and Rossby waves generates the total flow in response to a constant heating source such as that found in the western portions of the tropical ocean basins. d shows the total MWG solution for the system response to a heat source symmetric to the equator for ε=0.1 and L=2. In the figure, the winds are westerlies on the west side of the heating source and easterlies on the east side. Notice that there is an ascending motion over the heating zone. Also, the shape of the rising air area is similar to that of the forcing region. There is subsidence outside the heating region, especially to the west. The pressure is negative on all sides with a minimum over the heat source. The pressure to the west of the forcing region is relatively high at points farther from the equator.
Examining the meridional flows over the heating region at the limit when ε tends to 0, it is evident that v=0. This implies that the flow is longitudinal with no meridional component east of the heating zone. For the Rossby wave to the west, we have:
Therefore, v decays exponentially away from the equator. However, perhaps the most exciting feature revealed by the above equation is that v>0 for y>0, which is a flow to the north while for y<0, v<0 the flow is to the south. These signs mean that the low-level flow is poleward out of the heating zone. Besides, w>0, so the conjunction between v with w components generates a flow over the heating source that is upward and outward creating a vortex tube.
The conceptual scheme in figure S2,https://www.raccefyn.co/index.php/raccefyn/article/view/899/2838; presents the MWG solution with easterly winds to the east side of the heat source and strong westerly winds to the west. There is a poleward divergence flow over the heating zone in the lower troposphere. Meteorologically, easterly and westerly winds transport cold air towards the heating zone and, then, the wind will return towards east/west in the high troposphere according to the thermal wind relationship. Strong easterly winds associated with two anticyclones in the upper troposphere (300 hPa) compensate the two cyclones associated with strong westerly winds in the low-level troposphere (850 hPa). Similarly, at the east side of the heat source, low-level easterly winds are balanced by westerly winds associated with weak cyclonic circulation in the upper troposphere. In light of these results, it is much simpler to understand the response of the upper troposphere to a heat source dynamically formed in lower levels.
The numerical model of tropical circulation in northern South America
a shows MWG's numerical solution for heating symmetrical to the equator located at 77°W scaled on a map of the Americas using a numerical approximation of central finite differences scheme to solve the linear system of equations under the steady-state conditions. One can notice a Walker circulation over the tropical Atlantic and the Orinoco and Amazon basins with easterly winds that travel parallel to the equator toward the heat source where the winds ascend (w>0 with maximum x=0) and then return to the Atlantic in the high troposphere. On the west side of the heat source, two cyclones generate strong westerly winds, one of them located to the north-west, which forces the flow to cross Central America recurving to northern South America and there is also another similar recurving from the Peruvian coast. Winds rise on the heat source by a vortex tube that directs the winds poleward in the high levels of the troposphere. On the upper troposphere, there are two anticyclones which generate westerly winds to the west of the heat source. All those features of the MWG model match with the observations in the literature review.
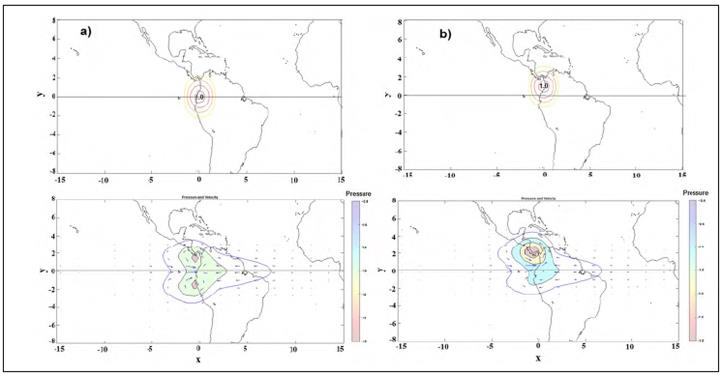
Figure 3 Low-level atmosphere’s general circulation over northern South America associated with: a) Left panel: A heat source symmetric to the equator located at 78°W using ε = 0.1 and L = 2. b) Right panel: a heat source located at 4°N using ε = 0.1 and L = 2
Using the MWG's solution we could integrate into a single physical framework all the previous analysis of the atmospheric circulation over northern South America. Among other things, this theoretical framework provides:
A new explanation for the Caribbean jet recurving (not explained until now).
A reinterpretation of the re-curvature of the winds coming from the Peruvian coasts (the so-called CHOCO Jet) using a new reason not related by Stenrud's mechanisms.
