INTRODUCTION
The coconut is a fruit with high nutritional and medicinal value of global importance, with impact in more than 90 countries and more than 80 million people [1]. The pulp in a ripe fruit contains approximately 35-64% water, 25-44% oil, 9-14% carbohydrates, 5 mg vitamin C/100g, 4,1 mg galic acid/100g [1]. Storage studies of coconut powder and/or derivatives are poorly reported; Jena and Das (2006) [2] y (2012) [3], carried out predictions of the shelf life of the coconut aqueous extract powder obtained by vacuum drying in accelerated storage conditions at relative humidity of 90±1% and 38±2°C, considering the criteria for the start of caking or formation of lumps, and lipid oxidation. In the prediction based on the oxidation of lipids, relationships were established between the O2 absorption rate, the oxygen concentration inside the package, the volume of O2 absorbed and the peroxide index (IP). An IP of 10 mEq. O2/kg fat was the threshold limit for oxidative rancidity. The properties of powdered food during storage are affected by several factors: temperature, relative humidity, light, O2, packaging, time, among others [4,5,6] highlighting the deterioration in its nutritional value [7,8], in fluency, agglomeration [9,10], and color [11,12,7]. Several research have reported an important effect during storage on the properties of avocado powder [13], pink guava [11], biofortified cane with kefir granules [14], uchuva [15], skim milk [10], and milk protein [9].
In general, powder products obtained by spray drying reach a non-thermodynamic, amorphous (vitreous) state; however, during storage they present changes of the amorphous state (vítreous ( rubbery) and at the same time in their properties, due to the effect of dependent variables: temperature, time and packaging; a higher storage temperature and a packing with greater water vapor permeability increase the moisture gain, the aw and the degree of agglomeration of the particles, decreasing the glass transition temperature (Tg) of the product [11,4]. The agglomeration of the powder depends on its composition; that is, of the superficial forces of the particles: van der Waals forces (attractive) and electrostatic (repulsive) forces [11,16], being favored in fruits for its sugar content [16]. The agglomeration is the result of the plasticization of the concentrated compounds, which is enhanced by the adsorption of water, which generates a greater mobility of the water in the structure and contributes to a greater rate of deterioration. The determination of the best storage condition is essential to preserve the quality of the product and its shelf life [13,4,3]. The aim of this research was to evaluate the stability of the quality attributes of spray dried coconut powder fortified with Ca and vitamins C, D3 and E (PC+FAC) and the effect of the type of treatment (temperature-packaging) and the storage time (tA).
METHOD
Materials
The coconut powder added with calcium and vitamins C, D3 and E (PC+FAC) was obtained by spray drying according to the methodology described by Lucas-Aguirre et al. 2018 [17]. Cocconut variety Enano Malayo (manila) was used and as fortifying agents were used: calcium citrate, 99,5% ascorbic acid, Vitamin D3 500,000 IU (cholecalcipherol), vitamin E (DL-α-tocopherol acetate 50% USP GRADE), in addition to the additives: maltodextrin (MD) with dextrose equivalent 18-20, whey (Instant WPC 80), Nicle, xanthan gum (GXantan) and terbutilhydroquinone.
Caracterization of PC+FAC
Moisture (X w ). Official method of AOAC 930.15/2016 [18].
Water activity (a w ). It was determined with a dew point hygrometer at 25ºC (Aqualab serie 3TE, Decagon, Devices, Pullman, WA, USA) [19].
Solubility (S). Method, described by Estrada, (2016) [20] and Lucas-Aguirre et al. (2018) [17].
Peroxide index (IP). Method described by Lucas-Aguirre et al. (2018) [17].
Total phenolic compounds (TPC) (mg galic acid/g). Methodology described by Zorić et al. (2017) [21].
Antioxidant activity. It was determined by means of two methodologies: 1) capture activity of free radicals DPPH and ABTS (mg trolox / g) described its extraction by Sridhar and Linton-Charles, (2019) [22] and quantification by Casagrande et al. (2018), [23].
Particle size. The D10, D50 and D90 percentiles were determined with the Mastersizer 3000 (Malvern Instrument Ltd., Worcestershire, UK).
Color. The CIE-La*b* coordinates were determined with an X-Rite spectrophotometer SP62, illuminant D65, observer 10° and specular component excluded [17].
Microestructural analysis. It was performed by SEM with a scanning electron microscope (Jeol 5910LV) at 15 Kv [17]. The micrographs were performed at 0 and 180 days of storage and the best storage conditions.
