Introduction
The rise of the Isthmus of Panama, commonly known as the Great American Biotic Interchange (GABI), connected North with South America and facilitated the exchange of faunas (Simpson, 1983). The highest peak in mammal migration rates occurred, however, one million years later compared to the complete formation of the land bridge itself (Bacon et al., 2016; Bacon et al., 2015; Carrillo et al., 2020; Carrillo et al, 2015; Jaramillo, 2018; Montes et al., 2015; Webb, 1978; Woodburne, 2010). Why is there a migration delay? Contrasting biomes can constitute major barriers for fauna to migrate (Vrba, 1992). Therefore, biome interchange due to the onset of the Northern Hemisphere Glaciation and concomitant reductions in precipitation across the Americas has been proposed as a leading driver in the acceleration of GABI (Bacon et al, 2016; Bacon et al, 2013; Carrillo et al, 2020; Leigh et al, 2014; Molnar, 2008; Smith et al., 2012; Webb, 1976, 1978, 2006). This hypothesis, however, has not been fully tested yet. What we know about GABI comes mostly from sites in temperate latitudes in North and South America. In contrast, there is scarce empirical information about biomes and their mammal record during the Plio-Pleistocene of Central America and northwestern South America (Colombia), the main and probably only corridor through which terrestrial migrations occurred.
A member of our team, Gheny Krigsfeld Shuster, discovered and has explored for the past three decades a proboscidean (e.g., elephants and their extinct relatives) fauna in deposits at the bottom of the Cauca River in the Cauca Valley (CV) near Cali (Figure 1). A detailed study of the sediments containing this fossil assemblage is crucial to understanding them in a proper spatial and temporal framework and the fauna can be useful to further understand the Pleistocene in the Neotropics. In this sense, a first study has identified the presence of Notiomastodon platenensis (Pelegrin et al, 2022).
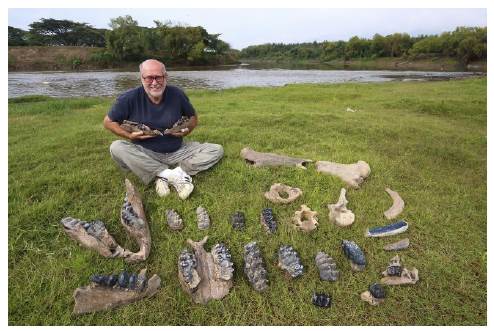
Figure 1 A. G.K. Shuster with some of the specimens he has collected over the past 25 years in the Cauca River (Photos by C. Ziegler)
Modern CV vegetation is heavily modified and less than 3% of the original forest remains in place (Pizano et al., 2014) (Figures S1A,S1B,https://www.raccefyn.co/index.php/raccefyn/article/view/1666/3225). A tree-dominated dry forest seems to have been the dominant biome in the CV during the Holocene (Berrio et al, 2002) with no sign of extensive savannas. Was the CV much drier and dominated by savanna during the Late Pleistocene? Climate and habitat models suggest that the CV was a dry corridor during the Last Glacial Maximum (LGM) (23,000 to 19,000 years ago) (Marchant et al, 2004) as precipitation and temperature dropped significantly in central and northern South America during glacial and last deglacial times (Escobar et al, 2012; Hodell et al, 2008; Hooghiemstra & Van der Hammen, 1998) but was it dry enough for savannas to replace the dry forest?
Here we present a geophysical study of the CV proboscidean deposits to assess their geometry and lateral extent, as well as a depositional time frame for the proboscidean deposits and a floristic reconstruction of the surrounding area at the time of deposition.
