Introduction
While the area of decomposition volatile organic compounds (VOCs) is becoming a more developed area in forensic science (Iqbal et al. 2017), the subcategory of residual odor VOCs is still an under researched field of study. Residual decomposition odor is the result of the alteration of the environment after contact with decomposing human remains and the subsequent deposition of chemical residue by a body in this putrefactive state (Alexander et al.2015). By studying VOC odor profile changes after a cadaver has been moved from a particular environment, better understanding of how long a cadaver was at a particular location, or even how long it has been since it was removed from a given location can be achieved. Residual decomposition odor detection has many uses in the law enforcement and forensic field; this includes locating previous holding sites or transportation methods, which can aid in criminal cases, as well as potential training sources for human remains detection dogs. (Prada-Tiedemann, Rojas-Guevara, Bohórquez, Ochoa-Torres & Córdoba-Parra, 2019). Based on the response of Human Remains Detection (HRD) dogs when using training aids, it is established that human remains leave behind residual odor on items they come in contact with. A seminal study was conducted in 2008 as a proof of concept to showcase how cadaver dogs alerted to corpse-contaminated carpet squares up to 65 days post-exposure (Oesterhelweg et el. 2008). In terms of decomposition studies, soil acts as a good sink for decomposition VOCs due to burial and concealment attempts (Forbes et el. 2014). Thus, a number of studies have utilized soil to study the persistence and detection of decomposition-related VOCs from a range of different angles, including burial depths, seasonal variations, and variable instrumental techniques (Iqbal et el. 2017). As better understanding of residual decomposition odor develops, it can have a wide range of benefits affecting not only the forensic science community but also in the way that law enforcement handles criminal case evidence and the training techniques employed by HRD dog trainers. Research of this nature can also have a positive benefit on developing instrumentation and best practices that can locate clandestine grave sites (Blau et el. 2018). Not only would HRD dogs and their handlers benefit from a better understanding of decomposition VOCs but it would allow for better development of cadaveric material detection devices as well as the ability to determine or narrow-down a post-mortem interval (PMI) based on a VOC profile [Dekeirsschieter,2009].
Decomposition can be broken into five stages: fresh, bloat, decay, post-decay and dry stage, which occurs over the course of about a month. During this process, the large macromolecules of the body break down into organic and inorganic matter, which release a very pungent odor [Statheropoulous et el, 2011]. It is this pungent odor that HRD dogs are trained to detect, which is composed of many volatile organic compounds (VOCs) and inorganic gases. The seminal work of Vass identified 30 key compounds as markers of human decomposition which were detectable at the soil surface (Vass et el. 2008). However, it cannot be guaranteed that one will find specific chemicals present during a specific timeframe of decomposition due to the effect that numerous taphonomic factors have on decomposition events (Ioan et el 2017). Most research agrees on the classes of compounds that are found in decomposition VOCs, with the most reported being polysulfides, such as dimethyl sulphide (DMS), dimethyl disulphide (DMDS), and dimethyl trisulfide (DMTS) (Vass et al 2004).
Human remains detection (HRD) dogs are trained to alert to the odors released when a body decomposes, this allows HRD dogs the ability to also detect areas were remains have been previously decomposing, but are no longer there [Forbes 2016]. The way in which HRD dogs locate and distinguish human remains is an area that is not fully understood, especially the specific chemical odor signature the dogs employ when making their detection alert and how the ratios of these chemical odors affect performance over time [Vass 2004]. Residual odor can be defined as "odor that originated from a target substance that may or may not be physically recoverable or detectable by other means" (ASB Technical Report 025). In an effort to experimentally evaluate residual odor effects on canine performance, a study examined the ability of eight nationally certified HRD canine teams to detect human remains odor in soil from under decomposing human remains (Alexander et al. 2015).The result highlighted that HRD canines were able to detect the odor of human remains from the contaminated soil surface with a post body removal of 667 days with 85.7% accuracy [Alexander, 2015]. An HDR dog is typically trained using the entire decomposition spectrum as its target odor source, hence they are trained with body parts, soft tissue, decomposition fluid (adipocere), blood, teeth, and even bone [Nizio, 2017; Prada-Tiedemann, Ochoa-Torres, Rojas-Guevara & Bohorquez, 2020]. Currently these dogs are trained on a combination of human remains, individual tissues, and/or synthetic training aids.
