Introduction
Since the discovery of fullerenes in 1985 (Kroto, et al., 1985) and their subsequent mass production by graphite resistive heating (Krätschmer, et al., 1990), these compounds have been tested for several applications. The uses cover a vast range of fields, from nanotechnology (Ya. Vul, 2002) to human health (Castro, et al., 2017; Martínez, et al., 2016; Sandoval, et al., 2019), materials science (Teprovich, et al., 2019), and photovoltaics (Castro, et al., 2018; Collavini & Delgado, 2018), among others. A quick search of the word fullerene in the Scopus database resulted in more than 32,000 papers (Elsevier B.V., 2019) with an average of almost 1,000 documents per year since 1985.
C60 fullerene has become very popular thanks to its physical properties, among them, the ability to reversibly accept up to six electrons (Ohsawa & Saji, 1992; Xie, et al., 1992), its non-linear optical properties (Ma, et al., 2019; Wang, et al., 2018), and its superconductivity under specific conditions (Buntar & Weber, 1996). To exploit these properties, exohedral functionalization of C60 has become the "go-to"" method to make the processing of these structures into more complex materials easier, partly because fullerene adducts are more soluble in common organic solvents than their pristine counterparts. Synthetic modifications have also been used to modulate the physical properties of the fullerene framework to insert it in several materials such as polymers (Giacalone & Martín, 2006), metal complexes (Romero, et al., 2017a; Zhou, et al., 2006), coordination polymers (Chen, et al., 2015; Peng, et al., 2013, 2014), metal-organic frameworks (MOFs) (Minar, et al., 2015) and dendrimers (Nierengarten, et al., 2003), just to name a few.
Among the vast number of fullerene derivatives, the search for symmetrical and highly soluble-in-water adducts, as well as polar solvents, have attracted attention given their potential applications (Hirsch, et al., 1994; Lamparth & Hirsch, 1994; Richardson, et al., 2000). In particular, the octahedral addition of malonate addends creates a unique platform with a special arrangement and icosahedral symmetry suitable for the construction of different supramolecular frameworks. To obtain these specific patterns, many protocols have been reported: in 1997 Kräutler proposed a methodology based on the topo-selectivity addition of bulky aromatic groups (orthogonal transposition) (Schwenninger, et al., 1997). With this methodology, C60 is protected, functionalized, and deprotected allowing the controlled addition on the equatorial fullerene region. This strategy has allowed the preparation of symmetrical hexakis adducts. Furthermore, variations in the Bingel reaction by Hirsch & Vostrowsky (2001) and Li, et al. (2006) have also allowed the one-pot synthesis of hexakis malonate addends.
Fullerenes hexakis adducts are ideal building blocks for the construction of 2D or 3D MOFs (Kraft, et al., 2014, 2017; Kraft & Beuerle, 2016) and the remarkable water solubility of carboxylic derivatives has given way to a handful of applications in medicine (Dugan, et al., 1997). However, reports on the synthesis of these compounds lack a proper characterization of their electronic properties, which seems necessary to construct better and accurate systems. In this sense, here we report the preparation of an equatorial tetrakis dicarboxylic [60] fullerene and hexakis [60] fullerene derivatives, both soluble in water, using the Kräutler methodology. We also conducted a full electrochemical and UV-Vis characterization of these compounds to study the effect of the substituents and the symmetry of the fullerene adducts versus pristine C60.
Experimental section
General methods
Reagents for synthesis were purchased from Sigma Aldrich and were used without additional purification. FT-IR, NMR, and UV-Vis studies were recorded on a Shimadzu FTIR-8400 spectrophotometer, an NMR 400 MHz Bruker UltraShield™, and a Pharma Spec Shimadzu JASCO V-730 UV-VIS spectrophotometer, respectively.
Electrochemical measurements. All electrochemical measurements were recorded in an autolab PGSTAT302N potentiostat. We used a three-electrode system with a glassy carbon electrode (3 mm diameter) as a working electrode, a platinum wire as a counter electrode, and a silver wire as pseudo-reference. The samples were solubilized in tetra-hydrofuran (THF) degassed with nitrogen using 0.1M of (n-Bu4)NPF6 as supporting electrolyte and ferrocene as an internal standard at the end of the experiments.
