1. Introduction
Measurements of the human body are essential to aid diagnostic processes in various areas of health science, especially those related to movement. In many cases, these measurements are conducted by highly trained professionals through traditional techniques, but gradually, a large part of them have migrated to technological systems.
Gait analysis supported by technological elements is usually carried out in specialized laboratories that contain a variety of advanced technological devices that are accessible and affordable for a small part of the population. Due to this reality, the capture and analysis of kinematic gait information is a limited and expensive process, coupled with the fact that these laboratories are not available locally in most situations,
Currently, the systems used to capture mechanical and kinematic movements in the human body are used in several diagnostic processes [1-3]. They are based on various types of technology [4-6], offering a broad set of characteristics. Within these types of technology, the magnetic-inertial based systems and optical systems stand out, widely used in different environments and case studies.
In order to identify and recognize the different technologies used for gait characterization, a chronological review of these systems is carried out from 2015 to 2018, a period in which the most important advances for the interest area have been documented.
In the same way, this work uses a commercial optical system (Microsoft Kinect) together with an inertial-magnetic measurement platform (Imocap) that will be applied in the gait analysis to assist in the diagnostic processes and creating rehabilitation plans. This last inertial-magnetic capture system (Imocap) was proposed, designed and developed by the GIS Software Research Group of the Pedagogical and Technological University of Colombia [7].
First, this article presents some basic concepts on the subject related to the research, describing the previous work on motion capture processes for the lower limbs of the human body and motion capture specifically in the gait cycle.
Afterward, a gait evaluation proposal by means of inertial-magnetic sensors and optical-based systems is presented, to finally achieve the discussion and conclusion derived from this investigation.
2. Literature Review
The aim is to present a review of the literature on current systems for collecting biomechanical information, based on different types of technologies such as inertial or magnetic sensors and optical recognition systems. This section is divided into subtopics that group similar works, facilitating the recognition of the main authors and their contributions.
2.1. Method
Some articles were selected that reported on the incorporation of technology into the motion capture for rehabilitation purposes (motion capture systems, rehabilitation motion capture systems) between the years 2017 to early 2020. Subsequently, publications that reported on the use and/or combination of sensors and other technologies, such as video cameras, infrared cameras and other types of optical and inertial-magnetic motion capture systems, were considered.
Then, some papers that reported motion capture processes with rehabilitation purposes focused on the lower limbs of the human body were selected. Finally, the works which reported on motion capture processes for rehabilitation, specifically in the gait cycle, were preferred.
Publication search was done in IEEE Xplore Digital Library (239 publications), Scopus (232), ScienceDirect (121), EMBASE (108), PubMed (86) and EBSCO (76). This search was related to the term "Mocap", "movement/motion capture", "lower limbs" and "rehabilitation". The scope of the search was limited to 2017-2020. The information filtering process was based on a search with the same terms in the title, abstract or keywords.
From the collected documents, 192 papers were obtained. Subsequently, duplicated documents were eliminated, reducing the sample to 83 documents with full-text availability. Then, using the Mendeley reference management tool, the documents were classified according to the technological tools used in the rehabilitation process, and a complete analysis of the literature was performed, grouped by the type of technology used. In the course of this section, the most relevant contributions of the selected documents are reflected to form this scientific literature review.
2.2. Motion Capture Systems
Technologies associated with medical diagnostics usually use motion capture systems. When the gait analysis is performed, the acquisition of biomechanical data from the lower limbs of the human body is imperative. Biomechanical information capture systems can be of three different types: optical, inertial, or both. The main technologies used to capture movements are shown in Figure 1, as described by Callejas [8].
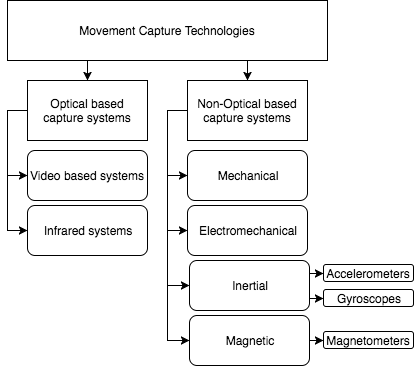
Figure 1 Motion capture technologies classification, adapted from the original figure by Callejas, etal [ 8].