The most widely recognized human analogue is the pig (Sus scrofa), due to its internal anatomy, fat distribution, size of chest cavity, lack of heavy fur, as well as their omnivorous diet which may lead to similar gut fauna as humans [Schoenly, 2006]. It is commonly noted that the most distinct difference between human and animal decomposition VOCs is that human remains tend to produce more esters than animals [Rosier, 2016]. The similarities between pigs and humans that are most commonly cited are diet, endogenous micro biome, the ratio of fat to muscle, body hair distribution, structure and density, and a monogastric digestive system [Anderson, 2011., Archer, 2004., Cockle, 2015., Connor, 2018., Cross, 2010., Horenstein, 2010., Pakosh, 2009., Reeves, 2009., Stadler, 2013., Turner, 1999 & Wilson 2007].
Even though research has geared toward the analysis of decomposition volatiles from various perspectives (Iqbal et al 2017), residual odor volatiles from soil samples have been even more limited which highlights the infancy of the field. The first decomposition study evaluating volatiles released into soil surfaces was conducted using buried pig carcasses (Brasseur et el 2012). This study reported 20 functional VOCs specific to the soil samples collected below the carcasses and 34 methyl-branched alkanes found throughout the grave depth profile. More recent studies have undertaken the analysis of soil samples collected from under pig carcasses utilizing various instrumental techniques to provide an enhanced analytical perspective on the characterization of volatiles in decomposition soil odor profiling (Perrault 2014, 2015a, 2015b). The purpose of this study is to continue longitudinal analysis perspectives of decomposition VOCs in soils contaminated with decomposing porcine remains throughout various decomposition phases in a West Texas outdoor environment. Knowing how long residual decomposition odor remains viable in soil surfaces will aid in training techniques (Rojas-Guevara, Prada-Tiedemann, Titus, Córdoba-Parra & Bohórquez, 2020 in press) for Human Remains Detection (HRD) dogs and help formulate a better understanding of volatile odor uses for victim recovery. This information can shed light as to how long decomposition odor contaminates a soil surface and thus may affect HRD canine operational performance. Currently, when a certified HRD canine gives a trained response in a location where no visible remains are present, the handler might inaccurately determine the response as a false alert or mistake when, in reality, it could be the presence of residual odor from a decomposing body being at the location previously. This study could also aid in the development of possible portable analytical field detection instruments that would allow for the reliable, cost-effective detection of clandestine burial sites.
Both a pig-meat simulation model as well as pig carcasses were used in place of human cadavers, allowed to decompose for three distinctive time periods, 14, 17 and 21 days, and then removed from the deposition site. After removal, the headspace of soil samples, taken from under the cadaver decomposition island (CDI), were analyzed once per week for a period of 4 weeks using solid phase micro extraction- gas chromatography/ mass spectrometry (SPME-GC/MS) as the instrumental analysis method. While there have been limited studies in terms of volatile residual odor in soil matrices, it is the goal of this work to expand the analytical knowledge of residual decomposition odor volatiles in the West Texas region characterized by arid and colder climate conditions. Characterization of VOCs of interest was conducted highlighting trends in abundance and presence as a function of remains contact with soil surface.
Materials & Methods
Field Materials and Study Location/Climate
All pork meat used in the study was Smithfield Pork Shoulder Picnic Roast which was purchased from Walmart. This pork meat contained no added ingredients or preservatives. The pigs used in this study were wild pigs (Sus scrofa), which were donated from a ranch in Centerville, Texas (Smith Ranch, 13715 Co Rd 122, Centerville, TX 75833). The pigs were already deceased when obtained, and hence not killed for this study. These particular Sus scrofa were pigs that were previously hunted, but were too small for hunters to keep. Since the pigs were donated postmortem and never handled while alive, Texas Tech University Institutional Animal Care and Use Committee (IACUC) did not require animal ethics approval and only needed a memo stating the purpose of the research and how the pigs were obtained. A total of 3 pigs were employed during this study. The pigs were frozen within 3 hours of death and remained frozen until ready to be placed for this study. Before study placement, the pigs were allowed to thaw after refrigeration and were placed on test site once completely thawed.