Extraction and quantification of vitamins and calcium. Vitamin C was extracted according to the methodology of Silva et al. (2018) [24] and the vitamins D3 and E according to the methodology of Ruiz-Ruiz et al. (2017) [13], modified by the inclusion of an ultrasound treatment (40 kHz) to the samples treated with hexane, for 20 minutes. The quantification of vitamins D3 and E was carried out by HPLC (Shimatzu Prominence 20A) according to the methodology described by Ruiz-Ruiz et al. (2017) [13], with wavelengths of 265 and 285 nm, respectively. Vitamin C was quantified by HPLC according to the methodology described by Hernández-Sandoval et al. (2014) [15^, at a wavelength of 244 nm and an injection volume of 5 μL and the quantification of calcium was performed by spectrophotometry by atomic absorption with flame [25].
Experimental design
A completely randomized factorial design was used with factors: treatment (temperature-packaging) and time (tA) and the dependent variables: Xw, aw, S, L*, a*, b*, IP, retention of vitamins C, D3, E and calcium (R-Vit.C, R-Vit.D3, R-Vit.E, R-Ca respectively), retention of TPC (R-TPC), retention of antioxidant activity (R-ABTS and R-DPPH), and microstructural analysis (D10, D50, D90). The samples were packed in multilayer bags (Alico S.A), thickness of laminated film (PET) 12 μm, with aluminum foil 8 μm and a polyethylene sealing layer 100 μm, weight of 136,54 g/m2, with vapor permeability of water <1 cm3/ (m2 24 h atm) and to O2 <1 c m3/(m2 24 h atm) and stored in climatic chambers with relative humidity: 65%, temperatures: 15, 25, and 35°C and packed: atmosphere of N2 and environment (Amb). The treatments were: 15-N2, 15-Amb, 25-N2, 25-Amb, 35-N2 y 35-Amb, and the tA: 0, 30, 60, 90, 120, 150, and 180 days. The analysis of the results was carried out with the Software Statgraphics XVI.I, ANOVA with multiple range test, 95% confidence level and honest significant difference test (HDS) of Tukey.
RESULTS
Figure 1 presents the average values and standard deviation of the quality attributes of the PC+FAC according to the treatment and tA. The ANOVA showed statistically significant differences (p<0,05) in all the dependent variables, with respect to the tA, treatment and interaction (treatment - tA); except for the R-Ca (%) that did not present statistically significant differences with respect to the treatment and the interaction and the IP with respect to the interaction (p>0,05) (ver table 1).
The variables Xw and aw showed a variation between (1,7-3,4%) and (0,170-0,358) respectively, results that guarantee a microbial stability and a possible control of deterioration reactions: auto-oxidation, lipid oxidation, enzymatic reactions, and hydrolytic and browning reactions [26,11]. An increase of Xw and aw was observed during the first 60 days, then an asymptotic behavior, wh re the treatments (35ºC-Amb) and (35ºC-N2) presented the lowest Xw (2.8-2,9%) and aw (0,295-0,304) at 180 days, due to the increase in vapor pressure at 35°C. Similar behaviors have been reported in tamarind powder [27]. The S showed an increase during the first 30 days (58,4(1,6 → 68,5±2,7%) and then a decrease until day 180. The treatment 35ºC-Amb was the most critical (68,5±2,7% → 37,2±2,2%) and the most favorable were 15ºC-N2 (58,4(1,6% → 51,5(2,3%) and 35ºC-N2 (58,4(1,6% → 50,4(1,1%).
Table 1 Significance level (p-value) of storage conditions and their interactions on powder properties.
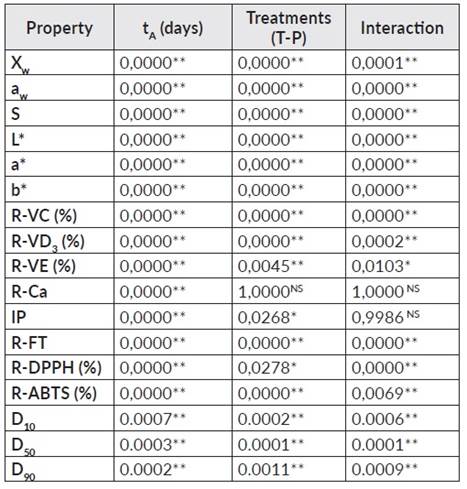
** Highly Significant Differences. * Significant differences. NS No Significance.
This situation is attributed to the high fat content (0,31 g/g solid dry) of the PC+FAC that gives it hydrophobic characteristics [28]; and to the observed agglomeration that is enhanced with the increase of Xw, which decreases the surface area and the water diffusion in the particles.
Similar behaviors have been reported in babassu milk powder [29]; tomato powder [6], and milk protein powder [30], where the formation of a reticulated protein network on the surface acts as a barrier to the diffusion of water and hydration of the particles.
