1. INTRODUCTION
Expansive soils are known all over the world for their poor volume stability. Construction on such soils results in disastrous effects on the structures built over them. Lime stabilization of expansive soils has been one of the most common solutions to mitigate the dangerous effects of ex pansive soils. However, there are also regions wherein the subsoil is subjected to extreme conditions. These include wetting-drying, freezing-thawing, high soil acidity and alka linity, high soil salinity, high sodic soil conditions, sulphate rich soils, and contaminated soils. In such conditions, the effectiveness of the stabilization used slowly reduces over an extended period. This may lead to a reduction in the du rability of the stabilized soil. James and Pandian [1] suggest that lime stabilized soils lose their effectiveness in extreme conditions, especially in tropical climatic conditions and in cold regions. In recent times, there have been several inves tigations by various researchers addressing the durability of the lime stabilized soils [2-7]. Based on these investiga tions, the researchers reported that the beneficial effect of lime in swell control and improvement of soil properties was partially lost due to multiple cycles of wetting and dry ing. Literature suggests that there have been investigations using industrial solid wastes as well as a few combinations of lime and industrial solid wastes for soil stabilization [8]. Over the years, few of the researchers have attempted to improve the performance of lime stabilization by combin ing it with pozzolans to improve its performance under such extreme conditions. A very early study conducted by Hoover et al. [9] attempted to study the performance of fly ash-lime stabilized mixes. Kampala et al. [10] studied the effect of the addition of fly ash on the wet-dry resistance of carbide lime stabilized soil. Sabat and Nanda [11] re searched the performance of marble dust modification of rice husk ash stabilized soil subjected cycles of wetting and drying. Yilmaz and Fidan [12] explored the effect of com binations of lime and perlite on the wetting-drying per formance of clayey soil. Harichane et al. [13] attempted to study the effect of lime-natural pozzolana on the durability of clayey soils subjected to multiple cycles of wetting and drying. These studies found that the addition of wastes/ pozzolans to lime during stabilization resulted in improved performance during wetting and drying cycles. However, despite the early attempt of using waste pozzolans with lime, studies on the utilization of combinations of lime and solid wastes for resistance against cycles of wetting and drying are still very minimal. Other researchers have also investigated the improvement of wetting-drying resistance of soils by the inclusion of fibres. Malekzadeh and Bilsel [14] studied the effect of polypropylene fibres on the mechani cal behaviour of expansive soils. They also investigated the effect of polypropylene fibre reinforcement on the swell and compressibility of expansive soils [15]. Phanikumar et al. [16] investigated the effect of wetting and drying cycles on the heave behaviour of fibre reinforced expansive soils. Greeshma and Joseph [17] included rice straw fibres into Kuttanad expansive clay to investigate its performance. Others went one step further by combining lime/cement with fibres to improve its durability performance. Prajisha and Ajitha [18] explored the performance of banana fibre reinforced lime stabilized soil subjected to cycles of wetting and drying. Anggraini et al. c considered the reinforcement benefits of nano modified coir fibre in lime treated marine clay. Sukontasukkul and Jamsawang [20] adopted metallic steel fibres for the strengthening of deep soil-cement col umns. Fatahi et al. [21] adopted polypropylene and steel fibres to improve the performance of cement stabilized silty clay. Correia et al. [22] investigated the effect of stabiliza tion of soil with a combination of cement, ground granulat ed blast furnace slag and steel fibres. The addition of fibres to reinforce stabilized soils provided the advantage of mi cro-reinforcement by the fibres in resisting crack propaga tion when stabilized specimens were subjected to durabil ity conditions like wetting and drying. Each fibre type has its own advantages and disadvantages. Natural fibres are renewable and environment friendly. But there is always a question over their durability when exposed to water and require treatment to ensure increased