The availability of different technological tools for diagnostic assistance allows an objective evaluation of patients, and therefore, this process will not be limited to the experience of the medical team or the technical evaluation team.
2.3. Motion Capture Based on Optical Systems
One of the most relevant works found in the area of information gathering on biomechanical movements belongs to Dawe's team [9]. The accuracy and feasibility of using an optical system based on depth detection is tested, quantifying the movement of the hip and knee joints of older adults. The results showed that the accuracy of the depth camera varies according to the analyzed gait parameter. For example, for the knee and hip joints, the system can follow a path tendency of the joints. In this work, no comparison was made between various optical devices with a reference system of another technology.
As a complement, a specific work highlights the validity of a Kinect-based system for the clinical evaluation of the knee kinematics [10]. In this case, the Kinect device was compared to another optical motion capture system. The results showed that it is possible to use a commercial device like Kinect for the study of this type of movement applied in robotics and biomechanics applications. In conclusion, the results shown are satisfactory, and a commercial system could be used for kinematic evaluations at a fraction of the conventional marker-based optical motion capture system.
Kim and Bae's work [11], proposes the use of advanced techniques to capture the joint movements of multiple users using only Microsoft Kinect. The results show that it is possible to capture motion using this low-cost system, but with some limitations, such as obstruction and localization problems of human body rotations.
Specifically for gait analysis, Tuan [12] found promising results using optical depth systems (Microsoft Kinect 2) and data fusion processing with hidden Markov models. More advanced models for motion pattern recognition use activity-recognition sensors fused with simple optical systems, such as the work shown in [13].
2.4. Motion Capture Based on Inertial-Magnetic Systems
The advent of inertial-magnetic systems has accelerated the development of several low-cost devices, revolutionizing the way motion capture is used. These systems are now available for multiple applications including gait analysis and others in the field of health and medicine [14-16]. In these works, it is emphasized that universities and institutions are committed to investing in inertial-magnetic technology over optical recognition systems since their multiple advantages have been demonstrated in various applications.
Likewise, the problems associated with inertial-magnetic systems have been solved more easily using mathematical tools and digital signal processing than in optical systems, laying the groundwork for the use of this technology in motion capture systems.
A comprehensive analysis of motion capture systems based on inertial units, including six specific diagnostic areas, is presented in [17]. The results show that the gait analysis carried out with this system allows to evaluate adequately the stability of the upright gait and its spatial-temporal variables.
Conversely, the main problems are concentrated in the numerical drift that affects the integration in the estimation of parameters that are not measured directly, the deviations due to gravity acceleration, and the need for the correct alignment between the sensors and the anatomical axes. The results of this research are confirmed by academics from the University of Beijing [18], where the use of special data fusion is proposed to correct the drift of the inertial sensors.
There is also a study that relates the pathologies that directly affect the gait cycle and its evaluation with different motion capture system architectures based on electromyographic signals [19], where three characteristics of each muscle involved in gait were extracted. This method allows them to summarize, quantify and simplify the evaluation protocol.
In the study of the University of Electronic Science and Technology of China [20] different movement frequency components were evaluated to detect degenerative neuropathies exposed in the gait. The results showed that other types of diseases could be characterized by measuring the gait cycle with inertial motion capture systems.
Park's team at the University of Ulsan [21] managed to control a robotic exoskeleton to assist in the recovery process for the proper gait cycle. In this study, an inertial motion capture system collected the motion data from five patients to create rehabilitation plans, validating the motion capture system for this rehabilitation application. On the other hand, the study of Wang and his team [22] compiles several works that focus their development on active monitoring systems for daily activities. It highlights a wide selection of Sensor-based human activity recognition (HAR) devices for the field of rehabilitation.
Many complementary works use inertial-magnetic technology for the detection of the movement parameters during the running cycle [23-27]. It stands out as the main quality, the speed of information collection, the efficiency in the application of various digital processing algorithms and the high precision of the sensors.
2.5. Motion Capture Based on Inertial-Magnetic and Optical Systems
Researchers from the University of British Columbia in Canada [28], developed a single shoe-embedded sensor module that can measure the foot progression angle (FPA) during walking and providing all-inclusive information for the analysis of the foot movement. As highlighted in the conclusions of the study, the shoe-embedded sensor module is a valid and reliable method of measuring FPA during over-ground walking without the need for laboratory equipment.
