Servicios Personalizados
Revista
Articulo
Indicadores
-
Citado por SciELO
-
Accesos
Links relacionados
-
Citado por Google
-
Similares en SciELO
-
Similares en Google
Compartir
Universitas Psychologica
versión impresa ISSN 1657-9267
Univ. Psychol. vol.13 no.3 Bogotá jul./set. 2014
https://doi.org/10.11144/Javeriana.UPSY13-3.rics
Response-Inhibition Capacity and Short-Term Memory are Robust to the Effects of High Fat Diet (HFD) during Pre and Periadolescence*
La capacidad de inhibición de respuesta y la memoria a corto plazo son robustos a los efectos de la dieta alta en grasa (HFD) durante la pre y periadolescencia
Elizabeth Watterson**
Federico Sanabria
Arizona State University, United States
Arturo R. Zavala***
California State University Long Beach, United States
Gregory J. Privitera****
Saint Bonaventure University, United States
*This study was supported by two grants from the National Institutes of Health (MH094562 and DA032632) and by seed funding from the College of Liberal Arts and Sciences, Arizona State University. The authors wish to thank Jennifer May, Bianca Zietal, Brittany Clark, Cameron Gibbons, Charles Wilson, Samuel Williams, Aaron Chaumont and Tara Mahmood for their contributions to data collection. The authors also wish to thank Gabriel Mazur and Ryan Brackney for their thoughtful comments during manuscript preparation.
**E-mails: elizabeth.watterson@asu.edu, federico.sanabria@asu.edu
***E-mail: arturo.zavala@csulb.edu
****E-mail: gprivite@sbu.edu
Recibido: enero 16 de 2014 - Revisado: marzo 27 de 2014 - Aceptado: marzo 27 de 2014
Para citar este artículo
Watterson, E., Sanabria, F., Zavala, A. R., & Privitera, G. J. (2014). Response-inhibition capacity and short-term memory are robust to the effects of high fat diet (HFD) during pre and periadolescence. Universitas Psychologica, 13(3), 1161-1180. http://dx.doi.org/10.11144/Javeriana.UPSY13-3.rics doi:10.11144/Javeriana.UPSY13-3.rics
Resumen
Se tiene evidencia que la exposición a una dieta alta en grasa puede ser perjudicial para la cognición a lo largo de la vida. Hemos demostrado previamente que el aprendizaje contexto-estímulo es sensible a los efectos de una dieta alta en grasas durante la adolescencia, pero no la edad adulta. En el presente estudio se determinó si la exposición a una dieta alta en grasa en la pre y peri adolescencia interfiere con la capacidad de respuesta de inhibición, el aprendizaje de reglas, y la memoria en la edad adulta. Las ratas fueron alimentadas con una grasa alta o dieta baja en grasa durante la pre y periadolescencia y se completaron las pruebas de comportamiento como adultos para evaluar la capacidad de respuesta de inhibición y eficacia reforzador regla-aprendizaje y la memoria a corto plazo. Los resultados indican que la dieta rica en grasas durante la pre y periadolescencia puede tener efectos a largo plazo sobre la eficacia del refuerzo y la atención sostenida. Sin embargo, los resultados indican que o bien el periodo de pre y periadolescencia es demasiado corto para que una dieta alta en grasas pueda inducir déficits a largo plazo en la respuesta de inhibición, regla de aprendizaje o la memoria, o que la maduración en ausencia de una dieta alta en grasas rescate a los individuos de estos déficits.
Palabras clave: adolescencia; enriquecimiento ambiental; dieta alta en grasa; memoria; Ratas Sprague Dawley; la capacidad de inhibición de respuesta; programa Fijo de intervalo mínimo; Tarea Alternancia de Intervalo-demorado.
Abstract
Several lines of evidence demonstrate that high fat diet exposure can be detrimental to cognition across the lifespan. We have previously shown that context-stimulus learning is sensitive to high fat diet effects during adolescence but not adulthood. In the present study we determined if pre and periadolescent high fat diet exposure interferes with response -inhibition capacity, rule - learning, and memory during adulthood. Rats were fed a high fat or low fat diet during pre and periadolescence and completed behavioral testing as adults to assess response -inhibition capacity and reinforcer efficacy rule-learning and short-term memory. Results indicate pre and periadolescent high fat diet may have long-term effects on reinforcer efficacy and sustained attention. However, results indicate that either the pre and periadolescence period is too short for a high fat diet to induce long-term deficits in response-inhibition, rule -learning, or memory, or that maturation in the absence of a high fat diet rescued these deficits.
Keywords: adolescence; environmental enrichment; high fat diet; memory; Sprague Dawley rats; response-inhibition capacity; Fixed Minimum Interval schedule; Variable Interval-Delayed Alternation task
Consumption of a high fat diet (HFD) is implicated in a variety of health concerns including overweight and obesity, diabetes, metabolic syndrome, and cardiovascular disease (Eckel & Krauss, 1998; Fung et al., 2001; McCullough, 2011; Riccardi, Giacco & Rivellese, 2004; van Dam, Willett, Rimm, Stampfer & Hu, 2002). Exposure to HFD also contributes to impaired cognitive functioning across the lifespan. In children, a HFD exposure is associated with an increased risk for depression and anxiety (Rofey et al., 2009) and attention deficit hyperactivity disorder (ADHD) (Waring & Lapane, 2008). Adults who consume a HFD have poor cognitive functioning that includes impaired semantic and declarative memory, and reduced psychomotor speed (Eskelin-en et al., 2008), and are at higher risk of developing Alzheimer's disease (Luchsinger, 2002).
Animal models also exhibit the detrimental effects of HFD on cognition in rodents and its related neural mechanisms (Greenwood & Winocur, 1990; Vucetic, Carlin, Totoki, & Reyes, 2012; Vucetic, Kimmel, & Reyes, 2011; Vucetic, Kimmel, Totoki, Hollenbeck & Reyes, 2010; Winocur, 1985, 1991; Yu et al., 2010). For instance, in utero exposure to a HFD leads to spatial memory and learning deficits (Yu et al., 2010), reduced hippocampal brain-derived neurotrophic factor (BDNF), reduced arborization of new hippocampal neurons (Tozuka et al., 2010), hippocampal inflammation (Bilbo & Tsang, 2010), impaired Morris water maze performance (White et al., 2009), changes in the regulation and expression of dopamine transporters (DAT) and µ opioid receptors (MOR) (Vucetic et al., 2011; Vucetic et al., 2012) and altered reward processing (Vucetic et al., 2010; Vucetic et al., 2011; Vucetic et al., 2012). Postnatal HFD exposure also leads to learning and memory deficits (Greenwood & Winocur, 1990; Winocur & Greenwood, 1999). Similarly, adult exposure to HFD has a detrimental impact on hippocampal structure and functioning and on performance on hippocampus-dependent tasks such as the Morris water maze (Pathan, Gaikwad, Viswanad & Ramarao, 2008), the water radial arm maze (Granholm et al., 2008), the Stone-T maze (Pistell et al., 2010), and the Y-maze (Kosari, Badoer, Nguyen, Killcross & Jenkins, 2012).
Extant evidence on the effect of pre and periadolescent HFD exposure on cognition in rodents is ambiguous. In most studies, exposure to HFD starts in pre and periadolescence but continues for up to 12 months, and behavior is assessed in adulthood (Boitard et al., 2012; Greenwood & Winocur, 1990; Greenwood & Winocur, 2005; Valladolid-Acebes et al., 2011; Vucetic et al., 2011, Vucetic et al., 2012; Winocur & Greenwood, 1999). Such studies have shown that HFD lead to deficits in spatial learning in the radial arm maze (Valladolid-Acebes et al., 2011), rule learning, and memory impairments on the variable interval delayed alternation (VIDA) task (Greenwood & Winocur, 1990, 2001; Winocur & Greenwood, 1999), reduced relational memory flexibility, hippocampal neurogenesis (Boitard et al., 2012), dopaminergic (DA) dysregulation (Vucetic et al., 2010; Vucetic et al., 2010), and MOR dysregulation (Vucetic et al., 2011). While it is apparent from these studies that the effects of HFD are detrimental, it is unclear whether HFD exposure restricted to the pre and periadolescent period is necessary or sufficient for cognitive deficits to occur. For instance, we have recently shown that pre and periadolescent exposure to HFD disrupts conditioned place preference (CPP) learning in adulthood (Privitera, Zavala, Sanabria & Sotak, 2011) . Notably, an identical 20-day exposure to HFD in adulthood failed to produce impairments in CPP. These results suggest that pre and periad-olescent exposure may be necessary and sufficient for the expression of some HFD-related learning and cognitive deficits in adulthood.