A connection between these two with the existence of a Walker cell between the tropical Atlantic and the north of South America.
The analysis of the annual diabatic heating cycle presented by Cruz (2017) shows that the heat source is usually at 4°- 5° N from mid-March to mid-November. Therefore, to have a more realistic condition of the circulation in northern South America, we should solve the problem associated with a heat source positioned to the north but close to the equator. We simulate asymmetric heating located at 4°-5°N represented by a meridional displacement of +0.9 in y in the non-dimensional model as presented in b.
The heat source is still close to the equator and many of the patterns observed in the symmetric forcing circulation are preserved, for example, the winds on the eastern flank remain almost intact preserving most of the features of Walker's cell with a slight reduction in the maximum value of the easterly winds. The most significant changes occur on the west side of the heating zone in the circulation associated with the planetary wave. Both cyclones are still present but that to the north-west of the heating source is strengthened by decreasing the pressure values in its center of action (decreases by 50%) while the cyclone to the south-west weakens due to an increase in pressure of 30%. These changes generate a slight increase in the magnitude of the westerly winds (5%) and a slight reduction in vertical velocity; an increase in the outflow from the heat source with preference to the north in the low-level troposphere can also be observed.
Scaling a map of the Americas on the numerical solution of the MWG model, it is possible to notice that the intense cyclone located north-west of the heat source should be positioned over Panamá creating the "Panamá Low" while the southwest has its center of action over the equator; both cyclones are responsible for generating strong westerly winds between 4° and 5°N. In the east of the heating zone, there are easterly winds from the tropical Atlantic crossing the north of the Amazon and the Orinoco basin before reaching the Andean mountain range and the diabatic heating source. Another interesting result is that the magnitude of the easterly winds located in the north of the cyclone on Panamá increases by 23%. This intensification would imply that the high velocities in low levels of the atmosphere associated with the Caribbean Jet from April to November could be also associated with the asymmetric location of the heating source above the equator.
Figure 4 shows a conceptual scheme of the general circulation of the atmosphere based on the results obtained for a heat source located north of the equator. The graph omits the existence of weak anticyclones. In contrast with the intense cyclone at a low level (the Panamá Low), there should be a strong anticyclone at high levels of the troposphere by thermal wind compensation (fist baroclinic mode). Besides, strong easterly winds in the upper troposphere compensate those from the west in the lower atmosphere. There is a Walker circulation with easterly winds in 850hPa and westerly winds over 300 hPa; also, there is a divergence of the low-level winds from the source of heat present towards the north, which must be compensated in the upper troposphere by winds towards the south.
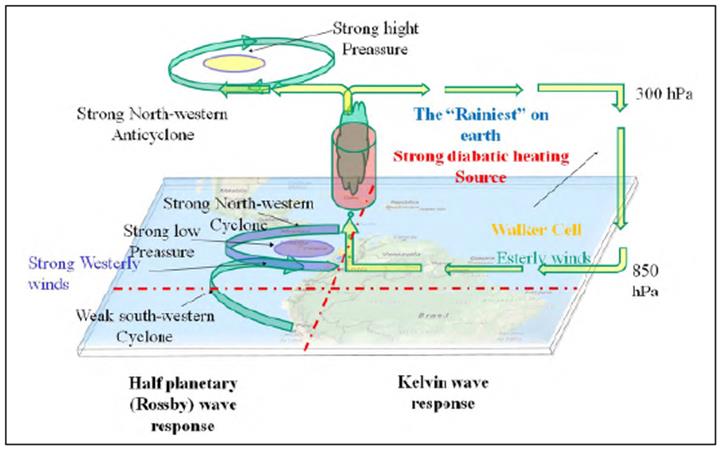
Figure 4 Schematic diagram of the general circulation of the atmosphere in northern South America and Mesoamerica using the heat-induced circulation
A review of the general circulation in the low (850hPa) and high (300 hPa) troposphere over northern South America confirms the previous conceptual scheme of the MWG circulation for which we draw the streamlines of the wind fields of the ERA-interim reanalysis. shows an intense cyclone over Panamá and a slightly weaker one on the equator; together those cyclones generate strong westerly winds on the western flank of the Andes. On the east side of the heating source, easterly winds go from the Atlantic to the eastern flank of the Andes mountains, most of them running parallel to the equator. In the upper troposphere, two anticyclonic circulations are evident, the first over Central America and the second over Bolivia (Bolivia High). The upper tropospheric anticyclone over Central America follows the conceptual scheme described in with strong easterly winds to the west of the heat source and winds from west to east.