Methods
The proboscidean-bearing deposits are not outcropping and they can only be found by digging underneath the main channel of the Cauca River (Figure S1B,https://www.raccefyn.co/index.php/raccefyn/article/view/1666/3225). For decades, sand and gravel have been extracted from the bed of the Cauca River as a mining product used in construction and building infrastructure in the region, an operation done mostly by small-scale dredges (Figures S1C,S1D,https://www.raccefyn.co/index.php/raccefyn/article/view/1666/3225). The material mined by the community in the context of dredging operations in Platanares and the Andrés family in Paso Las Torres was used for our sedimentological analysis, radiocarbon dating, and palynology (Figures S1C-S1F,https://www.raccefyn.co/index.php/raccefyn/article/view/1666/3225 and figure 2).
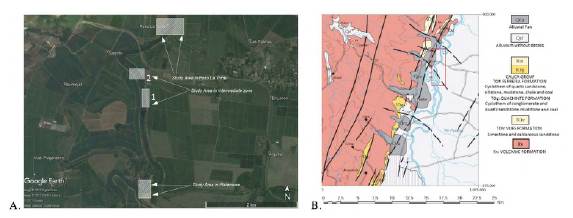
Figure 2 A. Geographical location of the survey areas. Platanares and Paso La Torre are the main areas while the two intermediate areas were surveyed to investigate spatial continuity (Modified from Landsat/Copernicus, 2017). B. Geological map of the survey area. The red rectangle indicates the area where the seismic and ERT lines were acquired (Modified from Nivia Guevara, INGEOMINAS, 2001)
We used seismic refraction and electrical resistivity tomography (ERT) to map the presence, depth, thickness, and geometry of a fossil-rich conglomerate layer under and adjacent to the Cauca River main channel in the two dredging sites. Our surveys included the fossiliferous layer at the two sites, as well as two intermediate points to investigate the possible spatial continuity and connectivity of the fossil-rich layer between the two sites. Seismic refraction is based on the propagation and refraction of artificially induced seismic waves (Redpath, 1973) (Figure S2,https://www.raccefyn.co/index.php/raccefyn/article/view/1666/3225). The method is performed on shallow targets by generating a seismic shock (simulating a micro-earthquake) and impacting the ground with a massive object such as a sledgehammer and recording direct and refracted waves at the surface with specialized seismic receivers known as geophones. We used a multi-channel (16 channel) digital seismograph with geophones evenly distributed along ~100 m straight survey lines (Table S1,https://www.raccefyn.co/index.php/raccefyn/article/view/1666/3225). Electrical resistivity tomography (ERT) determines the electrical resistivity of the ground and subsurface based on Ohm Law's rendering 2- or 3-dimensional models of resistivity distribution in the subsurface. The technique was used to provide seamless top-to-base images of the fossiliferous conglomerate and surrounding materials. We used a GeoAmp303 DC resistivity console, an ASM001 switch, and a 32-electrode digital tomography system (Table S1).
For the 'marine' seismic refraction (i.e., in the river), we placed two individual geophones on the riverbanks on opposite sides and created seismic waves by manually impacting the river bottom with a custom-made steel pole used as a marine seismic source. The pole was manually forced down onto the bottom from a boat to generate single shots and was designed to be expandable between 3 and 12-m long by adding consecutive pipe segments. The three points of each survey line -source and two receivers- were chosen collinear to ensure in-plane information only. Shots were kept at 5-meter intervals along the survey line. The source-receiver offset was designed to be 5 to 65 m. Due to the source-receiver symmetry of the seismic refraction technique, placing a geophone at point A on dry land and shooting at point B on the river bottom is equivalent to placing a geophone at B and shooting at A (Redpath, 1973). The result of this exercise was not successful as only the five channels closest to each shot point contained usable information (Figure S3,https://www.raccefyn.co/index.php/raccefyn/article/view/1666/3225). Bottom currents made it difficult to manually generate clean, strong shots on the bottom. In retrospect, our seismic source may have been too light as it was easily deflected in the downstream direction by strong bottom currents. Additionally, the turbulent stream appeared to impose additional seismic noise, which was not mitigated even by stacking numerous shots (Figure S3,https://www.raccefyn.co/index.php/raccefyn/article/view/1666/3225).