The PC+FAC color was not critical because the variation of its parameters was slight, L*: 76,8-80,5; a*: 1,4-2,7 and b*: 9,0-11,7; which indicates little influence of the evaluated factors and an effect protector of the MD and GXantan contained in the matrix. These changes less than 5, do not get to be perceptible to the human eye [31]; so they will be considered as: L*: 78,6(1,1; a*: 1,8(0,3 y b*: 9,7(0,7.
During storage there were significant losses in vitamins and antioxidants in all treatments; the greatest loss occurred in the samples stored at high temperatures and packed with atmosphere (Amb). Initial amounts of vitamins and antioxidants were: Vit.C: 2,1(0,1 mg/g; Vit.D3: 223,8(12,5 mg/g, Vit.E: 0,20(0.0 UI/g; TPC: 29,7(7,6 mg galic acid/g; DPPH: 0,5(0,0 mg trolox/g y ABTS: 0,5(0,0 mg trolox/g.
The Vit.C and Vit.E showed a similar behavior. The highest R-VC was found in the treatments 15ºC-N2 (62,6(5,7%), 25ºC-N2 (68,7(5,1%), and 35ºC-N2 (44,6(2,3%); while the lowest R-VC was found at 35ºC-Amb (11,9(0,2%).
For Vit.E the most favorable treatments were 15ºC-N2 (57,2(3,2%), 25ºC-N2 (54,6(1,2%), and 15ºC-Amb (51,8(0,6%); whereas the most critical were 25ºC-Amb (46,5(7,8%), and (35ºC-Amb) (46,2(0,3%).
The degradation of Vit.C and Vit.E is attributed to the greater reactivity of the chemical structure with the O2 present in the samples with Amb packaging, widely studied [8,32,27], which is enhanced by the increase in temperature [33,32,11]; in turn an oxidative pathway catalyzed by O2 and the Fe+3 and Cu+2 ions present, could accelerate the speed of the reaction [8,32].
Similar behaviors in Vit.C have been reported in mango powders [33], milk for infants [8], and tomato [6], reaching retention levels up to 81,0% (0°C), 63,1% (25°C), and 4,7% (37°C), respectively. In dehydrated pineapple products [34], the progressive degradation of Vit.C during storage was related to the higher content of Xw (>aw), acting as a solvent and catalyst. Moraga et al. (2012) [35], evaluated the effect of relative humidity and storage time on the bioactive compounds and the functional properties of the grapefruit powder, the glassy state of the amorphous matrix must be guaranteed, which will prevent an increase in the rate of deterioration reactions related to the loss of FAC of the fruit, such as vitamin C.
Regarding the Vit.E Chávez-Servín et al. (2008) [8], reported significant losses in milk powder for infants, favored with the increase in temperature and tA. The same behavior was reported for egg powder enriched with natural antioxidants [36], in 4 powdered formulas of supplementation and / or nutritional support [32,37], where the results showed that the increase in aw and tA have a marked effect on the stability of thiamine and vitamins E and A. The Vit.D3 showed an accelerated degradation during the first 30 days and later it was slow and progressive until 180 days. n all cases, the degradation was greater in the samples packaged in Amb than in N2, which is related to the reactivity of Vit.D3 with O2 [8,32]. The highest losses of Vit.D3 occurred in the treatments 35ºC-Amb and 35ºC-N2, with a retention of 32,9(0,3% and 35,3(0,9% respectively; while the smallest losses were at 15ºC-Amb (48,7(1,4%) and 15ºC-N2 (51,0(1,0%).
Some investigations describe the important effect of pH, light, and heat [36,32;38;37] on Vit.D3 in foods, however the studies of stability in powders are limited, highlighting mainly avocado powder added with Ca, Fe and vitamins A and D3 [38], where the greatest losses occurred with the increase of time and temperature; In addition, the packaging effect (N2 and vacuum) were similar. Mahmoodania et al. 2018 [32] reported that vitamin D in milk powder appears to be quite stable for atmospheric oxygen, but could be susceptible to reactive oxygen species. Therefore, the lipoxidation of fatty acids, which produces reactive oxygen species, could be one of the ways to cause the degradation of vitamin D in fortified products that contain fats, as is the case of PC+FAC. The R-Ca, in general was stable during the tA (aprox. 98,9% at 180 days). It was found that the highest R-DPPH, R-ABTS, and R-TPC at 180 days was presented in the treatment 15ºC-N2, with values of 91,9±1,8%, 42,1±2,2%, and 50,9±4,8% respectively, showing the lowest R-ABTS in the last 30 days; while, the R-TPC and R-DPPH had a linear behavior throughout the tA. The lowest R-TPC was observed at 35ºC-Amb and 35ºC-N2 (37,5±5,5 and 36,7±2,2 respectively); while for DPPH it was at 35ºC-Amb (86,3±2,1%). For the ABTS the values were practically similar for all the treatments in each tA, being the R-ABTS at 180 days of 41,5±1,1%.