Synthesis of the trans-l-bis(anthracene) adduct (3)
Compound 3 was obtained using a solid-state reaction methodology (Duarte-Ruiz, et al., 2001) mixing 100 mg (0.138 mmol) of C60 and 49.5 mg (0.278 mmol) of anthracene later sealed under vacuum in an ampoule. The solid was heated at 240 °C in an oven for 1 hour.
After cooling down, the product was treated with diethyl ether and ultrasound and centri-fuged to remove the unreacted anthracene. The solid was treated three times with 20 mL of CS2 and centrifuged to extract the unreacted C60. A light brown fine powder was obtained with a 60 % yield and its characterization matched the reported data (Duarte-Ruiz, et al., 2001).
Synthesis of the [2:4] hexakisadduct (4)
We functionalized 100 mg (0.093 mmol) of compound 3 through the Bingel reaction using diethyl bromomalonate (1.86 mmol) and 1,8-diazabicyclo[5.4.0]undec-7-ene (DBU) (1.86 mmol) in 20 mL CH2Cl2 under inert atmosphere. The reaction mixture was stirred for 24 hours, after which the same amounts of DBU and diethyl bromomalonate were added again and stirred for another 24 hours. The product was purified using column chromatography (silica-CH2Cl2). Compound 4 was obtained as a yellow solid in 95 % yield and its characterization matched the reported data (Schwenninger, et al., 1997).
Synthesis of the equatorial tetraadduct ester (5)
We obtained compound 5 using the Kraeutler procedure. Briefly, the retro-Diels-Alder reaction was accomplished by introducing compound 4 (100 mg) into a vacuum-sealed vial which was then heated at 195°C for 5 min. Afterward, the product was purified by column chromatography using CH2Cl2 as solvent resulting in the olive green tetraadduct 5 with a yield of 88% (69 mg) whose characterization matched the reported data (Cardullo, et al., 1996, 1997; Schwenninger, et al., 1997).
Synthesis of equatorial octacarboxylic tetraadduct (6)
Compound 6 was obtained using the Hirsch methodology (Lamparth, et al., 1995). For the hydrolysis of the ester group, compound 5 (60 mg) was treated with a twentyfold molar excess of NaH in 50 ml toluene for 3 hours at 60° C, the product was precipitated with methanol, washed with 2 M H2SO4 water, and then dried under vacuum at 60 oC for 12 hours to obtain the octacarboxylic acid [C60(C(COOH)2)4] 6 with a 95% yield.
Synthesis of equatorial dodecacarboxylic hexakisadduct (8)
Under similar conditions, we mixed 100 mg (0.074 mmol) of compound 5 with 38 μL (0.22 mmol) of diethyl bromomalonate and 33 μL of DBU in CH2Cl2. After 2 hours of reaction at room temperature, the equatorial hexaadduct 7 was purified using column chromatography (CH2Cl2) and obtained as a yellow powder with a yield of 90%. The ester was then hydrolyzed with NaH/toluene and methanol to produce compound 8 with a 90% yield using the methodology reported by Hirsch, et al. Compounds 7 and 8 were originally reported by Lamparth and Hirsch but the ester 7 was obtained by template activation using 9, 10-dimethylanthracene with a yield of 37% and HPLC. The synthesis was confirmed by comparing it with the literature data (Lamparth, et al., 1995).
Representative spectroscopic data for adducts
5: FT-IR (ATR, cm-1): 2976 (CH-stretch), 1739 (C=O carbonyl stretch), 1459 (C-O carbonyl asymmetric stretch), 1240 (C-C-O stretch), 1022 (O-C-C stretch). UV-Vis: λ max (nm): 289, 325, 350, 450,540, 645. 1H-NMR (500.2 MHz, CDCl3): δ = 1. 44 (t, J=7.1, 12H), 1.46 (t, J= 7.1 Hz, 12 H), 4.5 (q, J=7.1, (H), 4.52 (q, J=7.1, 8H).13C-NMR (125 MHz, CDCl3): δ = 164.15, 163.86, 145.44, 144.35, 144.24, 142.64, 142.45, 142.20, 140.87, 71.17, 69.98, 63.17, 63.12, 46.83, 44.28, 14.16, 14.14.