We used a standard multichannel acquisition for land seismic refraction. Sixteen live channels were placed along 120-m-long lines shooting at both endpoints and at three other intermediate locations. Geophones were planted every 8 m on line coordinates 0 through 120 m. Shots were 'fired' at line coordinates -8 m, 36 m, 84 m, and 128 m. An 18-pound sledgehammer was used as the land seismic source. In both marine and land seismic refraction work, the signal-to-noise ratio was increased by means of stacking records from multiple data gathers (9 to 11) recorded at each shot-receiver arrangement (Redpath, 1973). Seismic refraction proved very efficient to map the top of the conglomerate (Figure S3,https://www.raccefyn.co/index.php/raccefyn/article/view/1666/3225).
ERT survey lines were designed to be parallel or perpendicular to the river channel; intersect seismic lines when possible and provide extended spatial coverage (Figure S4,https://www.raccefyn.co/index.php/raccefyn/article/view/1666/3225). Original designs had to be slightly modified in the field due to operational limitations imposed by adjacent sugar cane farms and steep slopes at the riverbanks. Marine ERT implied additional field considerations relative to land ERT as lines had to be designed as parallel as possible to the stream flow direction to avoid cable bending and potential rupture. Besides, ERT survey lines were positioned so that the acquisition consoles remained on dry land during data recording to circumvent equipment exposure and mitigate electrical hazards.
A total of 23 seismic refraction and seven ERT lines were acquired (Table S2). Ground elevation values derived from high-resolution topography data provided by light detection and ranging (LIDAR) (Corporación Autónoma Regional del Valle del Cauca - CVC, 2016) were integrated to all models and profile sections. Our model was adjusted for LIDAR topography and, thus, z coordinates are referred to as mean sea level.
We analyzed the conglomerate samples collected from the dredge by clast counting using a hand lens. Clasts were counted using the area method (Howard, 1993) where the counted area should be at least 2.5 times the long axis diameter of the largest clast (in this case, 2.5 cm), and a lower clast size limit should be defined. Here, we counted all the clasts above 0.5 cm. We defined six classes before the counting by identifying the main compositional groups in all the samples; 150 grains were counted. The six classes defined for counting were: chert, aphanitic and porphyritic mafic igneous clasts, intermediate phaneritic igneous clasts, schists, vein quartz, and mafic phaneritic clasts.
Three wood samples taken from the conglomerates were sent for carbon 14 dating at the International Chemical Analysis (ICA) company (Miami, FL). Calibrated ages were attained using INTCAL13 (Reimer et al., 2013) and a 2-sigma calibration (95% probability). Conventional ages were rendered in BP (BP: Before Present, 1950 AD) and were corrected for fractionation using the delta C13. We treated samples with acid/base/ acid pretreatment (AAA) prior to graphitization and sent three bone samples for dating.