performance. Artifi cial fibres like polypropylene fibres are readily available in huge quantities and durable. But, they are comparatively costly and have issues of floating in water during their mix ing with soil. They also require considerable care and effort to ensure a uniform and intimate mix with the soil. Metallic fibres like steel fibres have the issue of corrosion over an extended period of time. They are however, comparatively stronger and stiffer than other fibres. Polypropylene fibres have been the most common type of fibre reinforcement considered by researchers. Other types of artificial fibres like nylon and polyester as well as natural fibres like banana and coir have also been investigated. But, there seems to be very few investigations considering metallic fibres in soil micro-reinforcement. Thus, in summary, it can be seen that researchers have attempted to improve the durability of lime stabilized soil by addition of industrial wastes/fibres. However, it was found that very few investigations have fo cussed on the combinations of lime, industrial wastes and fibres for improving the durability performance like the one done by Muntohar and Khasanah [23]. They examined the performance of clayey soil stabilized with lime and rice husk ash reinforced with polypropylene waste fibres sub jected to cycles of wetting and drying. There still exists a great potential for identification of optimal combinations of lime, wastes and fibres. This investigation attempted to address this gap in literature by considering a combination of lime, steel slag and copper filaments as well as poly propylene fibres in the stabilization and durability perfor mance of an expansive soil subjected to alternate cycles of wetting and drying.
2. MATERIALS USED
The various materials used in the present investigation were the virgin expansive soil, high-quality industrial-grade lime, steel slag, copper filaments and polypropylene fibres. Lime was used as the primary binder, whereas steel slag was used as an auxiliary additive. The copper filaments and polypropylene fibres were used as random micro reinforcement.
The virgin expansive soil adopted in this investigation was collected from the banks of Thaiyur lake, near Kalavakkam, Tamil Nadu, India. The soil sample was prepared fol lowing the Bureau of Indian Standards (BIS) code [24]. The soil sample was subjected to various geotechnical tests in the laboratory according to the various codes of BIS. The properties of soil are tabulated in Table I. High-quality in dustrial-grade hydrated lime was sourced from M/s. Shiyal Chemicals, Chennai. No specific preparation methods were adopted for lime, which was used as supplied in the com mercial packets. Steel slag is a by-product resulting from the manufacturing process of steel. It is obtained during the separation of molten steel from the impurities in the furnace in a liquid state. It is a complex solution of silicates and oxides, which solidifies into steel slag on cooling. Steel slag was obtained from Jindal Steel Works, Salem. Its specif ic gravity was found to be 2.67. The steel slag was prepared by crushing and sieving it through 75-micron sieve. The fin er fraction which passed through the 75-micron sieve was used in the investigation. Fig. 1 shows the various materials after preparation.
Copper filaments were adopted as fibre reinforcement as a replacement for steel fibres adopted by certain inves tigators in soil stabilization. Copper was bought as wires from a motor winding shop. It had a diameter of 0.14mm. It was cut into filaments of 10mm length based on which the aspect ratio was approximately 77. Polypropylene fibres were used in the investigation to obtain a comparative performance evaluation for copper filament reinforced soil. The polypropylene fibres were purchased from a commer cial retailer in Chennai. It had a length of 12mm and a di ameter of 0.025mm, based on which its aspect ratio came out to be 480.
The optimization of steel slag and copper filaments contents was also done by conducting UCS tests. Fig. 4 and Fig. 5 show the results of the UCS tests for determination of optimal content of steel slag and copper filaments. It can be seen that increase in steel slag content results in a reduction of the strength of the specimens. Therefore, the steel slag content with the minimum loss in strength was considered to be the optimal dosage. The incorporation of steel slag in the stabilization process is for the utilization of solid waste for effective waste management.