6: FT-IR (ATR, cm-1): 2969 (OH stretch), 1729 (C=O carbonyl stretch), 1466(C-O carbonyl asymmetric stretch), 1379 (OH stretch), 1240 (C-O stretch), 1036, 826 (flexion OH) . UV-Vis: λ max (nm): 261, 292, 501. 1H-NMR (500.2 MHz, d-MeOH): δ = 4.89 (s, 8 H); 13C-NMR (150.9 MHz, d-MeOH): δ = 167.27, 166.68, 146.75, 145.74, 145.41, 144.72, 144.30, 144.13, 142.30, 73.41, 72.40.
7: FT-IR (ATR, cm-1): 2962 (CH-stretch), 1715, 1474, 1255, 1029, 817. UV-Vis: λ max (nm): 280, 317, 334, 387, 626. 1H-NMR (600.2 MHz, CDCl3): δ = 4.31 (q, J=7.1, 24 H; CH2), 1.31 (t, J= 7.1 Hz, 36 H; CH3).13C-NMR (150.9 MHz, CDCl3): δ = 163.87, 145.85 (24 C), 141.20 (24 C), 69.16 (12 C), 62.89, 45.46, 14.11
8: FT-IR (ATR, cm-1): 1737, 1409, 1138, 715 UV-Vis: 1 λ max (nm): 278, 315, 333. 13C-NMR (100.13 MHz, D2O): δ =169.91 (12 C), 141.49 (24 C), 141.25 (24 C), 72.70 (12C), 47.30 (6C).
FT-IR (ATR, cm-1): 2971 (OH-stretch), 1730 (C=O carbonyl stretch), 1460 (C-O carbonyl asymmetric stretch), 1372 (stretching OH), 1243 (stretching C-O), 1036, 826 (flexion OH).
Results and discussion
The synthesis of the [60] fullerene derivatives was achieved following a protocol of protection-deprotection previously reported (Duarte-Ruiz, et al., 2009; Duarte-Ruiz, et al., 2001; Kräutler, et al., 2001; Ortiz, et al., 2009). The protocol can be divided into four steps: The first consisted of a Diels Alder coupling reaction between C60 fullerene and anthracene that was done in the solid-state at 240°C (Duarte-Ruiz, et al., 2008). Product 3 is formed by the regiospecific addition of two anthracene moieties in a trans-1 position. Thereafter, compound 3 was functionalized by cyclopropanation (Bingel reaction) with diethyl bromomalonate in CH2Cl2 (Schwenninger, et al., 1997). This reaction resulted in the highly regiospecific addition of four malonate groups in the equatorial positions of the fullerene (Scheme) to yield compound 4 in a 95% yield. The characterization matched the reported data (Schwenninger, et al., 1997).
The anthracene addition to the fullerene cage was thermolabile and could be detached from the fullerene adduct 4 with a retro Diels-Alder reaction to obtain the green-brownish compound 5. The tetrakisadduct 5 was hydrolyzed using NaH/methanol base as reported by Hirsch to produce compound 6 (Lamparth, et al., 1995). Dodecacarboxylic hexakisadduct 8 was prepared by a Bingel reaction of compound 5 using the same conditions used above. The result was the addition of two malonate esters at the trans-1 position that were later hydrolyzed using NaH/methanol to obtain compound 8 (Scheme). The synthesis was confirmed by comparing it with the literature data (Lamparth, et al., 1995).
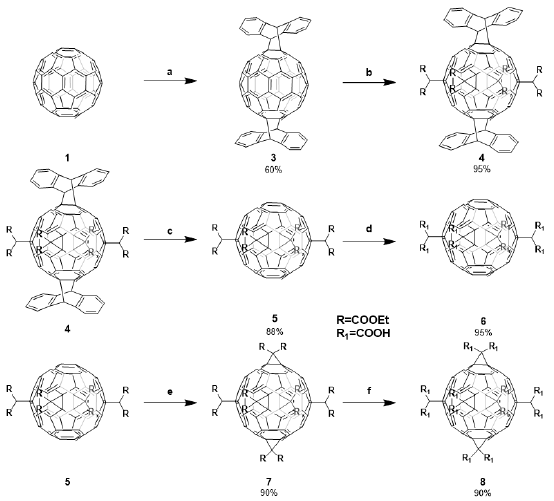
Scheme Synthesis of tetrahexakis Bingel adducts using a protection-deprotection protocol. a. Anthracene/240° C23 b. Diethylbromomalonate/DBU, CH2Cl2, 2 days.17 c. 195° C/5 min23 d. NaH/ Toluene-Methanol. e. Diethylbromomalonate/DBU, CH2Cl2, 2 hours. f. NaH/Toluene-Methanol.