The sensitivity of the MWG-type circulation to longitudinal and latitudinal heating or cooling sources
Figure S3, https://www.raccefyn.co/index.php/raccefyn/article/view/899/2839; presents the annual cycle of the total column-diabatic heating taken from the ERA-40 Atlas. For its estimation, all the components of the energy balance in the residual thermodynamic equation were considered; therefore, its values are higher than those presented by Cruz (2017). The idea was to determine the order of magnitude of diabatic heating (positive) or cooling (negative) sources around Colombia to simulate different scenarios.
Several studies have affirmed that the westerly winds on the Eastern Pacific arise from the sea surface temperature differences between Perú and Colombia's coasts (Poveda & Mesa, 1999, Poveda, et al., 2006; Poveda, et al., 2014). This argument follows Stensrud's (1996) mechanisms to explain the low-level jet but our analysis suggests the importance of considering the circulation's sensibility to other latitudinal cooling/heating zones.
This problem is similar to one developed by Gill (1980) when studying the response of the tropical atmosphere to an asymmetric forcing, i.e., a heat source north of the equator and cooling to the south. This scenario generates cross-equatorial winds that curve towards the heat source, like in the case of the Indian monsoon during the boreal summer. However, this analytical solution implies that there is no flow on the eastern side of the heat source and the response is limited to the west. If these arguments were correct, they would imply that a cold source over Perú with the same magnitude as the Colombian heat source would affect the easterly winds and Walker's cell but not the westerly winds. This question is just a theoretical introduction to a problem that needs further consideration.
We need the main features of the velocity and surface pressure fields to perform some basic numerical experiments. Let us denote Uw max as the maximum westerly wind speed; Ue max is the maximum easterly wind speed at the east of the heat source; Ue 2 corresponds to the maximum value of the easterly winds on the cyclone located north -west of the heat source; P 1 and P 2 are minimum values of the pressure of the cyclones located north-west and south-west of the heat source, and W max represents the maximum value of vertical velocity.
The diabatic heating fields presented by ERA-40 Atlas (Figure S3,https://www.raccefyn.co/index.php/raccefyn/article/view/899/2839) show that the magnitude of the heat source over Colombia is much higher than the cooling on the Peruvian coast with heating values higher than +800 W/m2 while the magnitude of the Pacific Ocean cooling in front of Perú is less than -150 W/m2. Being generous, the ratio between the two could be 1: -0.2. Figure 6 shows the results of the simulation of this scenario (case 1), and table 2 contains the numerical results. The magnitude of the westerly winds does not depend on the gradient of temperatures between the coasts of Colombia and Perú, but the Walker circulation would be a highly sensitive circulation if there were cooling sources near Perú because easterly winds reduce its magnitude in about 17% (from -0.96 to -0.80).
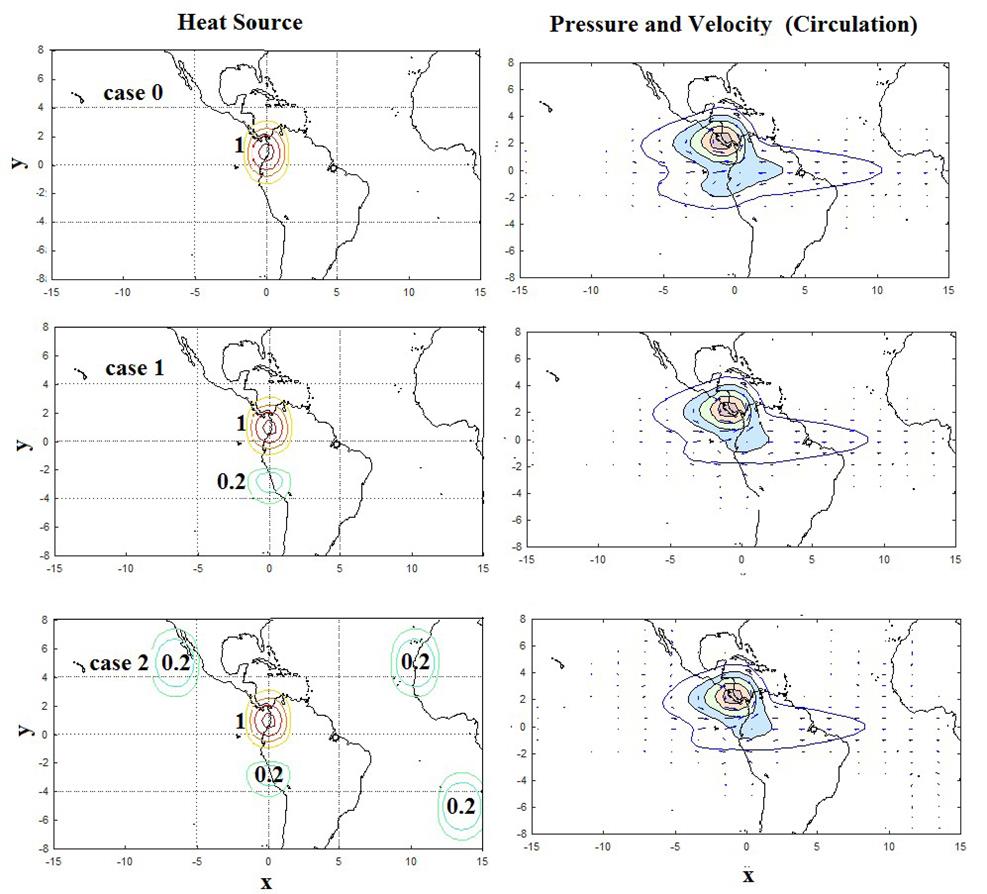