The optimization of copper filament content for fibre reinforcement of the specimens was performed using a similar procedure. The soil stabilized with 3% lime was amended with copper filaments starting from 0.5 % in in crements of 0.5 % up to 2 %, Fig. 5 shows the variation in the strength of the specimens amended with different pro portions of copper filaments by the weight of the soil. From the graph, it can be seen that there is an initial reduction in strength of the stabilized specimen reinforced with 0.5 % copper filaments. Further increase in the filament content to 1% leads to a rise in strength of the specimen. However, no further variations in the strengths were noticed for high er contents of copper filaments. Hence, it was concluded that a minimum of 1% copper filaments was the optimal dosage that resulted in the least loss in strength, Fig. 5 also shows the optimal copper filament content for 3% lime and 5% steel slag stabilized soil. It can be seen that the optimal copper filament content for steel slag amended lime sta bilized soil was also 1%. Thus, irrespective of the steel slag amendment, the optimum copper filament content for re inforcing the soil came out to be 1%, Fatahi et al. [21] iden tified 10% of steel fibre content developed the maximum strength for cement-treated silty clay soil. Sukontasukkul and Jamsawang [20] found that 1% of steel fibres resulted in the maximum flexural strength of fibre reinforced deep soil-cement column. This was irrespective of long or short fibre steel adopted in the study. The optimal dosage of polypropylene fibres (0.3%) was adopted from the work done by Jiang et al. [37], who had done extensive work on optimizing the fibre content based on aspect ratio and dosage. Muntohar and Khasanah [23] had adopted a similar polypropylene waste fibre content of 0.4%.
3. METHODS
The experimental methodology followed in this investiga tion consisted of the following stages: Collection and prepara tion of materials, characterization of materials, determination of initial consumption of lime (ICL), identification of optimum steel slag content, identification of optimum fibre content, preparation of specimens, simulation of wetting and drying and specimen testing. Each of the stages has been described in detail in the following subsections.
3.1 Collection and Preparation of Materials
The soil sample was collected from the source and transported to the laboratory. In the laboratory, it was crushed and pulverized into smaller fractions using a hand rammer. It was then sieved through various sieves as per requirement for various tests done following the codes of BIS. The lime was used as supplied by the manufacturer. Steel slag was crushed and sieved through 75-micron sieve and the fine powder passing through the sieve was used in the study. Copper filaments were cut to an aspect ratio of 77 from long wires. Polypropylene fibres were used as supplied by the retailer.
3.2 Characterization of Materials
The soil obtained for the investigation was subjected to various geotechnical properties as per different codes of BIS. The various properties evaluated include liquid and plastic limit [25], shrinkage limit [26], specific gravity [27], grain size distribution [28], compaction [29], unconfined compression strength (UCS) [30] and free swell index [31]. The soil was then classified based on the guidelines of BIS code [32]. Steel slag was subjected to a specific gravity test following the same procedure that was adopted for soil.
3.3 Determination of Initial Consumption of Lime
Based on the existing literature, for the soil under inves tigation, the ICL value was determined from the Eades and Grim pH test [33] based on the ASTM code D6276 [34].
3.4 Identification of Optimum Steel Slag Content
To determine the optimum steel slag content of the giv en soil sample, UCS tests were performed on the soil sam ples stabilized with lime (ICL). All UCS samples for deter mination of optimum steel slag content were compacted to MDD and OMC of the stabilized soil obtained from mini compaction tests [29] done based on the BIS code [35] pro cedure for stabilized soil. A calculated quantity of dry soil was weighed out. To this, minimum lime content and one trial content of steel slag by weight of dry soil was added in dry condition and manually mixed to achieve a uniform dry mix. The quantities of lime, steel slag and soil were calculat ed such that the final weight of the dry mix was equal to the weight required for achieving the dry density of the UCS sample, which in the present case was fixed as the MDD of the lime stabilized soil. To this uniform dry mix, water con tent equal to the OMC of the stabilized soil was sprinkled in stages, combined with manual mixing until a uniform wet mix was achieved. This mix was packed into the con stant volume split mould of internal diameter, 38 mm and height, 76 mm, and compacted by static loading. After the samples were cast, they were immediately demoulded and air-cured in sealed polythene covers to prevent loss of moisture. Three specimens were cast for each combination of steel slag adopted for stabilization. The steel slag content was varied from 5% to 20% in increments of 5% by weight of dry soil for determining the optimum content. The sam ples were cured for 3 days before they were tested. James and Pandian [36] had adopted a curing period of 2 days for identifying the optimum lime content in their investigation. The combination that gave the maximum strength/minimum loss in strength was adopted as the optimum content.