Compound 7 has already been reported by Hirsch and coworkers via the successive addition of malonate addends (Hirsch,Lamparth, & Karfunkel, 1994; Hirsch, et al., 1994). However, the reported yields were in the range of 0.2 to 0.5 %, and the reaction times went as far as seven or more days. Furthermore, the reported process included the separation by HPLC, which is expensive and time-consuming. Later on, Hirsch, et al., reported a one-pot synthesis to obtain compound 7 using 9,10 DMA and a reversible template-directed activation with a 37 % yield (Lamparth, et al., 1995). Remarkably, our methodology allowed us to obtain compound 7 with an 86% yield starting from compound 5 as a unique product thus increasing the efficiency of the process and a yield of 38% considering the global synthesis. The preparation of these carboxylic fullerenes in high yields opens the way for their use in the construction of highly symmetric structures (MOFs and supramolecular and other structures related) and on different biological applications thanks to their increased solubility in aqueous media (Ugan, et al., 1997).
Figure 1 shows the IR spectra for compounds 5 to 8, i.e., the equatorial tetraadduct, the hexadduct with malonyl groups, the equatorial tetraadduct, and the hexadduct with carboxyl groups. Observe the change given the hydrolysis suffered by the ester group and the subsequent appearance of a broad and pronounced band of around 2969 cm-1 due to the -OH stretching, and of 1466 cm-1 due to the C = O stretching. In the case of the esters, the three characteristic peaks of these functional groups of 1700, 1200, and 1100 cm-1 approximately are observed. The three vibrations involved are the C = O, the C-C-O, and the O-C-C stretching.
The thermogravimetric analysis of compounds 5 to 8 can be observed in figure 2. In general, the adducts with the ester groups (compounds 5 and 7) exhibited thermal stability up to around 350 °C while we observed that the adducts with carboxylic groups (compounds 6 and 8) had a loss of weight starting at around 100 °C probably due to the presence of water molecules associated with the carboxylic groups of the tetraadduct with 8 carboxylic groups and of the hexadduct with 12 carboxylic groups.
Given that compound 6 is reported here for the first time, figure 3 shows the 13C-NMR spectrum in methanol for the equatorial tetraadduct with the carboxylic groups (compound 6). At around 166 ppm, signals due to the carbons of the carbonyl group are observed; at around 144 ppm, those due to the cage of fullerene, and near 72 ppm, those due to the carbons of fullerene that make up the cyclopropane rings.
Electronic characterization
The spherical π system present in the fullerene cages makes UV-Vis spectroscopy a versatile tool for characterization. It has been reported that changes in the UV-Vis spectrum of different fullerene derivatives depend on the number of substituents present in the cage, the type of addition, and the geometry of the adduct (Djojo, et al., 1996; Kop, et al., 2015; Lu, et al., 1996). Moreover, some specific absorption can be used as a fingerprint for the identification of specific substitutions in the fullerene, although the nature of the specific transitions is still unknown.
Adduct 3 exhibited two anthracene moieties, the D2;¡-symmetric bisadduct presented a fingerprint weak maximum at 465 nm (Figure 4), and broader bands in the visible region at 500 nm as previously reported by Duarte-Ruiz, et al. (2001) and Kraütler, et al. (2001). The change in the UV-Vis spectra compared to pristine C60 responds to the combination of two phenomena: first, the loss of the double bond conjugation that leads to isolated benzenoid rings, and second, the change of the symmetry group.