The palynological content of two samples was analyzed to assess the vegetation in the region. The first sample was an intraclast within the conglomerate and the second was collected from a thin, muddy layer capping the conglomerate. Both samples were processed using the standard acetolysis method at the Instituto de Investigaciones en Estratigrafía-IIES laboratories at Universidad de Caldas, Manizales (Faegri & Iversen, 1989) and mounted in glycerin gelatin slides for their examination through a Leica microscope with 100x magnification. The palynomorphs were identified following the morphological descriptions by Roubik & Moreno (1991), Hooghiemstra (1984), the Morphological Electronic Database (Jaramillo & Rueda, 2021), and the Alan Graham collection stored at the Smithsonian Tropical Research Institute in Panama (Moreno et al, 2014). Frequency was expressed as relative abundance excluding spores, aquatic groups, reworked material, and overrepresented taxa from the total pollen sum. When needed, references to the excluded groups were expressed in terms of the proportion of the total pollen sum. The diversity and richness of each assemblage were calculated using the Vegan package (Oksanen et al, 2010; R-Development-Core-Team, 2019). For comparative purposes, the richness was rarefied to the lesser pollen sum following Hurlbert (1971) but the diversity index (Shannon) was estimated from the total community excluding only the reworked material. The palynological content of muddy samples was compared with the palynological assemblages previously recorded in the Cauca Valley at the Quilichao-1 core (Berrio et al, 2002) to determine whether the sample belonged to the same sedimentary sequence. The comparison was done using detrended correspondence analysis (DCA) (Hill & Gauch, 1980) to summarize the variability of the assemblages within a dimensionally reduced space. Aquatic taxa and fungi spores reflect the immediate lacustrine settings better than the general environmental conditions and, therefore, they were excluded from the analysis. Pollen and spore counts were transformed into relative abundance percentages to perform the DCA. The similitude between the muddy sample and the Quilichao's assemblages was estimated from the site scores resulting from the DCA ordination using two approaches: first, gathering the samples based on CONISS clustering analysis and, second, estimating the Euclidean distance between the muddy sample and the Quilichao-1 sequence. All the analyses were performed using R software (R-Development-Core-Team, 2019), the Vegan (Oksanen et al., 2010) and the Rioja (Juggins, 2015) packages for the DCA, and the CONISS clustering analysis, respectively (supplementary information S1). The Quilichao -1 data (Berrio et al., 2002) was available through the Neotoma package (Goring et al, 2019).
Results
Geometry of the deposit
A strong seismic velocity contrast was evident in the seismic data between the modern river terraces, the sediment transported by the river, and the fossil-bearing conglomerate underneath. The seismic refraction data indicated that P-waves traveled through the conglomerate significantly faster (at nearly twice the velocity) than waves traveling through the overlying topsoil (Table S3,https://www.raccefyn.co/index.php/raccefyn/article/view/1666/3225). There was an overall south-to-north increase in seismic velocities consistent with an increase in the DC electrical resistivity. We performed ground control of our seismic data at Platanares, Intermediate Area 2, and Paso La Torre, where dredging data confirmed the presence and top depth of the fossiliferous conglomerate as predicted by the geophysical survey. The two-dimensional ERT images also indicated a significant difference in resistivity between the conglomerate and adjacent materials (Figures S5,S6,S7,https://www.raccefyn.co/index.php/raccefyn/article/view/1666/3225).
The LIDAR data showed the river water surface is 937.5 meters above sea level (masl) with slight variations (+/- 0.1 m) probably caused by small waves. The adjacent terrace stands at a mean elevation of 942.75 masl. The integration of ten seismic refraction lines and four ERT lines indicated that at Platanares, the top of the conglomerate is a buried, continuous, and uneven surface at 923 to 927 masl which floors the Cauca River (Figure 3). The thickness of the conglomerate layer ranges from ~15 to ~44 meters and has a lenticular geometry (Figures S5,S6,S7,https://www.raccefyn.co/index.php/raccefyn/article/view/1666/3225). The conglomerate top appears to run NNE-SSW dipping towards the SE (Figure 3).