The present study sought to further investigate long-lasting deficits associated with pre and periadolescent (i.e., PND 21-40, a period analogous to preadolescence and adolescence in humans (Spear, 2000)) HFD exposure. Two experiments were conducted to examine, in rats, the effect of pre and periadolescent HFD exposure on response inhibition capacity and on rule learning and memory during adulthood. Response inhibition capacity was assessed using the fixed minimum interval (FMI) schedule of reinforcement (Hill, Herbst & Sanabria, 2012; Mechner & Guevrekian, 1962; Mika et al., 2012) ; whereas rule learning and memory deficits were assessed using the VIDA task (Greenwood & Winocur, 1990; Winocur, 1985). Because environmental enrichment (EE) improves HFD-associated impairments on the VIDA task (Winocur & Greenwood, 1999), we sought to determine whether EE reversed adult impairments in VIDA performance that were associated with pre and periadolescent exposure to HFD. Behavioral testing was followed by a sucrose preference test to determine whether groups differed in the extent to which they "liked" sucrose (Berridge, 1996).
Methods
Animals
Sixteen experimentally-naïve male Sprague Daw-ley rats (Charles River, Hollister, California) were used. Rats arrived on post-natal day (PND) 21. Rats were pair-housed immediately upon arrival and throughout the remainder of the experiment except where otherwise specified (see Environmental Enrichment). The colony room was on a 12:12-h day: night cycle, with lights on at 1700 h. Feeding procedures varied across rats for experimental purposes; details are provided in the Procedure section. All experimental protocols were conducted in accordance with the guidelines provided by the National Institutes of Health and approved by the Arizona State University Institutional Animal Care and Use Committee.
Apparatus
Experiments were conducted in eight MED Associates (St. Albans, VT) modular test chambers (three were 305 mm long, 241 mm wide, and 210 mm high; five were 305 mm long, 241 mm wide, and 292 mm high), each of which was enclosed in sound-and light-attenuating boxes equipped with ventilation fans. The front/back walls and the ceiling of the test chambers were made of Plexiglas; the front wall was hinged and served as a door to the chamber. One of the two aluminum side panels served as a test panel. The floor consisted of 5mm steel rods spaced 16 mm apart and positioned 36 mm above a catch pan. A square opening (51mm each side) located 15 mm above the floor and centered on the test panel provided access to a food hopper (MED Associates, ENV-200-R2M). One 45-mg sucrose pellet (Test Diet, Richmond, IN) was delivered to the hopper with each activation of the dispenser. A multiple tone generator (MED Associates, ENV-223) was used to produce 3 kHz tones at approximately 75 dB through a speaker (MED Associates, ENV-224AM) centered on the top of the wall opposite to the test panel, 240 mm above the floor of the chamber. Two retractable levers (ENV-112CM) were located on either side of the food hopper. Three-color light stimuli (ENV-222M) were mounted 35 mm above each lever; they could be illuminated yellow, green, and red. A force of approximately 0.2 N applied to the end of the lever was necessary for a lever press to be recorded. The ventilation fan mounted on the rear wall of the sound-attenuating chamber provided masked noise of approximately 60 dB. Experimental events were arranged via a Med- PC® interface connected to a PC controlled by Med-PC IV® software.
Procedure
Diet manipulations
Upon arrival, rats were randomly assigned to either a high fat diet (60 kcal % fat; Research Diet, Formula D12492) or low fat diet (10 kcal % fat; Research Diet, Formula D12450B). Rats had ad libitum access to high fat (HF) or low fat (LF) chow from PND 21-40. Water was always available ad libitum. Cagemates received the same diet treatment. On PND 41 rats were switched from high or low fat diets to Teklad 8604 rodent standard chow (Harlan Teklad, Madison, Wisconsin; 14 kcal % fat), which was available ad libitum until PND 70. Starting on PND 71 the amount of time during which food was freely available in the homecage was gradually reduced to 1 h/ day. Animals were weighed every morning and fed Teklad 8604 rodent chow in the afternoon, a minimum of 12 h before the scheduled experiment on the following day.
Fixed Minimum Interval (FMI) Schedule
Sessions were conducted daily, 7 days per week. Training started on PND 80 with one to two sessions of hopper training, which consisted of presenting sucrose pellets on a variable time (VT) 15-s schedule. Autoshaping commenced once subjects reliably ate all sucrose pellets provided during hopper training. Four autoshaping sessions were conducted, during which lever insertions were paired with sucrose pellet delivery. Following autoshaping, FMI sessions commenced, during which subjects initiated each trial by pressing a lever press (initial response), and were required to withhold a head entry response (terminal response) for a criterial time (t), which started at 0.5 s and increased by 1.25% following every correct response until the criterial time was 6 s. Each interval between an initial and a terminal response constituted an interresponse time (IRT). An IRT was considered correct if it was equal or greater than the criterial time; IRTs shorter than the criterial time were deemed incorrect and were never reinforced. Before reaching the 6-s criterion, every correct IRT was reinforced. After reaching the 6-s criterion, reinforcement for correct IRTs was arranged on a variable interval (VI) 30-s schedule, which is described in the Conjunctive VI schedule section. Sessions lasted for 1 h or until 150 reinforcers were achieved, whichever occurred first. Rats completed 10 conjunctive FMI 6-s VI 30-s sessions, the last 5 of which were analyzed.
Conjunctive VI Schedule
During FMI sessions, reinforcement was arranged on a VI 30-s schedule to ensure reinforcement rates were consistent across subjects, thereby reducing the potential for between-subject performance-driven differences in reinforcement rates. The VI schedule was implemented by randomly selecting without replacement an interval from a 12- item Fleschler-Hoffman list (Fleschler & Hoffman, 1962) at the beginning of the session and after each reinforcer. A stopwatch ran throughout the session; when it reached the randomly selected interval, reinforcement was programmed for the next correct IRT. If the interval elapsed within an IRT, that IRT was not reinforced; instead, reinforcement was set up for the next correct IRT. The conjunctive VI schedule was introduced upon reliable performance on t = 6 s. The VI schedule increased progressively over the course of multiple sessions from VI 9 s to 15. 23 and30 s. Progression was dependent upon reliable performance at each VI schedule, which was defined as > 50% correct IRTs across three consecutive sessions. Only one subject failed to meet criterion for progression and remained in the VI 9 s schedule for the entire study.
Variable Interval Delayed Alternation (VIDA) Task
VIDA testing started on PND 120 after all subjects finished the FMI procedure. The VIDA task (Winocur, 1985) is a Go/No-Go task in which Go and No-Go trials are presented in strict alternation with the first trial always being a Go trial. Data from the first Go trial was not included in analyses. Each Go and No-Go trial was 10-s long. Every lever press on a Go trial was reinforced with a sucrose pellet. Lever presses made during No-Go trials were not reinforced. Sessions consisted of 8 Go trials and 8No-Go trials separated by inter-trial intervals (ITIs) of 0, 7, 20, and 60 s, with each interval preceding Go trials twice and No-Go trials twice. For each trial type (Go vs. No-Go), ITIs were presented in pseudorandom order by sampling without replacement from a 4-item list of ITIs. Levers remained retracted during ITIs and were inserted at the beginning of each Go and No-Go trial, signaling the start of the trial. VIDA baseline (BL) testing was conducted for 15 days with sessions running once daily, 7 days per week.