3.5 Identification of Optimum Fibres Content
The optimum fibre content was also determined using the same procedure as adopted for the determination of optimum steel slag content. The soil specimens of 38 mm diameter and 76 mm height were prepared by mixing the minimum lime content and optimum steel slag content. In this mix, the fibre content was varied from 0.5% to 2% in increments of 0.5%. Three samples were prepared for each combination and cured for 3 days. The combination that produced the maximum strength/minimum loss in strength was considered as the optimum fibre content. Polypropylene fibres being one of the most commonly ad opted fibres, its optimum fibre content was adopted from the literature [37], for comparison with the performance of copper filaments.
3.6 Preparation of Test Specimens
The UCS samples were prepared in a constant volume split mould of dimensions 38 mm x 76 mm, de-moulded immediately and placed inside a sealable polythene cov er for curing. Three samples were prepared for testing each of the combinations. Figure 2 shows the preparation and testing of a UCS sample. Calculated quantities of soil, lime, steel slag and filaments/fibres were packed in sealed polythene covers for sample preparation. A steel UCS split mould of height 76 mm and internal diameter 38 mm was prepared by lubricating its interior with oil. The materials were emptied on to a mixing pan and mixed in dry state manually. The required quantity of water was sprinkled on to it and mixed thoroughly to obtain a uniform wet mix. The mixture was then packed into a steel UCS mould in layers with gentle compaction provided by a compaction plug. This was repeated until the entire wet mix was packed into the split mould. The mould was then plugged at both ends using the end plugs. It was loaded on to a universal sample compacter cum extruder and compacted by apply ing static pressure. Care was taken to introduce the layers of wet mix alternatively from either end of the split mould and compacted from the corresponding end to ensure uniform compaction of the prepared UCS sample [38]. The sample was then ejected after opening the split mould and applying gentle pressure using the compaction plug. The samples were cured at room temperature of 30oC +/- 2oC in sealed polythene covers for 14 days. Fig. 2 shows the prepa ration of samples for the investigation.
3.7 Simulation of Wetting and Drying
The samples were subjected to alternate cycles of wet ting and drying by simulating the conditions of wetting and drying. A wet-dry cycle consisted of two phases: A 24 hours wetting phase wherein the samples were covered by soaking wet cotton and a 24 hours drying phase at open-air room conditions. All specimens were subjected to 1, 2 and 3 cycles of wetting and drying after they were cured for 14 days. Va rious researchers have adopted a different number of cycles for their work. Phanikumar et al. [16] adopted three cycles of wetting and drying whereas Muntohar and Khasanah [23] adopted four cycles. Anggraini et al. [19] adopted five cycles of wetting and drying. Yilmaz and Fidan [12] had adopted 12 cycles of wetting and drying in their investigation.
3.8 Specimen Testing
After the end of the set period of curing and cycles of wetting and drying, the samples were loaded axially until failure in UCS loading frame of 50kN capacity. The rate of strain adopted for the test was 0.625 mm/min. The various shortlisted combinations that were evaluated for the wet ting and drying resistance performance is shown in Table II.
4. RESULTS AND DISCUSSION
An expansive soil was stabilized using a combination of lime, steel slag and fibres for improving its strength. It was then subjected to different cycles of wetting and drying to study its durability performance. The results of the investi gation are discussed in the following subsections.