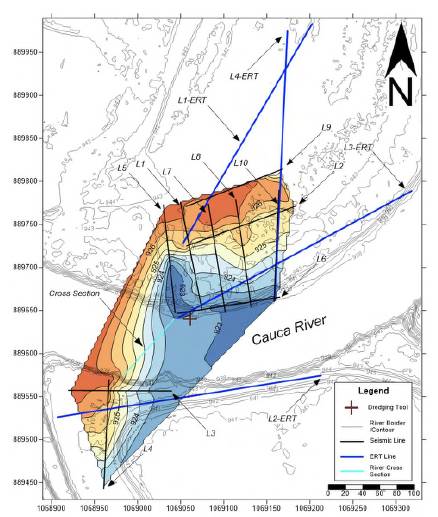
Figure 3 Subsurface elevation model in meters of the top of the fossil-bearing conglomerate in the Platanares area. Coordinates in Magna Sirgas Colombia West Zone
Intermediate Areas 1 and 2 show a lenticular geometry similar to that in Platanares (Figures S9,S10,S11,https://www.raccefyn.co/index.php/raccefyn/article/view/1666/3225). Paso La Torre imaging clearly showed the base of the conglomerate ranging from 14 to 29 m thick (Figures 4,5) and a lenticular geometry (Figures 4,S12,https://www.raccefyn.co/index.php/raccefyn/article/view/1666/3225). The intermediate Area 1, about 1.8 km north of Platanares, was imaged by two seismic refraction lines and one ERT line, all acquired on the eastern river terrace. The ERT line from this area provided strong geophysical evidence of the conglomerate presence and depth with a notoriously high resistivity dominating the image below ~920 m. The sinuous upper face of the high anomaly, where the top of the conglomerate was interpreted based on the strong resistivity contrast, matched the depth of the high-velocity layer in seismic refraction data and the conglomerate depth known at Platanares (Figure S8,https://www.raccefyn.co/index.php/raccefyn/article/view/1666/3225). The base of the conglomerate was not well imaged by this line. While structural contours drawn at the conglomerate top may suffer from spurious edge effects induced during interpolation, the map reflects the rough local conglomerate structure including two summits standing at 926 meters and an internal relief of ~ 7 meters (Figure S9,https://www.raccefyn.co/index.php/raccefyn/article/view/1666/3225).
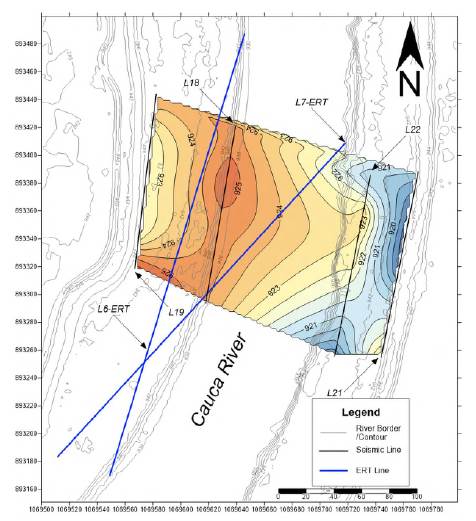
Figure 5 Subsurface model of the fossil-rich layer top at Paso La Torre. Coordinates in Magna Sirgas Colombia West Zone
The intermediate Area 2 represents the second infill area surveyed with the purpose of understanding and mapping the possible fossilifrous rock continuity between Platanares and Paso La Torre. This area lies some 400 m north of intermediate Area 1 and ~ 1 km south of Paso La Torre. Four shore-parallel land seismic refraction lines were surveyed there, two on each river terrace. Additionally, using a manual measurement made with the dredge tool 280 meters NW of this area we estimated the fossiliferous conglomerate top (at river bottom) 17.5 meters below the terrace level. A shot record from seismic Line 14 acquired on the western river terrace showed two distinct first-break slopes corresponding to seismic velocities of 204 m/s (direct P wave) and 384 m/s (refracted P wave) equivalent to the topsoil and conglomerate layers, respectively (Figure S10,https://www.raccefyn.co/index.php/raccefyn/article/view/1666/3225). The resulting two-layer model assembled from all shot records of Line 14 showed a gently dipping conglomerate under the low-velocity topsoil (Figure S10,https://www.raccefyn.co/index.php/raccefyn/article/view/1666/3225). The resulting structural map drawn at the top of the conglomerate from seismic refraction data shows depths consistent with those found in the southern areas (Figure S11,https://www.raccefyn.co/index.php/raccefyn/article/view/1666/3225).