Environmental Enrichment (EE)
On PND 150 all subjects were transferred to environmental enrichment chambers (74 X 91 X 36 cm), where they were housed in groups of four. Each group of four consisted of two LF cagemates and two HF cagemates. Environmental enrichment chambers were equipped with bedding, PVC pipes, ceramic toys, two water bottles, two food bowls, running wheels, and nesting materials. All toys were changed three times per week. After 20 days in environmental enrichment (EE), VIDA data was gathered for five days. Rats were then returned to standard housing conditions for 20 days and left undisturbed. Finally, VIDA performance was assessed again for five sessions (Post-Enrichment, PE).
Sucrose Solution Preference
Immediately after all behavioral testing was completed, preference for sucrose solution (15%, w/v) was measured across two days in the afternoon after rats had been water deprived for 4 h. Testing was conducted in a cage similar to their homecage, which contained bedding and a bottle. On Day 1, subjects were randomly assigned to receive 1 h of free access to either water or sucrose solution. On Day 2, subjects were given 1 h of free access to whichever solution they did not receive the previous day. The order in which rats received sucrose solution and water was counterbalanced within each group. The amount of water and sucrose solution consumed was recorded for each rat and compared across groups.
Data Analysis
FMI performance
Latencies were computed as the time between lever insertion and the initial response. Because reinforcer deprivation yields shorter latencies (Mechner & Guevrekian, 1962) and mild chronic stress (Mika et al., 2012) and pre-feeding (unpublished data from our laboratory) yield longer latencies, latencies provided a measure of incentive motivation. Median latencies were calculated daily and then averaged over the last five days of FMI testing for individual animals. Latencies were separated into those that followed reinforced trials (post-R) and those that followed non-reinforced trials (post-N). Post-N latencies include latencies following both correct and incorrect non-reinforced trials which were collapsed because we have previously shown them not to differ significantly (Mika et al., 2012). Post-R and post-N latencies were analyzed separately.
Mean IRTs and the percentage of correct IRTs were calculated daily for each subject and then averaged across the last five FMI sessions. Previous work in our laboratory has shown latencies, but not IRTs to be sensitive to previous trial outcome (Mika et al., 2012), so mean IRTs and the percentage of correct IRTs were not analyzed according to previous trial outcome.
Temporal Regulation (TR) Model Parameters
The Temporal Regulation model (Sanabria & Killeen, 2008) was used to describe IRT distributions pooled across the last 5 FMI sessions. The model assumes that IRT distributions are a mixture of two underlying distributions, one of IRTs controlled by timing processes (gamma-distributed timed IRTs) and one of IRTs that are not controlled by timing processes (exponentially-distributed non-timed IRTs). Thus,
p(IRT = t - t < δ) = 0
N, c, k, δ > 0; 0 > P >1 (1)
where p(IRT = t) is the probability of an IRT of duration t; P is the probability that the IRT will be timed; r(N,c) is the gamma distribution of timed IRTs, with shape parameter N and scale parameter c; k is the mean non-timed IRT; δ is the shortest possible IRT. These parameters were used to estimate the mean (μ = Nc + δ) and standard deviation (δ = N0.5c) of timed IRTs. Normalized mean timed IRT (θ = μ / 6 s) were used to draw inferences on response inhibition capacity, (Hill et al., 2012) with higher estimates indicative of greater capacity. The coefficient of variation (w = δ/μ) is an index of timing precision (Hill et al., 2012; Mika et al., 2012), with larger estimates indicative of less precise timing. TR model parameters (θ, w, P, k) were calculated for each subject from the pooled distribution of IRTs from the last five FMI sessions.
VIDA Performance
Median latencies were calculated for each trial type (Go and No-Go) following each ITI for individual animals. Mean median latencies were calculated by averaging median latencies for each trial type following each ITI duration in each of three blocks of five sessions (last 5 during BL, 5 during EE, 5 during PE) for individual subjects. In trials that did not contain a response, latencies were recorded as 10 s (the length of the trial) and the number of responses was recorded as zero. Go and No-Go latencies are typically reported as a ratio(Greenwood & Winocur, 1990), however, we analyzed these latencies separately so as improve our ability to determine whether Go or No-Go latencies were differentially sensitive to diet manipulations.
Mean run IRTs (i.e., the mean time between consecutive lever presses) were calculated from mean latencies and mean number of responses for each trial type following each ITI, i.e., (10 s-mean latency) / mean number of responses. Mean run IRTs were calculated for individual subjects and were then averaged across blocks of five sessions (BL, EE and PE).
Inferential statistics
All inferential statistics were conducted on log-transformed data. Weights were tracked and compared across groups at arrival and during the last five days of HF/LF diet access, regular chow access, FMI testing and BL, EE, and PE VIDA testing. Comparisons were made using a repeated measures analysis of variance (ANOVA) with group as the between-subjects factor and condition as the within-subjects factor. The effect of diet on FMI latencies was analyzed using a 2 (diet: HF vs. LF) X 2 (trial type: post-R vs. post-N) mixed-design ANOVA. The effect of diet on FMI IRTs and TR parameter estimates (θ, w, P, and k) were also analyzed using a series of ANOVAs. The effect of diet and environmental enrichment on VIDA dependent measures —median latency and mean run IRT—was analyzed using a 2 (diet) X 2 (trial type: Go vs. No Go) X 3 (enrichment condition: BL vs. EE vs. PE) X 4 (ITI: 0 vs. 7 vs. 20 vs. 60 s) mixed-design ANOVA. Significance threshold was set at P = 0.05. Significant 2-way interaction effects were followed up by pairwise t-tests. Significant 3-way interaction effects were followed up by 2-way ANOVAs at each level of the third factor. A significant 4-way interaction effect was not observed. Near-significant main and interaction effects (P < 0.1) that involved diet were followed up by pairwise trimmed boostrapped comparisons (Keselman, Algina, Lix, Wilcox & Deering, 2008), to establish whether non-normality and outliers may have reduced statistical power (see Bootsrapping section). Sucrose preference was analyzed using 2 (diet) X 2 (liquid type: sucrose solution vs. water) mixed-design ANOVA.
Bootstrapping
Pairwise trimmed bootstrapped comparisons (Ke-selman et al., 2008) were conducted as follows. First, the maximum and minimum score for each diet group (n = 8) were eliminated and (trimmed) means were calculated for the remaining scores in
each group. The difference between the trimmed means across groups was denominated the observed difference. Second, 16 scores were resampled by randomly sampling with replacement from the pooled non-trimmed scores. Third, the difference between the trimmed mean of the first 8 and the trimmed mean of the last 8 resampled scores was calculated. This difference was denominated the resampled difference. The second and third steps were then repeated to produce 5000 resampled differences. A bootstrap P-value was then computed as the proportion of resampled differences that were equal to or more extreme than the observed difference.
Results
Animal weights were tracked immediately upon arrival and throughout the entirety of the study and are displayed in Figure 1. An ANOVA revealed a significant main effect of Condition, F (6,84) = 1048.38, P < 0.001 with weights increasing across conditions. However, there was no significant effect of diet, P = 0.218, or significant diet X condition interaction, P = 0.601.