Adducts 4, 5, 7, and 8 (Figure 5,Table 1) presented an absorption band in the UV region at the range between 270 and 293 nm related to π- π* transitions whose different wavelength changes depend on structural features like the number of addends or even the type of substituent. For example, compound 4 exhibited a maximum band at 293 nm that hypsochromically shifted with the cleavage of the anthracene moiety (5-287 nm, 7-281 nm, 8-278 nm). A hypothesis on the loss of the anthracene π-system to explain this phenomenon is possible, but more experiments are needed.
Table 1 UV-Vis data collected from the UV-Vis spectra of the different prepared fullerene adducts
Compound | Absorbance bands (nm) | Fingerprint of absorbance bands (nm) | ε (cm-1 M-1) * | ∆E (eV)*** |
3 | 407, | 465, 500**, | 1.99 | |
4 | 291, 310**, 355, | 625 | 20150.38 | 2.88 |
5 | 289**, 322, 348, 454, | 623 | 20071.70 | 2.35 |
6 | 258, 289** | 623 | 20259.51 | 2.62 |
7 | 279**, 319, 338, 383, | 480 | 19978.45 | 2.91 |
8 | 283**, 312, 335 | 480 | 19558.05 | 2.92 |
* Calculated from the highest absorbance band; ** Band with the highest absorbance; *** Calculated as first oxidation potential-first reduction potential
The fingerprint region, which consists of the late UV and the visible region, can be used as stated above to identify different substitution patterns on a fullerene. Tetrakis adduct 5 exhibited a broad band going from 440 nm to 550 nm, which may be explained by the specific functionalization resulting in two benzenoid rings with localized electronic sextets (Cardullo, et al., 1997). Hexakis adducts 4, 7, and 8 displayed a lighter yellow color in solution while their UV-Vis spectrum in CH2Cl2 showed little absorption at the visible region and ended at 450 nm. The octahedral addition to the fullerene cage generated four isolated benzenoid rings in a cyclophane structure resulting in a large decrease in the chromophore π system conjugation.
Table 1 shows the effects previously explained. The optical band gap is a hint of the HOMO (Highest Occupied Molecular Orbital)-LUMO (Lowest Occupied Molecular Orbital) band gap. From these data, it is clear that the loss of sp2 carbons was the largest phenomenon affecting the absorption of the fullerene compounds and that there was not much difference between the esters and the carboxylic acids in terms of the bandgap. Finally, it is apparent that the addition of anthracene moieties increased the energy between the HOMO and the LUMO, although the increase was less than in fullerenes bearing just malonate substituents. These phenomena could be attributed to the benzenoid rings resulting from the Diels Alder addition, as it could help to stabilize the excited state.
Electrochemical characterization
Cyclic voltammetry experiments were conducted in THF with NBu4PF6 as supporting electrolyte using a 3 mm diameter glassy carbon disk as the working electrode, a silver wire as the pseudo-reference electrode, and a platinum wire as the counter electrode. At the end of each measurement, a small amount of ferrocene was added as an internal standard to report the potentials.
Considering the electronic effects shown in the absorption spectra, we expected that the number of substituents and the geometry of the fullerene adduct would affect the electrochemical properties of the C60 cage (Boudon, et al., 1995). To analyze such effects, we recorded the cyclic voltammetry of the different derivatives in THF (Figure 6andtable 2). In this sense, it is important to emphasize that there are few reports of electrochemical studies of fullerene addends in polar solvents (Paolucci, et al., 1999; Romero, et al., 2017b) and that the addition of the different malonates to the fullerene cage structure is a good methodology to increase the solubility of these compounds.
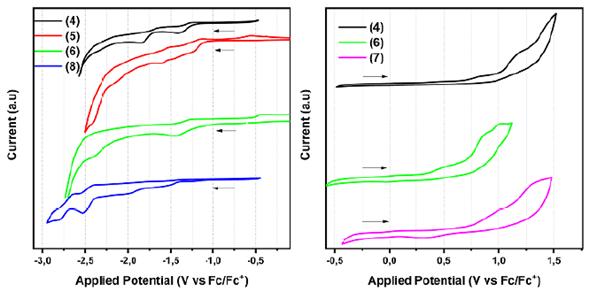
Figure 6 Cyclic voltammograms of adducts 4, 5, 6, 7, 8 in THF. Supporting electrolyte: 0.1M (n-Bu4N)PF6 (arrows indicate the direction and the start point of the sweep). The graph on the left shows the reduction events of compounds 4, 5, 6, and 8. On the right, the oxidation processes of compounds 4, 6, and 7 are plotted.