4.1 Optimization of Lime, Steel Slag and Fibre Content
The ICL is the minimum lime content required to raise the pH to 12.4. In highly alkaline conditions, the soil silica and alumina dissolve and react with the calcium from lime forming stabilization reaction products. Thus, high pH val ue is essential for stabilization reactions to proceed. The ICL was determined using the Eades and Grim pH test [33] based on ASTM D6276 [34]. The ICL for the soil under inves tigation was found to be 3% as shown in Fig. 3.
4.2 Effect of Stabilization and Reinforcement on the Strength
Figure 6, shows the effect of the amendment of 3% lime with the various optimal doses of additives, viz. 5% steel slag, 1% CF and 0.3% polypropylene fibres in different com binations. It can be seen that all the optimal amendments have resulted only in a positive gain in strength due to the modification. The polypropylene fibre modification devel oped the least gain in strength when compared to all com binations. On the other hand, the samples reinforced with copper filaments produced the maximum gain in strength under normal conditions. The result was in contrast to the results obtained by Fatahi et al. [21] and Sukontasukkul and Jamsawang [20]. Both the studies reported polypropylene fibres developing better strength when compared to metal lic steel fibres. This may be because the polypropylene fibre dosage for the soil under investigation was not identified but rather chosen from literature for comparison. Introduc tion of steel slag resulted in a slight reduction in strength in the case of copper filament reinforcement whereas it re sulted in an increase in strength in the case of polypropyl ene reinforced lime stabilized soil. Thus, different reinforce ment materials produce different degrees of improvement and modification of lime stabilization. The same can also be said for steel slag amendment of lime stabilization.
4.3 Effect of Wetting and Drying on the Strength of Stabilized Soil
The effect of wetting and drying cycles on the compres sive strength of the various specimen combinations were studied by conducting UCS tests on the samples after sub jecting them to multiple cycles of wetting and drying.
4.3.1 Effect of Fibres on Durability of Lime Stabilized Soil
Figure 7, shows the effect of fibre reinforcement on the wetting and drying resistance of pure lime stabilized soil. For comparison of performance, the wetting and drying resistance of pure lime stabilized soil without fibre rein forcement (control) has also been shown in the figure. The strength of the lime stabilized soil increases from 204.35 kPa to 329.31 kPa after the first cycle of wetting and dry ing. The increase in strength of the stabilized soil after the first cycle of wetting and drying may be indicative of a deficiency in water content during the moulding process. This deficiency may have been fulfilled during the first im mersion cycle leading to an increase in the strength. The lime stabilized soil loses strength on a further increase in the number of wetting and drying cycles. The strength of the stabilized soil decreases to 161.15 kPa for three cycles of wetting and drying. Anggraini et al. [19] also reported a reduction in the strength of lime treated marine clay with an increase in cycles of wetting and drying. In the case of copper filament reinforced lime stabilized soil as well, there is an increase in the strength of the stabilized soil after the first cycle of wetting and drying. Again, this may be indic ative of a deficiency in water content, fulfilled by the wet ting stage of the cycle resulting in improved strength. The strength of the copper filament reinforced lime stabilized soil increases from 270.58 kPa to 325.82 kPa after the first cycle of wetting and drying. On the subsequent increase in the number of cycles, there is a reduction in the strength of the specimens. However, even after three cycles of wet ting and drying, the strength of the specimens has not low ered below that of the control specimens. The reduction in strength seems to have stabilized after the second cycle with no big difference in the strength of the specimens subjected to two and three cycles of wetting and drying. In general, it can be seen that the strength of the specimens subjected to cycles of wetting and drying is higher than the control specimens. This indicates a better resistance to wet ting and drying due to the reinforcement of the specimens with copper filaments.