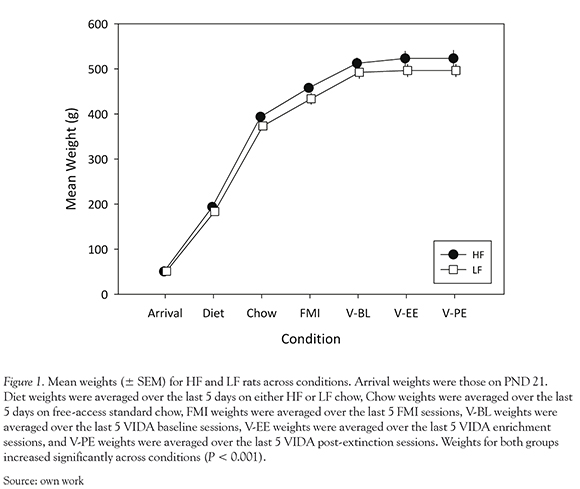
FMI Performance
Reinforcement rates (reinforcers per minute) were calculated and averaged across the last 5 FMI sessions. Individual reinforcement rates are shown in Table 1. On average, the HF group earned 0.94 (SEM = 0.04) reinforcers per minute and the LF group, on average, earned 0.93 (SEM = 0.03) reinforcers per minute. The similarity in reinforcement rates across groups indicates that any group differences in FMI performance cannot be explained by differences in the number of reinforcers obtained.
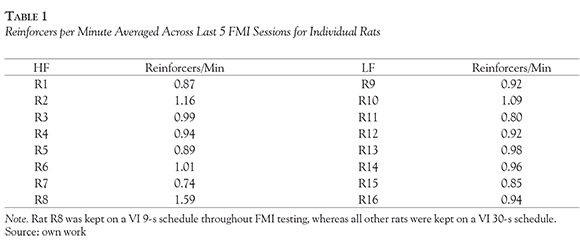
Latencies
Mean median latencies for HF and LF rats are displayed by type (post-R vs. post-N) in Figure 2. A significant main effect of trial type was observed, F (1, 14) = 61.05, P< 0.001. Post-R latencies were significantly longer than post-N latencies for both HF (M = 2.7 and 1.64, respectively), t(15) = 3.35, P = 0.004, and LF (M = 2.93 and 1.18, respectively) groups, t(15) = 4.78, P < 0.001. Results also indicate a nearly significant diet Xtrial type interaction effect, F (1, 14) = 4.03, P = 0.064. Bootstrapping was used to further analyze this interaction effect. Bootstrapping revealed that post-N latencies were significantly longer for the HF group than for the LF group, P = 0.032; post-R latencies did not significantly vary across groups, P = 0.232.
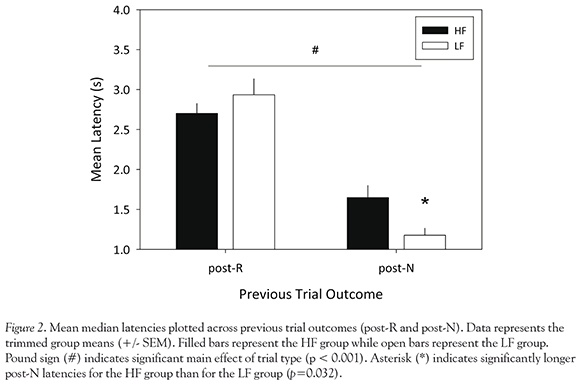
IRTs
Analyses revealed that mean IRTs did not vary significantly between the HF (M =6.78) and LF (M = 6.58) groups, P = 0.794. The mean distribution of IRTs for each group is displayed in Figure 3. The curves corresponding to each distribution are the mean individual fits of the probability density function predicted by the Temporal Regulation (TR) model. Overall, the TR model provided a good fit to IRT distributions as indicated by the proximity of the fitted curves for each group.
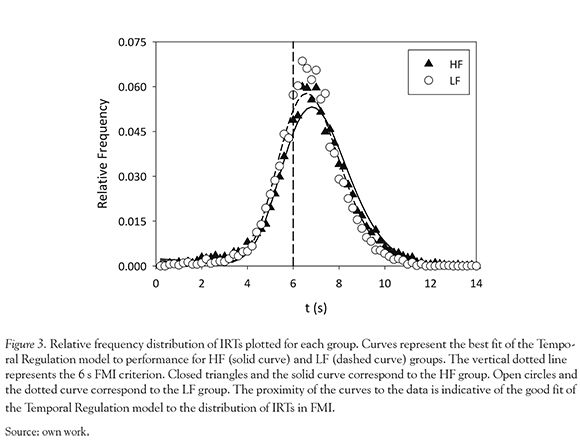
The mean estimates of TR parameters are shown in Table 2. The mean estimates of the proportion of gamma-distributed (timed) IRTs, P, did not vary significantly across groups, P =0.264. The mean estimates of non-timed interval, k, was shorter for the LF group than for the HF group, however this effect was only near-significant, P = 0.088. Bootstrapping was applied to further analyze the effect of diet on estimates of k. This analyses revealed that estimates of k were significantly shorter for the LF group than for the HF group, P = 0.04. No significant effect of diet was observed on estimates of θ, P = 0.405, or w, P = 0.521.
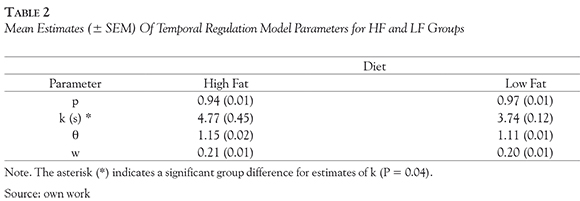
VIDA Performance
Run IRTs
Figure 4 shows mean run IRTs for each trial type (in separate panels), diet (indicated by symbols), enrichment condition, and following each ITI. No significant effect of diet was observed on VIDA performance, although a near-significant diet X trial type interaction effect on run IRTs was observed, F (1, 14) = 3.84, P = 0.07. A trimmed bootstrap follow up did not reveal a significant difference in mean run IRTs across diet treatments at any level of trial type.
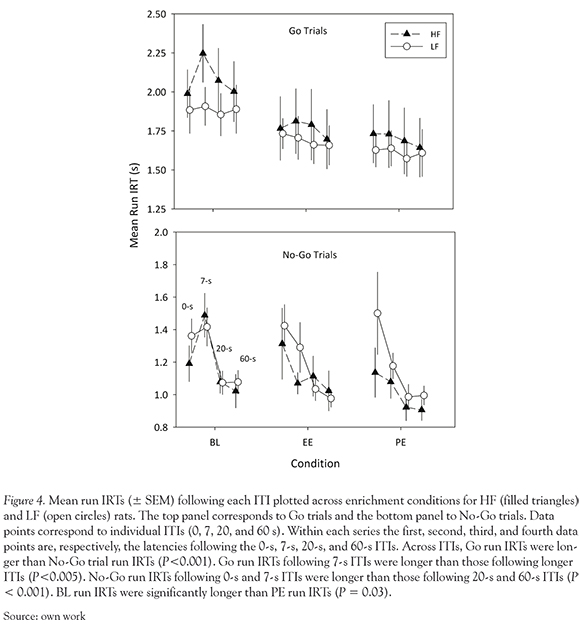
A significant trial type X ITI interaction effect on run IRTs was observed, F (3,12) = 19.76, P <0.001. Post hoc pairwise comparisons showed that, across ITIs, Go run IRTs were longer than No-Go run IRTs; t (15) ranged between 5.64 and 17.96, P -values were all < 0.001. This effect is not surprising, given that pellet consumption is likely to contribute to IRTs in Go trials, but not in No-Go trials. Go run IRTs following the 7-s ITI were longer than Go run IRTs following the 20-s ITI, t (15) = 3.70, P = 0.002, and the 60-s ITI, t(15) = 3.41, P = 0.004. No-Go run IRTs were consistently longer at shorter ITIs (0-s and 7-s ITIs) than at longer ITIs (20-s and 60-s ITIs); in all comparisons, 0-s vs. 20-s ITI, 0-s vs. 60-s ITI, 7-s vs. 20-s ITI, 7-s vs. 60-s ITI, t(15) > 5.32, P < 0.001. There was also a significant main effect of condition, F (2,13) = 5.95, P=0.015. Post hoc pairwise comparisons revealed that BL (M = 5.01) run IRTs were significantly longer than PE (M = 4.86) run IRTs, t(15) = 2.38, P = 0.03.