Sedimentology
The top few meters of the conglomerate could be accessed by the dredges. The conglomerates are polymictic, matrix-supported, with clasts of volcanic and igneous composition and abundant intraclasts represented by semi-consolidated muddy layers and pieces of tree trunks of different sizes (Figure 6). Clasts are subrounded and subangular ranging from 0.2 cm to 2.5 cm in size. The clasts show no imbrication or internal stratification. The matrix is made up of medium to coarse sand, poorly sorted, with subrounded clasts, made predominantly of quartz with some lithics. Some samples also had a muddy sandstone matrix. The conglomerate's clast composition is dominated by chert, which makes up for 41% of the clasts, and vein quartz, with 28% of the clasts. In all the samples, there were also intermediate phaneritic igneous clasts with 14% and 11% of aphanitic and porphyritic mafic igneous clasts. Schists with 4% and mafic phaneritic clasts with 2% were the minor components and were not present in all the samples. Bone fragments of different sizes were often found within the conglomerates and the large ones, including skulls and tusks, had conglomerate clasts attached to the cavities.
Age
Wood samples found as clasts in the conglomerate produced reliable dates. Two out of three yielded C14 dates and the third one was beyond the limit for C14 dating. The two samples yielded dates of µ = 41,546 Cal Yr. BP (42,198 - 40,841 Cal Yr. BP) and µ = 13,689 Cal Yr. BP (13,780 - 13,575 Cal Yr. BP) (Table 1,Figure S13,https://www.raccefyn.co/index.php/raccefyn/article/view/1666/3225). None of the three bone samples had enough collagen material to yield a dating.
Table 1 Radiocarbon dating of three wood samples, ICA laboratory
Lab access number | Sample | Material | Conventional radiocarbon age (BP) | Calibrated age |
---|---|---|---|---|
ICA 17W/1049 | STRI 44179 | Wood | >40,000 BP | |
ICA 17W/1050 | STRI 44177 | Wood | 37010 +/- 400 BP | Cal 40250 - 38890 BC |
ICA 17W/1051 | STRI 44176 | Wood | 11890 +/- 40 BP | Cal 11830 - 11630 BC |
Forest composition
The muddy layer assemblage (STRI ID: 44171) comprised 1,796 palynomorphs corresponding to 45 different morphotypes (Supplementary information S2,https://www.raccefyn.co/index.php/raccefyn/article/view/1666/3225). Cyperaceae and Ambrosia types were found overrepresented accounting each one for more than 100% of the sum of the less abundant morphotypes altogether (372% and 186%, respectively). Ambrosia grains also showed signals of being reworked material (probably from the Cenozoic), thus, its presence was not interpretable in terms of the environmental setting. Only 33 palynomorphs accounted for the total pollen sum including Arecaceae (27.6%) and Moraceae-Urticaceae (21.8%) dominating the pollen total sum while Apocynaceae, Amaranthaceae, and Celtis also contributed with 4% to 9%. The aquatic taxa Typha and Sagittaria were 10% and 5%, respectively, and the spores sum including Lycopodium and Selaginella was 8.4% of the total pollen sum. The intraclast assemblage (STRI ID: 44168) was composed of 17 different palynomorphs totaling 427. From these, only 13 taxa contributed to the pollen sum with 85 grains. There was a high proportion of reworked material including Polypodiisporites aff. specious and Maurittidiites franciscoi var. minutus probably from the Cenozoic formations outcropping along the foothills of the Cauca Valley, mainly La Paila Formation (Jaramillo et al., 2009). The Cyperaceae (60%), Arecaceae (15%), Moraceae-Urticaceae (6%), and Bignoniaceae (5%) taxa were the most abundant pollen types. Acanthaceae, Apocynaceae, and Polygonaceae also contributed to the total pollen sum. Spores of Selaginella type and Lycopodium were also present in the sample (11% and 2 %). The estimation of rarefied richness calculated on the total sum of the smallest sample (the intraclast) was 11.8 and 8.7 while the Shannon index was 1.3 and 1.7 for the muddy layer and the intraclast, respectively.