In the figure 7, is clear that the trends of polypropylene reinforced lime stabilized soil are similar to that of pure lime stabilized soil. The major difference is an increase in strength up to two cycles of wetting and drying beyond which there is a reduction in strength of the specimens. Muntohar and Khasanah [23] reported an increase in the strength of the specimens stabilized with a combination of lime-rice husk ash-polypropylene fibres up to three cycles of wetting drying. Anggraini et al. [19] reported an increase in the strength of coir fibre reinforced lime treated marine clay up to five cycles of wetting and drying. Muntohar and Khasanah [23] also report that samples prepared with mois ture content lesser than optimum (dry side of optimum) have a higher strength development. In the present study as well, polypropylene fibre reinforced lime-steel slag sta bilized soil specimens had an increase in the strength for more than one cycle of wetting and drying. The presence of the fibres may have resulted in improved hydration of the soil specimens while resisting the spread of cracking. Anggraini et al. [19] state that the presence of the fibres in creased the number of wetting cycles by which sufficient moisture for hydration was supplied, resulting in improved strength of the specimens. Muntohar and Khasanah [23] attribute the increase in strength with wetting and drying cycles to two reasons. First, the increase in the duration of chemical reactions during wetting and drying cycles and second, the increase in the quantum of cementitious com pounds during wetting and drying cycles.
4.3.2 Effect of Fibres and Steel Slag on Durability of Lime Stabilized Soil
The effect of fibre reinforcement was also studied on lime stabilized soil modified with optimal steel slag con tent. The procedure adopted was the same as for fibre re inforced pure lime stabilized soil, Fig. 7 shows the effect of wetting and drying cycles on lime-steel slag stabilized soil. The inclusion of steel slag in the stabilization process results in a slight modification in the response of the sta bilized specimens subjected to wetting and drying cycles.
It can be seen that the lime-steel slag stabilized specimens modified with 1% copper filaments result in better resis tance to wetting and drying up to two cycles. Further in crease in the cycles results in a drastic reduction in strength of the stabilized specimen. Thus, the inclusion of steel slag in the stabilization process results in strength benefits only up to two cycles of wetting and drying.
Figure 8 also shows the effect of wetting and drying on the 0.3% polypropylene reinforced lime-steel slag sta bilized soil. The inclusion of steel slag in the stabilization process results in a similar trend as for copper filament re inforced lime-steel slag stabilized soil. There is a significant benefit in the resistance to wetting and drying cycles until two cycles. Beyond two cycles, there is a drastic reduction in the strength of the stabilized soil. Thus, in both the cases of copper filaments and polypropylene fibres, the benefi cial effect of steel slag was obtained only up to two cycles of wetting and drying. The introduction of steel slag as a pozzolan in the mix could not extend the resistance to a higher number of wetting and drying cycles. Hasan et al. [39] found that the introduction of bagasse ash in lime sta bilization resulted in the stabilized composite becoming more durable when subjected to ten cycles of wetting and drying. A similar objective attempted with steel slag in the present investigation could not be achieved.
4.3.3 A Comparative Discussion
To understand the extent of strength development of fibre reinforced lime stabilized soil subjected to alternate cycles of wetting and drying, a comparison with similar stu dies was attempted. Two studies were identified for compa rison with the present study viz. the work done by Anggraini et al. [19] and Muntohar and Khasanah [23]. These two were selected because the former adopted a combination of lime and fibres and the latter adopted a combination of lime, waste and fibres. However, there are inherent limita tions of such a comparison. At the outset, the obvious di fferences in the type of soil, type of binder/stabilizer and its content, type of auxiliary additive and its content and lastly, the type of fibre and its contents do not allow a di rect one to one comparison. Therefore, this comparison is a superficial one. Secondly, both the studies subjected these combinations to multiple cycles of wetting and drying. But, the comparison was limited to only 3 cycles of wetting and drying adopted in the present study. Lastly, other studies with a combination of lime and metallic fibres subject ed to wetting and drying could not be found and hence, were not included. Table III gives a brief overview of the combinations used in the investigations. The combinations shown in the table have been modified according to the same naming convention used in the present study. Only the optimal dosage combinations from the present study have been shown in Table III.