Latencies
No significant effect involving diet was observed on VIDA latencies. Figure 5 shows median latencies for each trial type (symbols) and enrichment condition, following each ITI (0-s, 7-s, 20-s, and 60-s), collapsed over diet groups. A significant trial type X condition X ITI interaction effect on VIDA latencies was observed, F(6, 84) = 2.61, P = 0.023. Post hoc analyses conducted separately on each ITI duration revealed a significant trial type X condition interaction effect on ITI 0-s latencies, F(2, 30) = 5.68, P = 0.008, a significant main effect of trial type on ITI 7-s latencies, F(1, 15) = 27.98, P<0.001, and a significant main effect of enrichment condition on ITI 60-s latencies, F(2, 30) = 7.88, P = 0.002.
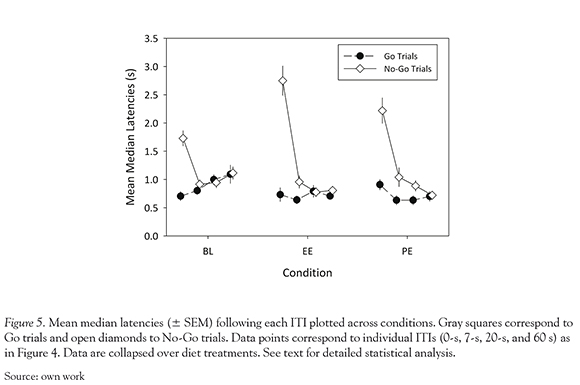
Following 0-s ITIs, Go latencies remained short and relatively constant across enrichment conditions. No Go latencies were longer than Go latencies, t(15) ranged between 6.58 and 10.38, all P-values < 0.001, and peaked during EE; BL vs. EE: t(15) = 3.94, P = 0.001; EE vs. PE: t(15) = 2.73, P = 0.016. Following 7-s ITIs, No-Go latencies were significantly longer than Go latencies, t(15) = 2.57, P = 0.021. The differences between No-Go and Go latencies were attenuated at the 20-s ITI. Following 60-s ITIs, latencies of both types declined significantly between BL and EE (t (15) = 2.96, P = 0.01) and PE (t(15) = 3.12, P = 0.007) conditions.
Post hoc analyses conducted separately on each level of trial type and on each enrichment condition revealed significant condition X ITI interaction effects on Go, F(4.85, 72.74) = 3.49, P = 0.007, and No-Go latencies, F(6, 90) = 4.52, P<0.001, and significant trial type X ITI interaction effects on BL, F(3, 45) = 22.15, P < 0.001, EE , F(3, 45) = 32.63, P < 0.001, and PE latencies, F(3,45) = 22.51, P < 0.001. Pairwise comparisons following up on condition X ITI interaction effects suggest that Go latencies at longer ITIs were generally longer in BL compared to EE (60-s ITI: t(15) = 2.59, P = 0.02) and PE (7-s ITI: t(15) = 2.46, P = 0.027; 20-s ITI: t(15) = 3.56, P = 0.003; 60-s ITI: t(15) = 2.32, P < 0.035) blocks. This pattern reflects a change in the relation of Go latency to ITI, which was generally positive during BL (0-s vs. 20-s ITI: t(15) = 2.38, P = 0.031; 7-s vs. 20-s ITI: t(15) = 2.74, P = 0.015), relatively constant during EE, and somewhat negative during PE (0-s vs. 7-s ITI: t(15) = 2.57, P = 0.021). BL No-Go latencies were shorter than EE latencies following 0-s ITIs, t(15) = 3.94, P = 0.001, but were longer than EE latencies, t(15) = 2.96, P = 0.01, and PE latencies, t(15) = 3.12, P = 0.007, following 60-s ITIs. Across conditions, No-Go latencies were longer at 0-s ITIs than at longer ITIs; t(15) ranged between 3.41 and 11.05, P -values ranged between 0.004 and < 0.001.
Effect of Diet on Sucrose Solution Consumption
The amount (g) of water and sugar water consumed during 1 hr free access is shown for each group in Figure 6. The top panel depicts mean water consumption for HF (M = 3.62 g) and LF (M = 5.62 g) groups whereas the bottom panel shows mean sugar water consumption for each group (M = 29.25 g and 28.50 g for HF and LF groups, respectively). A significant main effect of liquid type was observed, F (1,7) = 91.98, P < 0.001. Consumption of sugar water was significantly greater than consumption of water for the HF and LF groups; respectively, t(15) = 4.89, P < 0.001, t(15)= 5.19, P < 0.001. However, there was no observed effect of diet on water or sugar water consumption, F (1,7) = 0.08, P = 0.782.
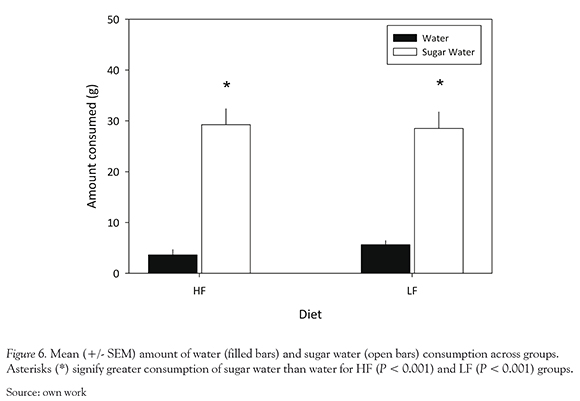
Discussion
The primary goal of the present study was to investigate the long-term consequences of pre and peri-adolescent HFD exposure on learning, memory and response inhibition capacity. Adult rats were tested on the FMI schedule of reinforcement (Mechner & Guevrekian, 1962) to assess response inhibition capacity (Hill et al., 2012; Mika et al., 2012) and also on the VIDA task to assess rule learning and memory (Winocur, 1985). VIDA performance has been shown to improve with environmental enrichment, particularly for rats maintained on a HFD (Greenwood & Winocur, 1990). We sought to determine whether environmental enrichment would reverse VIDA performance deficits associated with pre and periadolescent HFD exposure.
Effects of Diet on Motivation for Sucrose Reinforcement
FMI latencies provided a measure of motivation for sucrose reinforcement. Latencies following non-reinforcement (post-N) were significantly longer for HF rats than LF rats (Fig. 2); no such difference was observed in latencies following reinforcement (post-R). Across various schedules, including FMI (Mika et al., 2012), it has been shown that post-N latencies are typically shorter than post-R latencies (Harzem, Lowe & Priddle-Higson, 1978; Kello, 1972; McMillan, 1971; Mellon, Leak, Fairhurst & Gibbon, 1995; Papini & Hollingsworth, 1998; Priddle-Higson, Lowe, & Harzem, 1976; Staddon & Innis, 1966, 1969; Talton, Higa & Staddon, 1999; Zeiler, 1972). This difference may be due to postprandial behavior interfering with operant performance. Furthermore, previous studies have shown that chronic stress, which induces anhe-donia (Kleen, Sitomer, Killeen & Conrad, 2006), increases post-N latencies but not post-R latencies (Mika et al., 2012). These findings support the interpretation of our results as suggesting an enduring HFD-induced reduction in the reinforcement efficacy of sucrose, which may be an expression of a more generalized anhedonia. Such interpretation is consistent with reports of attenuated operant responding for sucrose caused by extended HFD exposure (Davis et al., 2008) and with impaired conditioned place preference for amphetamine (Davis et al., 2008), cocaine (Wellman, Nation & Davis, 2007), and foods with high sugar content and high fat content (Privitera, Mayeaux, Schey, Lapp, n.d.).
Differences in post-N latencies between groups may also be related to a HFD-induced decrease in sucrose "wanting" (related to appetite or incentive motivation) as opposed to sucrose "liking" (related to palatability) (Berridge, 1996; Kelley & Berridge, 2002). Results from the sucrose solution preference test did not reveal a significant difference in how much HF and LF groups "liked" sucrose. Both groups also consumed significantly more sucrose solution than water, suggesting that for both groups the sucrose solution was more palatable than water.