Fullerene has been considered for several electronic applications thanks to its remarkable ability to accept electrons. Different studies have shown that pristine fullerene can reversibly accept from three up to six electrons under certain experimental conditions, for instance, low temperatures (Xie, et al., 1992). Figure 6 shows the effect of the malonate addition on the electron affinity of the prepared compounds. Hexakis compound 4 showed two reversible reduction peaks at -1.43 V and -1.85 V, which were cathodically shifted when compared with the first two reductions of pristine C60. This decrease of the electron affinity of the fullerene cage was attributed to the loss of the conjugated π-system, which is necessary for stabilizing reduced species.
The reversibility of the reduction processes for compound 4 was also affected. As mentioned before, pristine fullerene reductions are reversible, however, the reduction peaks in 4 exhibited a peak current ratio different from one (higher cathodic current than the anodic current), and the peaks were separated from each other when the voltammograms were recorded at higher scan rates. The modification of the conjugated π-system affects not only the electron affinity of the cage but also the reversibility of these reduction processes, probably because, after reduction, a chemical process that affects the reverse current intensity occurs.
The number of additions to the fullerene cage had a direct effect on the electron affinity. Compared to compound 4, the tetrakis adducts 5 and 6 exhibited larger electron affinities, and their peak current ratios were closer to one. It has been proved that the addition of substituents to the fullerene cage increases the LUMO energy (Zhang, et al., 2006), an effect that is closely related to the extra energy necessary to transfer an electron from the working electrode to the fullerene compound.
The difference in the reduction potentials could be the result of the nature of the substituents. In the case of the hexakis compounds 4 and 8, the latter exhibited cathodically-shifted reduction potentials. It is possible that the presence of the benzenoid rings in 4 (anthracene addition) helped to stabilize the negative charge in the fullerene cage. Furthermore, carboxylic acids, present in 8, are far more reactive and tend to form dimers based on hydrogen bonds, which could explain why these compounds presented more reduction processes than their former esters in solution and why they exhibited two non-reversible processes.
Oxidation events are also related to the fullerene cage. It has been proposed that the electrons transferred from the fullerene cage to the electrode are part of the benzenoid rings generated with the addition of substituents to the fullerene (Cardullo, et al., 1997; Francesca Cardullo et al., 1996), a behavior that explains the little or non-difference in the oxidation potentials of the prepared compounds. These results (Table 2) are also consistent with those reported by Boudon, et al (1995) regarding the presence of an oxidation peak between 0.80 to 0.99 V in adducts with 1 to 6 addends.
For a better understanding of the electrochemical behavior of these compounds, cyclic voltammetry was recorded at different scan rates and, then, using the Randles-Sevcik equation (Equation 1), we graphed the relation between the peak current and the square root of the scan rate. From the results shown in figure 7, it is clear that the relation between the sweeping rate and the current was linear (Table 3), which means that the current was limited by the diffusion of species from the solution to the electrode and their speed towards the electrode (diffusion coefficient). The Nernst equation demonstrates that the increase in potential will also increase the movement of the species and the diffusion to the electrode thus increasing the current, which means that these electrochemical events are diffusion-controlled.
Equation 1. Randles-Sevcik equation
Conclusions
Anthracene can be used as a template to control the stereospecific addition of malonates in the equatorial region of the fullerene cage. Furthermore, the methodology we describe is an easy and controlled method for the synthesis of the highly symmetric and water-soluble carboxylic fullerene adducts (6 and 8) without using expensive and complicated techniques such as the HPLC. Cyclic voltammograms of the different adducts showed that the first reduction potential cathodically shifted with the increase of the number of addends attached to the fullerene cage in agreement with several reports of different fullerene compounds in the literature. Besides, the effect of addends was also related to the chemical nature of the substituents present in the fullerene. As we have shown here, there is a marked difference between the cyclic voltammograms of hexakis compounds 4, 7, and 8.