A similar behavior was reported in reported for apple peel powder [5], using high density polyethylene bags and high barrier metallized films, where the TPC decreased with the tA and the temperature in both materials. The results indicated that a higher Xw accelerated the degradation of phenolic compounds; On the other hand, it has also been found that the availability of O2 as a result of the permeability of packaging materials is determinant in the loss of phenols [39]. Other investigations suggest that the loss of phenolic compounds and antioxidant capacity are associated with TA, pH, exposure to O2, light, and changes in Xw and aw, which generate agglomeration due to the rapid absorption of water in the first days of tA and finally, to the formation and accumulation of melanoidiones, as well as the reaction products of Maillard [40]. The latter coincides with that reported for cranberry powder [7^, stored in the dark at 4°C (losses of 10% in TPC and 15% as ABTS after 4 weeks); while, at 25°C and in the presence of light, the losses were 33% in both. For all treatments, the IP increased from 2,43(0,89 to 3,5(0,17 meqH2O2/kg fat at the beginning of storage (0 ( 60 days); subsequently, sharply decreased to average values of 1,0±0,2 meqH2O/kg fat (day 90) and from there, showed a tendency to decrease slightly until day 180, obtaining average values between 0,12±0,03 and 0,89±0,3. An IP value of 10 mEq. O2/kg fat was considered as the critical limit for acceptance of PC+FAC, beyond this value the product could perceive rancidity [41^.
This behavior of the IP is attributed at the beginning to the formation of hydroperoxides and to the increase of the surface oil content [42,26,43] and subsequently, to the conversion of free fatty acids into secondary oxidation products (alkanes, alkenes, malonaldehydes) [43].
The PC+FAC is vulnerable to lipid oxidation leading to the formation of fat peroxides caused by molecular O2 and fatty acids, such behavior may be due to the oil being released to the surface of the powder during storage, due to physical and chemical changes in the wall materials and the molecular diffusion of the oil through them [44]. At the same time the encapsulation structure could also be weakened when the samples are exposed to higher temperatures during longer tA, in the presence of air, light and the O2 permeability of the packaging used, which could cause a higher oil release rate on the surface. Other phenomena can also occur: the presence of antioxidants such as tocopherols and TBHQ, which reduce the production of peroxides and free fatty acids; which could explain the low IP values during PC+FAC storage. The particle sizes showed a similar behavior, increasing in the 3 percentiles during the storage and being the treatment 15°C-Amb which showed the highest increase: D10 (1,7(0,03 ( 13,3(3,0 µm), D50 (8,5(2,3 ( 870,5(55,2 µm) y D90 (78,8(27,0 ( 1350,1(1,8 µm); while, in the other treatments, the differences were not significant at 180 days (D10: 2,03(0,3 µm; D50: 33,2(14,9 µm; D90: 168,5(81,8 µm). This behavior is related to the increase of Xw, which favors the agglomeration of PC+FAC particles, losing their free-flowing characteristics and forming lumps.
Figure 2 shows the microstructure obtained by SEM of PC+FAC particles at 15°C and times of 0 and 180 days. Agglomeration on the surface of the powder during storage was evident [6,30]. The particles initially exhibit a spherical shape and variability in their sizes, smooth and rough surfaces, some with collapsed walls and agglomerated structures, due mainly to Van der Waals (attractive) and electrostatic (repulsive) forces [45,30] which is characteristic of powders obtained by SD [41,25,18,30].
At 180 days, the PC+FAC exhibits a structural collapse due mainly to the high agglomeration, probably caused by the absorption of water on the surface of the particles, which could be the early stage of the caking phenomenon described above. [11]. On the other hand, the increase of the Xw favors the humidification of the sugars that are crystallized forming agglomerations by the effect of the stickiness; the same behavior has been reported in borojo powder encapsulated with MD [26,30]. The collapse of the pulverized products implies time-dependent structural changes, which are accompanied by changes in the mechanical properties that are related to caking or stickiness; on the other hand the speed of caking and/or agglomeration is a function of several variables: temperature, RH, time, and position within the powder, where particles exceeding the moisture threshold value stick to each other; presenting four different states: bridge formation, agglomeration, compaction and liquefied [35;26,30].
CONCLUSIONS
The PC+FAC obtained by SD is a food matrix whose encapsulants effectively protect the FAC present during storage. In general, the quality attributes of the PC+CFA were affected by all the independent variables, the storage condition that most favored PC+FAC was 15°C-N2, where the gain of Xw and the increase of aw were low; losing its free flow characteristics and presenting formation of lumps where the PC+CFA is a stable product, nutritionally rich in fat, fiber and CFA and hygroscopic, where the absorption of water confers a plasticizing effect on its structure, additionally, there was a greater retention of vitamins and antioxidants.