In addition, a comparison of motion capture technologies was performed in [29], where the characteristics of radar-based motion capture systems were compared with optical-depth motion capture systems (augmented optics) and some wearable devices. They show that the use of enhanced optical systems is favorable, excluding radar-based systems due to the multiple constraints found.
Marxreiter and other researchers [30] proposed the use of their inertial sensors for the gait parameters measurement in patients with Parkinson's disease. The team has successfully demonstrated that gait analysis based on inertial sensors achieves a clinical reliability degree, providing high-resolution biomechanical information for the gait cycle recording in patients with the pathology mentioned above, and without the need to have a specialized gait analysis laboratory.
Watanabe et al [31] conducted a study in which their prototype based on inertial sensors was used for gait analysis, focusing on stride length and joint angle measurement. As in the review made by Petraglia et al [32], the results showed that the angles of the lower limb joint are accurately estimated using inertial sensor-based system, exhibiting a high correlation between the joint angles in some characteristic points and the striding speed. These results suggested that systems based on inertial sensors can detect sufficient characteristics for an adequate analysis of the gait.
Additionally, in a later work carried out by Hanawa and his work team [33], it was demonstrated that inertial sensors are efficient to find movement estimations and reaction forces in lower limbs. It should be noted that the method based on inertial sensors is suggested as an option to estimate the junction moments of the trunk segments.
An interesting job is the one developed by Fleron et al [34], where the objective was to determine the accuracy of trunk speed while walking, using an inertial motion system compared to a gold standard optical motion system. It was concluded that trunk speed extracted from inertial systems has a reasonable precision during the gait movement, but the accuracy was reduced during the transition periods.
Finally, the work of Tjhai is presented [35], since it makes a comparison between an optical motion capture system versus low-cost inertial sensors in the gait movement on a treadmill. The results were satisfactory for the inertial sensors, where the result shows that the average errors of pitch angles and step lengths are 3 degrees and 6 cm, respectively.
2.6. Results of the Review
Research on human movement and gait analysis is expected to use sensor fusion technologies to obtain more accurate estimates. Digitally captured data is intended to be as close as possible to actual movements with the inclusion of complementary technologies. Furthermore, the use of portable and reliable platforms is required in different environments and locations, decentralizing the use of these systems to have a more direct reach to the population.
It is emphasized that the conduction of technological diagnostic techniques using motion capture devices based on inertial systems can reduce costs and evaluation times compared to optical technologies. The use of inertial technologies can be widely available as a diagnostic alternative that assists or replaces traditional evaluation processes that do not have the support of biomedical devices.
Finally, as can be seen in the literature review, there is no specific point of comparison between motion capture technology based on inertial sensors and motion capture with optical systems. For this reason, it is considered conducive to the development of this research, considering the movement of the gait in the lower limbs of the human body.
3. Gait Measurement and Evaluation Proposal
The following section describes the architecture of a biomechanical inertial-magnetic motion capture platform and an optical based system, coupled with a methodological proposal that allows to evaluate the gait cycle.
3.1. Motion Capture Architecture Proposal
The motion capture system used in this research is based on two components: an inertial-based motion capture system and an optical-based motion capture system. The first system, called Imocap [7], was developed in the GIS Software Research Group (by its acronym in Spanish). Imocap uses a variety of sensors that operate with inertial technology (accelerometers and gyroscopes) together with magnetic technology (magnetometer) which, using the appropriate data processing technique, provide three-dimensional motion information performed by a patient.
In addition, the Microsoft Kinect device is also used, which is complementary to the previously described systems. This device is included since it has a set of cameras and depth sensors that allow motion detection. With adequate data fusion, the use of these two systems collects biomechanical data in simultaneous processes and provides the necessary information for a complete gait analysis measuring architecture is shown in Figure 2.
3.2. Methodological Proposal
The gait evaluation is carried out systematically following the proposed stages, which are based on the works of various researchers [36-39]. The use of the optical system (Microsoft Kinect) stands out in stage 3 measurements since this system has a better performance in the measurement for the spatial parameters related to distances. The Imocap motion capture system (inertial-magnetic technology) will be used to capture the biomechanical movement and to extract the kinetic variables and articular amplitudes related to the lower limbs, as proposed in stage 5. The following is a detailed description of the proposed stages.