Although the pollen sum was almost an order of magnitude larger in the muddy sample and the total number of taxa was almost three times as large, the rarefied richness and the diversity index were similar for both assemblages indicating that the difference in the ecological traits between samples was attributable to the sample size rather than differential ecological structures and, consequently, the intraclast flora can be considered as a subset of the muddy sample.
The sample ordination from the DCA (Figure 7) suggests that the palynological assemblage of the muddy sample related more to the samples from the bottom of the Quilichao sequence. The CONNIS clustering closely resembled that by Berrio et al. (2002), although our analysis was based on the site scores from the DCA ordination instead of the original assemblages (Figure 7). Our cluster grouped the muddy sample within the older zone (QIO-1b) in the original publication with a cophenetic distance smaller than the observed to discern among groups in Berrio et al.'s dataset. The Euclidean distances among samples exclusively from Quilichao-1 DCA scores varied between 0.013 and 2.767 standard deviations (SD) while those between the muddy sample and all the Quilichao samples were considerably larger, between 1.085 and 3.110. The samples closer to the muddy assemblage were, again, those from the Upper Pleistocene samples from Quilichao (Figure 7, bottom). The palynological assemblage from the muddy sample was closely related to zone QUI-1a (~13,000 to 10,520 14C yr BP) representing the typical Caribbean dry forest dominating the CV vegetation during the latest Pleistocene and early Holocene (Berrio et al., 2002). Furthermore, the palynological record indicated the lack of extensive grasslands in the CV during this period. The main difference with the QIO-1a zone in the Quilichao-1 record was that our assemblage lacked the contribution of the Andean forest elements (Alnus, Myrica, and Quercus), probably due to reduced connectivity with sources from upstream.
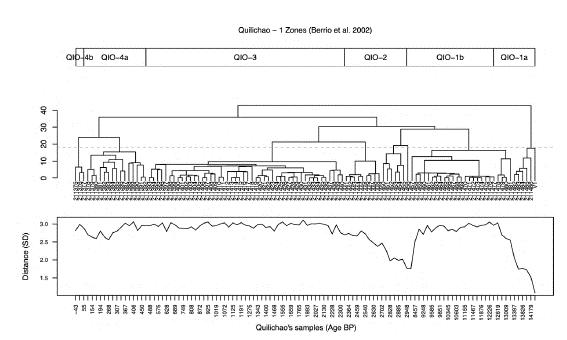
Figure 7 Comparison of the Quilichao-1 and the muddy sample assemblages. Top: Zones and subzones based on the cluster analysis and visual inspection of the down-core changes in the pollen spectra (Berrío et al., 2002)charcoal and radiocarbon data are presented. These records document the Late-glacial and Holocene dry forest vegetation, fire and environmental history of the southern Cauca Valley in Colombia (1020 m. Center: CONISS clustering based on the four axes from the DCA ordination. The dashed line marks the cophenetic distance where the muddy sample (V1) gathers the older branch of the cluster. Bottom: Euclidean distances between the muddy sample and the Quilichao-1 assemblages. Notice the similarity of the muddy sample and the samples at the bottom of the core.
Discussion
The high contrast of the fossil-bearing conglomerate layer observed by independent geophysical methods clearly indicated the lenticular shape of the deposits observed at Platanares, Paso La Torre, and the two intermediate areas (Figures S5-S11,https://www.raccefyn.co/index.php/raccefyn/article/view/1666/3225). Furthermore, the thickness of the layer ranged from 15 to 44 meters in Platanares (Figures 3,S5,S6,S7,https://www.raccefyn.co/index.php/raccefyn/article/view/1666/3225) and from 14 to 29 meters in Paso La Torre (Figures 4-5), and the lenses were aligned NNE-SSW dipping towards the SE (Figures 3,5). The internal consistency within the two datasets provides additional reassurance of the accuracy of the model. The P-wave velocities clearly fell in two distinct non-overlapping populations and the slow velocities measured in the topsoil were consistently about half the velocity of the conglomerate. These distinct velocity populations reduce the probability of rock misidentification and, thus, result in clear-cut layer models. While electrical resistivity values are less compelling than seismic velocities (given that absolute resistivities may suffer from mathematical artifacts introduced during the geophysical inversion process) the south-to-north gradual increase observed in seismic velocities is evidently mimicked by a gradual increase in electrical resistivity. Furthermore, the rapid convergence of ERT inversions (converging consistently within a few iterations) attests to the robustness of our models and relatively low noise levels in our field data.