Figure 6 Comparisons of the circulation in northern South America for different cases: Diabatic heating source located at 4°N (Case 0); diabatic heating source located at 4°N and a cooling zone on Perú (12 ° S) with a 1: -0.2 relation (Case 1); diabatic heating source located at 4°N and cooling zones on the extra-tropical anticyclones (Case 2)
Table 2 Numerical experiments for cases: 1) Diabatic heating source located at 4 ° N and a cooling zone on Perú (12 ° S) with a 1: -0.2 relation and 2) diabatic heating source located at 4 ° N and cooling zones on the extra-tropical anticiclones

Although the Pacific Ocean in front of Perú is quite cold, this does not imply the existence of a concentrated cooling zone on this region; in fact, the existence of the Southeast Pacific Subtropical Anticyclone off the coast of Chile and close to the Atacama Desert suggest that this area is the correct location of the subsidence zone. Case (2) presents an analysis of sensitivity to the southern location of cold zones in the MWG model according to the magnitude and location along the extratropical anticyclones. The results show that the influence of extratropical cold zones upon the circulation in northern South America is almost nil.
Other analyses (not shown here) explain what happens when there is another heating source in front of the coasts of Perú. In that case, the westerly winds associated with the two anticyclones would be located south of the equator between the two warming zones generating intense precipitations on the western side of the Andes close to the Equator and Perú. The result is a decrease in the magnitude of the westerly winds entering Colombia and an increase of the speed of the easterly winds on the eastern flank of the Andes. These anomalies usually occur during a coastal El Niño event (Takahashi & Martínez, 2017).
Different macro-climatic events at different time scales can generate diabatic heating or cooling anomalies over the Pacific. The MWG model also helps to explain the response of the atmosphere to these scenarios. As an exercise, we proposed to locate other heat or cooling sources over the central Pacific in the same way and magnitude as that located over Colombia. These exercises are of particular interest because they resemble what happens during El Niño, La Niña, or other events on the Pacific.
Table 3 and figure 7 show the main parameters of the numerical experiments. The first observation is that the presence of an additional heating source over the central Pacific (case 3) produces a reduction in westerly winds of 25% from 2.44 to 1.84 while the easterly winds to the east of the original heat source increase by 50%. In this case, there is a weakening of the two anticyclones and a strengthening of the easterly winds on the eastern side of the Andes, which explains rainfall increase during El Niño events in those areas while the rest of Colombia is under dryer conditions. These observations were explained by Wang (2004) who demonstrated how the Walker cell over the Atlantic extends to the eastern Pacific under El Niño conditions due to the increase of easterly winds in the low and medium levels of the troposphere. Over the rest of Colombia, the vertical velocity is reduced by decreasing the buoyancy, facilitating the spatial acceleration of the wind in the middle troposphere levels, and generating a significant reduction in precipitation over the Andean region.