Early access to a sucrose-rich diet is also associated with impaired operant responding for sucrose (Frazier, Mason, Zhuang & Beeler, 2008) and saccharin (Vendruscolo, Gueye, Darnaudéry, Ahmed & Cador, 2010) and with conditioned place preference for a high sugar content US (Privitera et al., n.d.). Notably, adult rats maintained on sugar- rich diets for the same length of time as their pre and periadolescent counterparts did not demonstrate impaired performance on progressive ratio and fixed ratio schedules for saccharin (Vendruscolo et al., 2010). The "high sugar" diet used by Privitera and colleagues (Privitera et al., n.d.) was identical to our LFD. It is possible that our LFD reduced the reinforcing efficacy of sucrose but not as much as the HFD. Future studies should include a chow-fed control group to determine the effects of pre and periadolescent LFD exposure on adult responsiveness to sucrose.
Response Inhibition Capacity
Results suggest that response inhibition capacity was not significantly influenced by pre and peri-adolescent HFD exposure. TR model parameters corresponding to gamma-distributed IRTs (P, 6, w), which accounted for about 95% of IRTs, are not significantly sensitive to diet manipulations duringpre and periadolescence. Only the mean non-timed IRT, k, appears to be significantly sensitive to this manipulation. Based on IRT distributions, it was inferred that, when rats are delinquent on the timing task, they spend 3.5 to 5 s engaged in other activities. This delinquency time appears to be somewhat longer for HF rats, suggesting that HF-rat behavior is under a weaker control of the contingencies of reinforcement, an effect that may be related to reduced sustained attention (Sagvolden, Johansen, Aase, & Russell, 2005). HFD-associat-ed impairments in sustained attention have been shown in humans (Edwards et al., 2011; Holloway et al., 2011). Our findings encourage a more detailed examination of the long-term effects of pre and periadolescent HFD on sustained attention.
Rule Learning and Memory
In the VIDA task, alternation rule learning can be inferred from latencies on Go and No- Go trials following 0-s ITIs, when memory is not substantially taxed. Go and No-Go latencies following longer ITIs (7 s to 60 s) are indicative of short- and long-term memory capacity (Winocur, 1985, 1991), because Go and No-Go trials are differentially signaled only by the presence or absence of reinforcement before the ITI, and not by any contemporaneous stimulus. Reduced memory capacity is inferred from longer latencies in Go trials and shorter latencies in No-Go trials. Memory processes, conversely, are unlikely to control iterative responses emitted on Go and No-Go trials. Mean run IRTs—the mean time between consecutive responses—on Go trials are likely related to incentive motivation, whereas run IRTs on No-Go trials may be related to perseveration or reduced response inhibition capacity (Means, Walker, & Isaacson, 1970; Winocur & Hasher, 1999).
VIDA performance is sensitive to extended diet manipulations (Greenwood & Winocur, 1990). Go and No-Go trial latencies, analyzed as a ratio, have previously been shown to be sensitive to extended HFD access (Greenwood & Winocur, 1990, 2001; Winocur & Greenwood, 1999). Environmental enrichment mitigates HFD-associated impairments in VIDA performance (Winocur & Greenwood, 1999). The present study is the first to assess pre and periadolescent HFD effects on adult VIDA performance when HFD is discontinued during postadolescence and adulthood. The present study also verified the sensitivity of critical VIDA measures to environmental enrichment.
No measure of adult VIDA performance was significantly sensitive to a HFD that was restricted to pre and periadolescence. Thus, adult rule learning, memory capacity, incentive motivation, and response withholding abilities do not appear to be affected by a HFD during pre and periadolescence. The absence of a significant long-term effect of HFD on motivation appears to be inconsistent with inferences based on FMI latencies. This ostensive inconsistency, however, probably stems from differences in the processes that control latencies to a response that is not preceded by reinforcement and are reinforced after a delay (post-N in FMI), relative to the processes that control iterative responses that mostly preceded by reinforcement and are reinforced immediately (Go in VIDA). These inconsistent results suggest that appropriate experimental conditions—i.e., without the interference of preceding reinforcement and iterative responding—are required to observe the effects of pre and periadolescent HFD on incentive motivation.
Although VIDA performance was not significantly sensitive to pre and periadolescent HFD exposure, it was sensitive to environmental enrichment. Rule-learning, as expressed in longer No-Go vs. Go latencies following 0-s ITIs, was improved by environmental enrichment and declined once enrichment was removed (Fig. 5). Memory capacity, as expressed in longer No-Go vs. Go latencies following the 7-s ITI also appears to improve with enrichment (Fig. 5). Although this improvement seems to continue even after enrichment was removed, and extend to latencies following the 20-s ITI, the absence of a return to baseline may also reflect an effect of amount of VIDA training on performance. Nonetheless, our findings are consistent with prior reports of baseline VIDA performance and of its sensitivity to environmental enrichment (Winocur & Greenwood, 1999). The present results thus confirm that VIDA testing was carried out properly; the absence of significant pre and periado-lescent HFD effects on response inhibition capacity and memory is unlikely due to experimenter error.
Compared to the present study, prior studies that have demonstrated HFD effects on learning and memory have either exposed rats to HFD for a longer period of time (20 days here vs. 75-90 days in (Boitard et al., 2012; Greenwood & Winocur, 1990; Winocur & Greenwood, 1999) that extended throughout adolescence and well into adulthood. Relative to the present study, earlier studies initiated behavioral testing shortly after discontinuation of HFD exposure (8 (Boitard et al., 2012) or 14 days (Greenwood & Winocur, 1990; Winocur & Greenwood, 1999) vs. 80 days here), or have tested learning and memory using a substantially different paradigm (spatial discrimination learning in (Boitard et al., 2012); CPP in (Privitera et al., 2011)). Thus, our results suggest that, although a HFD restricted to pre and periadolescence has long-lasting effects on certain aspects of learning and memory (context-stimulus association learning (Privitera et al., 2011), but not on other aspects (response inhibition capacity in FMI, and short- and long-term memory in VIDA and object recognition task (Privitera et al., n.d.).
Conclusions
There are several lines of clinical and pre-clinical evidence demonstrating that exposure to a HFD has long-term detrimental effects on cognition. Past research suggests that context- stimulus and spatial learning are sensitive to HFD effects during pre and periadolescence and not during adulthood (Boitard et al., 2012; Privitera et al., 2011). The purpose of the present study was to determine if such selective effects extended to response inhibition capacity, rule-learning, and memory. Our results suggest that either the pre and periadolescence period is too short for a HFD to induce response-inhibition, rule-learning, or memory deficits, or maturation in the absence of a HFD rescued these deficits. Nonetheless, FMI data suggests that pre and periadoles-cent HFD may have long-term effects on reinforcer efficacy and sustained attention. Future research may test these hypotheses using behavioral paradigms explicitly designed to test for responsiveness to sucrose reinforcement and distractibility.