The lenticular geometry of the conglomerate beds together with the poor sorting, the lack of imbrication or internal stratification of the conglomerate, and the polymodal grain size distribution suggest deposition in an alluvial fan (Tucker, 2001). The composition of the clasts, dominated by black chert and vein quartz and also containing basalts and gabbro, could be interpreted as sourced from the nearby eastern flanks of the Western Cordillera where such lithologies are exposed (Nivia, 2001). However, an eastern source cannot be completely ruled out by the conglomerate clast composition alone as units with similar lithologies are also present in the western slopes of the Central Cordillera (Nivia, 2001), even though this source seems less likely.
Many of the large proboscidean fossils are often found with conglomerate clasts attached to the bone suggesting that they are found in the conglomerate layer. Unfortunately, the bones themselves did not have enough enamel to be dated. Additional taphonomic studies are required to understand the nature of the proboscidean fossilization that seems related to the alluvial fan deposition. A radiocarbon age of 13,689 Cal Yr. BP (13,780 - 13,575 Cal Yr. BP) (Table 1) indicates that the deposit accumulated during the latest Pleistocene and the overall palynological record demonstrates that the vegetation corresponds to an inter-Andean dry forest. The most abundant taxon was Cyperaceae, which has been reported as part of the open vegetation community rather than the swamp assemblage in the dry ecosystems of the CV (Ramírez-Padilla et al., 2015). Therefore, its abundance does not necessarily imply the prevalence of wetlands. Arecaceae and Moracea-Urticaceae were abundant as well comprising ~50% of the pollen sum on each sample while Apocynaceae, Bignoniaceae, Asteraceae, and Amaranthaceae were also modest components of both assemblages. The environments reflected by these assemblages coincide with those of dry forests similar to extant inter-Andean dry forests and swamps described in the region (Ramírez-Padilla et al., 2015), a trait that has been persistent throughout the Holocene (Berrio et al., 2002; González-Carranza et al., 2008). A difference between the samples was the considerable extant amounts of aquatic taxa such as Typha and Sagittaria and the variety of spores in the muddy sample, which is probably a taphonomic effect. While the intraclast is a portion of old fluvial plain eroded incorporated as a clast into an alluvial fan conglomerate in a high-energy environment, the high abundance of the aquatic taxa in the muddy sample is probably the result of a filled oxbow lake with standing water and abundant aquatic vegetation. Both multivariate analyses suggested that the palynological assemblage is similar to those found in older Quilichao sequence sediments (Berrio et al., 2002) that are also Upper Pleistocene (Figure 7) representing the typical Caribbean dry forest that dominated the vegetation of the Cauca Valley during the latest Pleistocene (Berrio et al., 2002). Furthermore, the palynological record indicates the lack of extensive grasslands in the Valley during this time period.
Conclusions
Aworld-class locality ofwell-preserved proboscidean fossils in western Colombia is reported and characterized here for the first time providing a strong anchor for paleontological, paleogeographic, and paleoenvironmental reconstructions of the Americas during the late Quaternary. The conglomerate contains the proboscidean fauna accumulated during the Late Pleistocene in alluvial fan settings. The geometry of the bodies is lenticular and oriented perpendicularly to the modern course of the Cauca River.
The vegetation during the Late Pleistocene in the Cauca Valley corresponds to a tropical dry forest and no evidence of extensive savannas was found.
The three-dimensional geometry of the buried and submerged fossiliferous rock layer was successfully revealed using two independent geophysical methods based on seismic velocities and electrical resistivities.