Table 3 Numerical experiments for another heating (case 3) or cooling (case 4) source over the tropical Pacific Ocean

Case 4 corresponds to the location of a cooling zone on the central Pacific Ocean; the effects are contrary to case 3. There is a 20% increase of the westerly winds and a 50% weakening of the easterly winds while the vertical velocity increases significantly creating convergence and precipitation.
Another interesting feature of Central America and northern South America clima tology is the existence of the Veranillo de San Juan or Canícula, also known as summer drought. This reduction of the precipitation during June, July, and August comes from "so far unexplained causes" (Poveda, et al., 2006). One possible explanation is the Eastern Pacific Warm Pool, which reaches maximum temperatures during the boreal summer (Magaña, et al., 1999; Wang, 2004; Xie, et al., 2005). The increment in the magnitude of the wind current in the Caribbean Jet could also play a role.
When analyzing the total vertically-integrated diabatic heating in the ERA 40 Atlas, one notes the development of another heating zone associated with the Eastern Pacific Warm Pool. Indirectly, the location of this additional heat source may have similar effects to those of case 3 in the previous analysis. The consequences are already known: the westerly winds entering Colombia from the Pacific reduce and the in easterly winds from the east increase. In fact, during the Andean summer drought, the eastern flank of the Andes presents the highest rainfall in response to the increase in easterly winds and the heat source could probably move towards the eastern Pacific reducing the ascent of air over Colombia. The strengthening of the easterly winds in the mid-level troposphere produces moisture divergence over the Colombian Andean region thus decreasing precipitation.
Conclusions
The heat-induced tropical circulation model proposed by Matsuno-Webster-Gill represents a physical explanation of the general circulation of the atmosphere in northern South America and Mesoamerica and changes the paradigm for understanding hydroclimatology in Colombia and its surroundings.
This new explanation of Colombia's hydroclimatology is remarkable because the physical principles of a heat-induced circulation could be applied to different time scales of the geophysical fluid dynamics (at daily, intra and inter-annual scales, and to climate change). On the other hand, the linear approximation is potent: it allows superposition exercises without limit, positive and negative sources, time dependence, and all can be constructed from linear solutions tables. Also, we can deconstruct the circulation to have a better understanding than the one presented here.
The heat source over western Colombia produces a strong low-level westerly inflow because a half planetary wave propagates over Mesoamerica and the far Eastern Pacific generating two cyclonic flows. This wave is an excellent feedback example between westerlies and latent heating on west-facing mountain slopes. According to this explanation, winds from the Caribbean Sea and the Peruvian coast recurve towards Colombia giving rise to the rainiest region on Earth. Since heating sources drive westerlies, this feedback process is inevitable. On the east side of the heating source, a Kelvin wave generates low-level easterly flow from the tropical Atlantic Ocean and the Northern Amazon and Orinoco basins in a Walker type circulation.
The asymmetrical location of the diabatic heating (4-5° N) increases the magnitude of the north-western cyclone and the low-level pressure over Panamá becomes stronger. For this reason, we can conclude that the asymmetric diabatic heating location forces the "Panama Low." With the thermal wind compensation and the first baroclinic mode, there is also a strong anticyclone in the upper atmosphere over Central America.
This type of circulation is highly sensitive to the appearance of other latitudinal or longitudinal sources of cooling or heating. In this sense, we can conclude that the magni tude of westerly winds does not depend on the gradient of temperatures between the coasts of Colombia and Perú. However, the Walker circulation is highly sensitive to the presence of a cooling source near Perú.
At seasonal scale, warming in the Pacific Ocean generates an acceleration of easterly winds leading to a reduction in the continental convection and increases the divergence in the easterly winds over some regions, which translates into a dry season with a significant reduction in precipitation. On the eastern flank of the Andes mountain range, the acceleration of the easterly winds implies greater orographic and low-level convergence, increasing the precipitation in the area. From the annual perspective, the position of heating or cooling zones to the west of the heat source, which according to the MWG model generates a response comparable to that of the north winds from South America, explains events such as El Niño or La Niña.
To summarize, the diabatic heating and its anomalies (both negative and positive) associated with the rainiest place on Earth are really the drivers of circulations over Colombia and its neighborhood with a pervasively forced flow (everywhere); the planetary and Kelvin waves are "characteristic" or "caustics" creating information pathways which, in turns, dominate the low lever an upper-level winds fields.