References
Berridge, K. C. (1996). Food reward: Brain substrates of wanting and liking. Neuroscience and Biobehavioral Reviews, 20(1), 1-25. [ Links ]
Bilbo, S. D., & Tsang, V. (2010). Enduring consequences of maternal obesity for brain inflammation and behavior of offspring. FASEB Journal : Official Publication of the Federation of American Societies for Experimental Biology, 24(6), 2104-2015. http://dx.doi.org/10.1096/fj.09-144014 [ Links ]
Boitard, C., Etchamendy, N., Sauvant, J., Aubert, A., Tronel, S., Marighetto, A., Layé, S., et al. (2012). Juvenile, but not adult exposure to high-fat diet impairs relational memory and hippocampal neurogenesis in mice. Hippocampus, 22(11), 2095-100. http://dx.doi.org/10.1002/hipo.22032 [ Links ]
Davis, J. F., Tracy, A. L., Schurdak, J. D., Tschöp, M. H., Lipton, J. W., Clegg, D. J., et al. (2008). Exposure to elevated levels of dietary fat attenuates psychostimulant reward and mesolimbic dopamine turnover in the rat. Behavioral Neuroscience, 122(6), 1257-1263. http://dx.doi.org/10.1037/a0013111 [ Links ]
Eckel, R. H., & Krauss, R. M. (1998). American heart association call to action: Obesity as a major risk factor for coronary heart disease. Circulation, 97(21), 2099-2100. http://dx.doi.org/10.1161/01.CIR.97.21.2099 [ Links ]
Edwards, L. M., Murray, A. J., Holloway, C. J., Carter, E. E., Kemp, G. J., Codreanu, I., Brooker, H., et al. (2011). Short-term consumption of a high-fat diet impairs whole-body efficiency and cognitive function in sedentary men. FASEb, 25(3), 1088-1096.http://dx.doi.org/10.1096/fj.10-171983 [ Links ]
Eskelinen, M. H., Ngandu, T., Helkala, E. -L., Tuomilehto, J., Nissinen, A., Soininen, H., & Kivipelto, M. (2008). Fat intake at midlife and cognitive impairment later in life: A population-based CAIDE study. International Journal of Geriatric Psychiatry, 23(7), 741-747. http://dx.doi.org/10.1002/gps.1969 [ Links ]
Fleshler, M., & Hoffman, H. S. (1962). A progression for generating variable-interval schedules. Journal of the Experimental Analysis of Behavior, 5, 529-530. http://dx.doi.org/10.1901/jeab.1962.5-529 [ Links ]
Frazier, C. R. M., Mason, P., Zhuang, X., & Beeler, J. A. (2008). Sucrose exposure in early life alters adult motivation and weight gain. PloS one, 3(9), e3221. http://dx.doi.org/10.1371/journal.pone.0003221 [ Links ]
Fung, T. T., Rimm, E. B., Spiegelman, D., Rifai, N., Tofler, G. H., Willett, W. C., & Hu, F. B. (2001). Association between dietary patterns and plasma biomarkers of obesity and cardiovascular disease risk. The American Journal of Clinical Nutrition, 73(1), 61-67. [ Links ]
Granholm, A. -C., Bimonte-Nelson, H. A, Moore, A. B., Nelson, M. E., Freeman, L. R., & Sambamurti, K. (2008). Effects of a saturated fat and high cholesterol diet on memory and hippocampal morphology in the middle-aged rat. Journal of Alzheimer's Disease, 14(2), 133-145. [ Links ]
Greenwood, C. E., & Winocur, G. (1990). Learning and memory impairment in rats fed a high saturated fat diet. Behavioral and Neural Biology, 53(1), 74-87. [ Links ]
Greenwood, C. E., & Winocur, G. (2001). Glucose treatment reduces memory deficits in young adult rats fed high-fat diets. Neurobiology of Learning and Memory, 75(2), 179-189. http://dx.doi.org/10.1006/nlme.2000.3964 [ Links ]
Greenwood, C. E., & Winocur, G. (2005). High-fat diets, insulin resistance and declining cognitive function. Neurobiology of Aging, 26 (Suppl. 1), 42-45. http://dx.doi.org/10.1016/j.neurobiolaging.2005.08.017 [ Links ]
Harzem, P., Lowe, C. F., & Priddle-Higson, P. J. (1978). Inhibition function of reinforcement: Magnitude effect on variable-interval schedules. Journal of the Eexperimental Analysis of Behavior, 30, 1-10. [ Links ]
Hill, J. C., Herbst, K., & Sanabria, F. (2012). Characterizing operant hyperactivity in the spontaneously hypertensive rat. Behavioral and Brain Functions, 8(5). http://dx.doi.org/10.1186/1744-9081-8-5 [ Links ]
Holloway, C. J., Cochlin, L. E., Emmanuel, Y., Murray, A., Codreanu, I., Edwards, L. M., Szmigielski, C., et al. (2011). A high-fat diet impairs cardiac high-energy phosphate metabolism and cognitive function in healthy human subjects. The American Journal of Clinical Nutrition, 93(4), 748-755. http://dx.doi.org/10.3945/ajcn.110.002758 [ Links ]
Kelley, A. E., & Berridge, K. C. (2002). The neuroscience of natural rewards: Relevance to addictive drugs. The Journal of Neuroscience : the Official Journal of the Society for Neuroscience, 22(9), 33061. http://dx.doi.org/20026361 [ Links ]
Kello, J. E. (1972). Schedules : Frustration effect on fixed-interval schedules: Frustration or inhibition?. Learning and Motivation, 3, 138-147. [ Links ]
Keselman, H. J., Algina, J., Lix, L. M., Wilcox, R. R., & Deering, K. N. (2008). A generally robust approach for testing hypotheses and setting confidence intervals for effect sizes. Psychological Methods, 13(2), 110-129. http://dx.doi.org/10.1037/1082-989X.13.2.110 [ Links ]
Kleen, J. K., Sitomer, M. T., Killeen, P. R., & Conrad, C. D. (2006). Chronic stress impairs spatial memory and motivation for reward without disrupting motor ability and motivation to explore. Behavioral Neuroscience, 120(4), 842-851. http://dx.doi.org/10.1037/0735-7044.120.4.842 [ Links ]
Kosari, S., Badoer, E., Nguyen, J. C. D., Killcross, A. S., & Jenkins, T. A. (2012). Effect ofwestern and high fat diets on memory and cholinergic measures in the rat. Behavioural Brain Research, 235(1), 98-103.http://dx.doi.org/10.1016/j.bbr.2012.07.017 [ Links ]
Luchsinger, J. A. (2002). Caloric intake and the risk of Alzheimer disease. Archives of Neurology, 59(8), 1258-1263. http://dx.doi.org/10.1001/archneur.59.8.1258 [ Links ]
McCullough, A. J. (2011). Epidemiology of the metabolic syndrome in the USA. Journal of Digestive Diseases, 12(5), 333-340. http://dx.doi.org/10.1111/j.1751-2980.2010.00469.x [ Links ]
McMillan, J. C. (1971). Percentage reinforcement of fixed-ratio and variable-interval performances. Journal of the Experimental Analysis of Behavior, 15(3), 297-302. [ Links ]
Means, L. W., Walker, D. W., & Isaacson, R. L. (1970). Facilitated single-alternation go, no-go acquisition following hippocampectomy in the rat. Journal of Comparative and Physiological Psychology, 72(2), 278-285. http://dx.doi.org/10.1037/h0029459 [ Links ]
Mechner, F., & Guevrekian, L. (1962).The effects of deprivation upon counting and timing in rats. Journal of Experimental Analysis of Behavior, 5(4), 463-466. [ Links ]
Mellon, R. C., Leak, T. M., Fairhurst, S., & Gibbon, J. (1995). Timing processes in the reinforcement-omission effect. Animal Learning & Behavior, 23(3), 286-296. http://dx.doi.org/10.3758/BF03198925 [ Links ]
Mika, A., Mazur, G. J., Hoffman, A. N., Talboom, J. S., Bimonte-Nelson, H. A., Sanabria, F., & Conrad, C. D. (2012). Chronic stress impairs prefrontal cortex-dependent response inhibition and spatial working memory. Behavioral Neuroscience, 126(5), 605-619. http://dx.doi.org/10.1037/a0029642 [ Links ]
Papini, M. R., & Hollingsworth, P. R. (1998). Role of nonreinforcement in the fixed-interval performance of pigeons. Psychonomic Bulletin & Review, 5(1), 84-90. [ Links ]