Stage 1: Identification of motor dysfunctions shown by the study subjects. It is important to make a prior medical assessment to know the patient's overall health status, and to identify the origins of their possible motor dysfunction.
Stage 1 activities:
To typify patients with mild motor injuries in lower limbs, on which the evaluation will focus.
To define the motor disorders and dysfunctions of the patient, as well as the possible pathological neuromotor origins.
To register the results obtained in the patient's medical record.
Stage 2: Determination of anthropometric variables and characteristics presented by study subjects.
Stage 2 activities:
To record the patient's age, overall height, left leg length, right leg length, left foot sole length, right foot sole length, left leg length up to the knee, right leg length up to the knee, and body weight.
Stage 3: Determination of preliminary spatial variables in the gait cycle, using the Microsoft Kinect optical system as a motion capture system. Preliminary variables of gait movement describe general cyclical behaviors, which must be recorded for a thorough evaluation
Stage 3 activities:
To measure the overall length of the gait cycle, in centimeters.
To measure the step length, on both the left and right side, in centimeters.
To observe the ankle movement, specifically the plantar/dorsal flexion and foot support in the ground. Record if heel shock occurs.
Stage 4: Anthropometric location of the inertial-magnetic motion capture system. The biomechanical information capture system is accurate if it is used correctly, and its sensors are located in the correct position.
Stage 4 activities:
To select the side of the human body where the measurements will be made.
To place the first sensor on the dorsal side of the foot, at the height of the metatarsal bones, seeking sensor stability. The measuring Z-axis must be aligned with a perpendicular plane to the leg center axis or the gait progression axis. The X-axis will measure the foot displacement movement in the support and takeoff phases. The Y-axis will measure the movement of the foot sideways.
To place the second sensor in the leg's tibial tuberosity, just below where the anterior surfaces of the lateral and medial tibial condyles end, with the Z-axis aligned in parallel with the sagittal plane. The X-axis will measure the angle of the leg in conjunction with Sensor 3. The Y-axis will measure the lateral displacement when the patient rests the foot on the ground.
To place the third sensor on the leg above the knee, resting on the front of the thigh. The X-axis will measure the angle of the leg in conjunction with Sensor 2.
To place the fourth sensor on the lower back, resting on the lumbar area, aligned with the spine. The X-axis will measure the flexion-extension movement of the back. The Y-axis will measure the lateral displacement of the hip.
Stage 5: Determination of spatial-temporal variables of the gait cycle with the Imocap (inertial-magnetic) motion capture system. The characteristic motion variables describe the complete behavior of the gait cycle.
Stage 5 activities:
To record the gait activity for a specific time (not less than 2 minutes, not longer than 12 minutes).
To extract the characteristic variables from the sensors.
To find the spatial-temporal values: gait balancing speed, average gait speed and cadence.
Stage 6: Gait motion analysis. Gait motion analysis allows you to collect the information needed to generate evaluation reports and results.
Stage 6 activities:
4. Discussion
One of the main advantages of using motion capture systems based on inertial-magnetic technology is that the costs associated with medical diagnosis are reduced since these systems are much more affordable than those based on optical technology. In addition, measurement systems with inertial-magnetic technology are portable, require less operational infrastructure, and therefore, satisfy the demand of more population.
Some of the disadvantages of inertial-magnetic systems described by Iosa [37], are solved by the implementation of additional processing techniques such as Kalman filters. Other solutions can rely on effective data fusion, including Attitude and Heading Reference Systems (AHRS), that partially solve the different numerical derivations presented. Also, the use of the methodological measurement and evaluation proposals presented in this work can provide troubleshooting for sensor locations in the human body.
5. Conclusion
In the global context, the use of technologies for gait analysis is generally carried out by means of optical systems without the use of inertial-magnetic sensors. The proposal presented is considered to be a pioneer in the fusion of sensors to provide diagnostic assistance of gait movement. A contribution is made in the evaluation of human movement in a decentralized way, since the motion capture systems with inertial-magnetic sensors do not require a previous technological infrastructure, their installation is simple and they are economically affordable devices.