Table III OVERVIEW OF STABILIZER AND FIBRE COMBINATIONS OF THE TWO INVESTIGATIONS
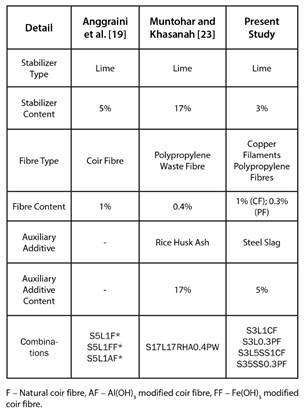
Source: The authors
Figure 9 shows a graph between the residual strength index (RSI) and no. of cycles. RSI may be defined as the ratio of the UCS of the samples subjected to wetting and drying cycles to that of the control samples (no wetting and drying) [40]. The same was also adopted by Anggraini et al. [19] as well as Muntohar and Khasanah [23]. Figure 9 represents the data under two categories. The curves for fibre reinforced lime stabilized soil is represented by solid lines and the curves for fibre reinforced, waste amended lime stabilized soil is represented by dotted lines. At the outset, it can be seen that RSI curves of Anggraini et al. [19] are different from that of the present study. S5L1F and S5L1FF are very similar to each other in terms of wet-dry resistance represented by the RSI curves. S5L1AF differen tiates itself after the first cycle of wetting and drying and shows improved resistance compared to the other two. S3L1CF of the present study is different from them in the fact that it shows very good wet-dry resistance for the first cycle but that resistance reduces thereafter. But all these combinations lie within the RSI range of 1 to 1.2. The com bination S3L0.3PF of the present study, however, shows a vastly different wet-dry resistance as seen from its RSI curve. Its RSI goes as high as 1.63 at cycle two and then drastically drops to 0.87. Comparing the work of Muntohar and Khasanah [23] and the present study, there is a vast difference between their corresponding RSI curves. The wet-dry resistance of the former shows a steady im provement with the number of cycles (considered up to three), with RSI value up to 2.81. In comparison, both the combinations in the present study show improved wet dry resistance only up to two cycles of wetting and drying. This may be due to a significantly high lime content adop ted by Muntohar and Khasanah [23], in their investigation compared to the present study. Secondly, the difference in auxiliary additives and their contents in both investiga tions could have also played a significant role.
4.3.4 Percentage Strength Change Analysis
To better understand the effect of wetting and drying on the strength of the stabilized soil samples, a percenta ge strength change analysis was performed. This analysis was performed from two perspectives. The first perspecti ve was the change in strength with the number of cycles of wetting and drying. The second perspective was the strength change from that of pure lime stabilized soil for corresponding cycles of wetting and drying, Fig. 10 shows the strength change with the number of cycles of wetting and drying. It can be seen that all the combinations, gain strength after the first cycle of wetting and drying when compared to the control specimens. However, the com binations S3L and S3L0.3PF gave the maximum strength gain of 61% and 51% respectively. For the second cycle as well all the combinations produced positive strength gain. But, the combinations of polypropylene reinforced stabilized soil (S3L0.3PF and S3L5SS0.3PF) produced the maximum strength gain of 63% and 60% respectively. Af ter three cycles of wetting and drying, all the combina tions resulted in a loss in strength barring the combination reinforced with copper filaments (S3L1CF). It still had a strength of around 9% higher than the control specimens, though marginally lesser than the 9.7% gain achieved af ter the second cycle of wetting and drying. Thus, it can be seen that copper filament reinforced lime stabilized soil (S3L1CF) was the most durable of all the stabilized soil combinations. Figure 11 shows the percentage strength change from pure lime stabilized soil for corresponding cycles of wetting and drying.