Pathan, A. R., Gaikwad, A. B., Viswanad, B., & Ramarao, P. (2008). Rosiglitazone attenuates the cognitive deficits induced by high fat diet feeding in rats. European Journal of Pharmacology, 589(1-3), 176-179. http://dx.doi.org/10.1016/j.ejphar.2008.06.016 [ Links ]
Pistell, P. J., Morrison, C. D., Gupta, S., Knight, A. G., Keller, J. N., Ingram, D. K., & Bruce- Keller, A. J. (2010). Cognitive impairment following high fat diet consumption is associated with brain inflammation. Journal of Neuroimmunology, 219(1-2), 25-32.http://dx.doi.org/10.1016/j.jneuroim.2009.11.010 [ Links ]
Priddle-Higson, P. J., Lowe, C. F., & Harzem, P. (1976). Afftereffects of Reinforcement on Variable-Ratio Schedules. Journal of the Experimental Analysis of Behavior, 25(3), 347-354. [ Links ]
Privitera, G. J., Zavala, A. R., Sanabria, F., & Sotak, K. L. (2011). High fat diet intake during pre and periadolescence impairs learning of a conditioned place preference in adulthood. Behavioral and Brain Functions, 7(1), 21. http://dx.doi.org/10.1186/1744-9081-7-21 [ Links ]
Privitera, G. J., Mayeaux, D. J., Schey, R.A., Lapp, H. E. (2013). Conditioned place preference deficits in adulthood following high fat and high sugar diet intake during pre- and periadolescence: A test of the specificity hypothesis. Journal of Behavioral and Brain Science, 3(7), 556-563. [ Links ]
Riccardi, G., Giacco, R., & Rivellese, A. A. (2004). Dietary fat, insulin sensitivity and the metabolic syndrome. Clinical Nutrition, 23(4), 447-456. http://dx.doi.org/10.1016/j.clnu.2004.02.006 [ Links ]
Rofey, D. L., Kolko, R. P., Iosif, A. -M., Silk, J. S., Bost, J. E., Feng, W., Szigethy, E. M., et al. (2009). A longitudinal study of childhood depression and anxiety in relation to weight gain. Child Psychiatry and Human Development, 40(4), 517-526. http://dx.doi.org/10.1007/s10578-009-0141-1 [ Links ]
Sagvolden, T., Johansen, E. B., Aase, H., & Russell, V. A. (2005). A dynamic developmental theory of attention-deficit/hyperactivity disorder (ADHD) predominantly hyperactive/impulsive and combined subtypes. The Behavioral and Brain Sciences, 28(3), 397-419. http://dx.doi.org/10.1017/S0140525X05000075 [ Links ]
Sanabria, F., & Killeen, P. R. (2008). Evidence for impulsivity in the spontaneously hypertensive rat drawn from complementary response-withholding tasks. Behavioral and Brain Functions, 4(7). http://dx.doi.org/10.1186/1744-9081-4-7 [ Links ]
Spear, L. P. (2000). The adolescent brain and age-related behavioral manifestations. Neuroscience and Biobehavioral Reviews, 24(4), 417-463. [ Links ]
Staddon, J. E., & Innis, N. K. (1966). An effect analogous to "frustration" on interval reinforcement schedules. Psychonomic Science, 4(7), 287-288. [ Links ]
Staddon, J. E. R., & Innis, N. K. (1969). Reinforcement omission on fixed-interval schedules1. Journal of the Experimental Analysis of Behavior, 12(5), 689-700. http://dx.doi.org/10.1901/jeab.1969.12-689 [ Links ]
Talton, L. E., Higa, J. J., & Staddon, J. E. (1999). Interval schedule performance in the goldfish Carassius Auratus. Behavioural Processes, 45(1-3), 193-206. http://dx.doi.org/10.1016/S0376-6357(99)00018-2 [ Links ]
Tozuka, Y., Kumon, M., Wada, E., Onodera, M., Mochizuki, H., & Wada, K. (2010). Maternal obesity impairs hippocampal BDNF production and spatial learning performance in young mouse offspring. Neurochemistry International, 57(3), 235-247. http://dx.doi.org/10.1016/j.neuint.2010.05.015 [ Links ]
Valladolid-Acebes, I., Stucchi, P., Cano, V., Fernández-Alfonso, M. S., Merino, B., Gil-Ortega, M., Fole, A., et al. (2011). High-fat diets impair spatial learning in the radial-arm maze in mice. Neurobiology of Learning and Memory, 95(1), 80-85. http://dx.doi.org/10.1016/j.nlm.2010.11.007 [ Links ]
Van Dam, R. M., Willett, W. C., Rimm, E. B., Stampfer, M. J., & Hu, F. B. (2002). Dietary fat and meat intake in relation to risk of type 2 diabetes in men. Diabetes Care, 25(3), 417-424. http://dx.doi.org/10.2337/diacare.25.3.417 [ Links ]
Vendruscolo, L. F., Gueye, A. B., Darnaudéry, M., Ahmed, S. H., & Cador, M. (2010). Sugar over-consumption during adolescence selectively alters motivation and reward function in adult rats. PloS one, 5(2), e9296. http://dx.doi.org/10.1371/journal.pone.0009296 [ Links ]
Vucetic, Z., Carlin, J. L., Totoki, K., & Reyes, T. M. (2012). Epigenetic dysregulation of the dopamine system in diet-induced obesity. Journal of Neurochemistry, 120(6), 891-898. http://dx.doi.org/10.1111/j.1471-4159.2012.07649.x [ Links ]
Vucetic, Z., Kimmel, J., & Reyes, T. M. (2011). Chronic high-fat diet drives postnatal epigenetic regulation of u-opioid receptor in the brain. Neuropsychopharmacology, 36(6), 1199-1206. http://dx.doi.org/10.1038/npp.2011.4 [ Links ]
Vucetic, Z., Kimmel, J., Totoki, K., Hollenbeck, E., & Reyes, T. M. (2010). Maternal high-fat diet alters methylation and gene expression of dopamine and opioid-related genes. Endocrinology, 151 (10), 4756-4764. http://dx.doi.org/10.1210/en.2010-0505 [ Links ]
Waring, M. E., & Lapane, K. L. (2008). Overweight in children and adolescents in relation to attention-deficit/hyperactivity disorder: results from a national sample. Pediatrics, 122(1), e1-6. http://dx.doi.org/10.1542/peds.2007-1955 [ Links ]
Wellman, P. J., Nation, J. R., & Davis, K. W. (2007). Impairment of acquisition of cocaine self- administration in rats maintained on a high-fat diet. Pharmacology, Biochemistry, and Behavior, 88(1), 89-93. http://dx.doi.org/10.1016/j.pbb.2007.07.008 [ Links ]
White, C. L., Pistell, P. J., Purpera, M. N., Gupta, S., Fernandez-Kim, S. -O., Hise, T. L., Keller, J. N., et al. (2009). Effects of high fat diet on Morris maze performance, oxidative stress, and inflammation in rats: contributions of maternal diet. Neurobiology of Disease, 35(1), 3-13. http://dx.doi.org/10.1016/j.nbd.2009.04.002 [ Links ]
Winocur, G. (1985). The hippocampus and thalamus: Their roles in short- and long-term memory and the effects of interference. Behavioural Brain Research, 16(2-3), 135-152. [ Links ]
Winocur, G. (1991). Functional dissociation of the hippocampus and prefrontal cortex in learning and memory. Psychobiology, 29(1), 11-20. [ Links ]
Winocur, G., & Greenwood, C. E. (1999). The effects of high fat diets and environmental influences on cognitive performance in rats. Behavioural Brain Research, 101(2), 153-161. [ Links ]
Winocur, G., & Hasher, L. (1999). Aging and time-of-day effects on cognition in rats. Behavioral Neuroscience, 113(5), 991-997. [ Links ]
Yu, H., Bi, Y., Ma, W., He, L., Yuan, L., Feng, J., & Xiao, R. (2010). Long-term effects of high lipid and high energy diet on serum lipid, brain fatty acid composition, and memory and learning ability in mice. International Journal of Developmental Neuroscience, 28(3), 271-276. http://dx.doi.org/10.1016/j.ijdevneu.2009.12.001 [ Links ]
Zeiler, M. D. (1972). Fixed-interval behavior: Effects of percentage reinforcement1. Journal of the Experimental Analysis of Behavior, 17(2), 177-189. http://dx.doi.org/10.1901/jeab.1972.17-177 [ Links ]