It can be seen from the figure that all the combinations have given positive strength benefits when compared to the pure lime stabilized soil, when not subjected to wetting and drying cycles (0 Cycle). After the first cycle of wetting and drying, pure lime stabilized soil was better than all the combinations as there was a strength loss when compared to lime stabilized soil. But, copper filament (S3L1CF) and polypropylene fibre (S3L0.3PF) reinforced lime stabilized soil specimens were more durable compared to the steel slag amended combinations. Their loss in strengths were around 1% and 2.5% respectively. After two cycles of wet ting and drying, all the combinations were better than pure lime stabilized soil. However, in this case, the polypropylene fibre reinforced combinations (S3L0.3PF and S3L5SS0.3PF) were better than pure lime stabilized soil (by 29% and 47% respectively). After three cycles of wetting and drying, it is evident that the steel slag amendment did not perform as expected, losing strength. But, on the other hand, the fibre reinforcement of lime stabilized soil resulted in good resis tance to wetting-drying. The copper filament reinforced specimen (S3L1CF) and polypropylene fibre reinforced specimen (S3L0.3PF) performed 83% and 15% better than the pure lime stabilized combination (S3L), respectively. Thus, fibre reinforced lime stabilized soil performed better than fibre reinforced lime-steel slag stabilized soil, under conditions of wetting and drying. The choice of including steel slag in the stabilization can be decided based on the extent of wet-dry cycles and the location of stabilization. However, extensive studies with a greater number of wet dry cycles need to be conducted before an effective combi nation can be suggested for such field conditions.
5. CONCLUSION
The investigation involved studying the resistance of va rious combinations of lime, fibres and steel slag stabilized soil to alternate wet-dry cycles. Steel slag was adopted as the au xiliary additive whereas copper filaments and polypropylene fibres were used as reinforcement. The UCS of the specimens exposed to 1, 2 and 3 cycles of wetting and drying was eva luated to study the resistance to the loss in strength against control specimens. Based on the experimental investigations carried out on various combinations of lime, steel slag and fibres, the following points can be concluded.
The soil under investigation was found to be an expan sive soil, classified as high plastic clay. This soil required a minimum of 3% of lime for modification of its properties. The optimal steel slag content was found to be 5%. The optimal dosage of copper filaments required for maximum strength gain/minimum strength loss was found to be 1%. This 1% filament content was found to be the optimal dos age irrespective of whether the soil was stabilized using lime or combinations of lime and steel slag.
All combinations of additives and fibres identified to be optimal dosages resulted in UCS higher than pure lime stabilized soil. This indicates that the introduction of steel slag as an auxiliary additive in combination with fibre re inforcement improves lime stabilization. Thus, steel slag can be effectively used in soil stabilization applications, but definitely along with fibre reinforcement.
Irrespective of combinations, all specimens investigated increased in strength after wetting and drying, which may be indicative of a water deficiency in the specimens. It can be su ggested that for soils subjected to wetting and drying cycles, stabilization on the dry of optimum water content can prove to be beneficial. However, more detailed investigations need to conducted to confirm the veracity of the inference.
Introduction of auxiliary additive and fibre reinforce ment resulted in improved resistance to the loss in strength when compared to pure lime stabilized soil, irrespective of the combination of additive and fibre adopted. However, the beneficial effect of the additive could be seen only till two cycles of wetting and drying. The fibre reinforcement of lime stabilized soil resulted in good resistance to wetting and drying cycles even up to three cycles. Thus, the choice of introducing an auxiliary additive like steel slag can be de cided based upon the expected severity of the cyclic condi tions of wetting and drying.
Based on the investigation, it can finally be concluded that copper filament reinforcement of lime stabilized soil was found to be more effective in resisting wetting and dry ing cycles. This can open up an avenue for potential reuse of waste copper filaments from old wiring as soil reinforce ment. To conclude, waste copper filaments can be effec tively used in soil stabilization activities, especially under conditions of wetting and drying.
Future investigations are needed with a higher number of wetting and drying cycles for identifying an effective and durable combination of stabilizers and fibres for resisting extreme field conditions.