The study site was land located at 850 N State Road 1490, Levelland, Texas 79336, which can be seen in Figure 1. Permission to use this land was obtained by the land owner, Shane Grissom, prior to the start of the study. All pork meat models and pigs were placed with ample space between each different set of decomposition time periods. A control area was designated, where no pig or meat model was placed at any time, in which blank soil samples were collected from. This control area was enclosed by the same type of cage material that enclosed both the pork meat models and pig samples. This control area provided a baseline of VOCs that were present in the soil matrix alone, which helped in determining which VOCs should have been determined to be a product of decomposition. The control area, as well as the placement for the pork meat model samples and Sus scrofa trials can be seen in Figure I. Furthermore, the figure depicts the arid terrain from where the samples were collected as well a map highlighting the geographical difference from other common study sites of decomposition studies. The cage materials were purchased from a local hardware store (The Home Depot) and consisted of EVERBILT V> inch wire cloth, rebar and zip ties (The Home Depot, Atlanta, GA). The V inch wire cloth was cut into 3ft sections and formed into a circular cage, secured with zip ties. Each cage was secured to the ground with the soil exposed and then a rock barrier (found in nearby environment) was built around the outside of each cage to deter scavengers from attempting to dig under the cages.
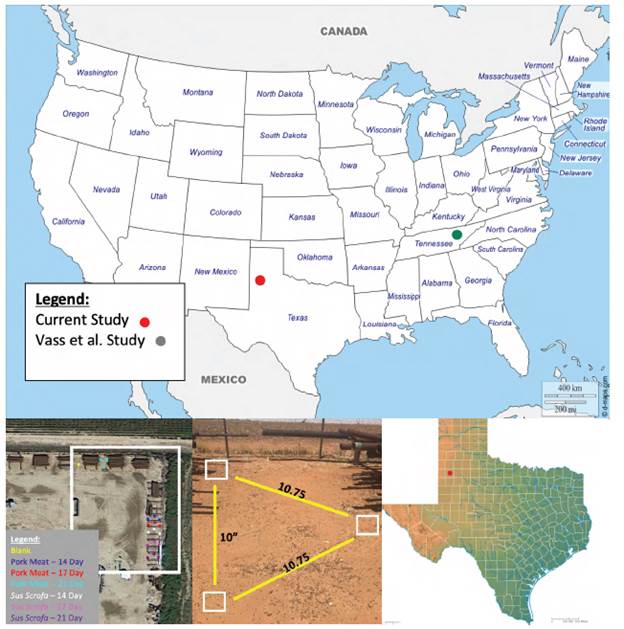
Source: Own elaboration.
Figure 1 Map of study location and placement of the cages for the duration of the study.
Part of the novelty of this study is the climate in which it was conducted. It has been discovered in previous studies that temperature can influence the decomposition odor profile. According to the Koppen-Geiger climate classification, Levelland, Texas is considered to be a BSk, the B is for climate which is considered arid, the S is for precipitation which is classified as steppe and the k is for temperature which is considered to be cold arid. The average rainfall per month rages between 0.67 to 2.82 inches [https://www.usclimatedata.com/climate/levelland/Texas/united-states/ustx0760]. It has been determined that sandy soils, such as those in dry, arid climates, tend to have a lower soil porosity with a larger pore size, this allows better draining which in turn allows more pore space to air than water [Alexander 2016]. Due to having more air than water in the soil pore space, decomposition gases are better held in sandy soils versus clay soils [Alexander 2016]. It has been proven that VOC profiles released by a decomposing corpse can be affected by environmental biotic and abiotic factors.
Laboratory Materials
All standard laboratory procedures and protocols were followed during the duration of this study. Optimization procedures for the SPME methodology included initial conditioning of all SPME fibers (Supelco, Inc., Bellefonte, PA). SPME fibers used throughout this study were StableFlex Divinylbenzene/Carboxen/ PDMS (DVB/CAR/PDMS) (Supelco, Inc., Bellefonte, PA). SPME fibers were baked at 250°C for 30-minute increments until the fiber showed a clean run in the GC-MS. All samples were collected in either 10mL or 15mL clear glass vials with screw tops containing PTFE/ Silicone septa (Supelco, Inc., Bellefonte, PA). The glass sample vials were sterilized with acetone and baked for one hour at I05°C. Each fiber was cleaned between samples and sample vials were discarded after each use. The temperature and humidity at collection time, equilibrium time, and exposure time were recorded for each sample collection. Temperature and humidity were collected using a digital thermometer and hygrometer (Fisher Scientific). The soil moisture content and pH were also recorded at time of sample collection using a Gain Express ZD-06 soil pH and moisture meter (Gain Express, To Kwa Wan, Hong Kong). For this study an Agilent 6890N gas chromatography (GC) was used in conjunction with a 5973-mass selective detector (MSD) to analyze VOCs found in soil samples.
Proper personal protection equipment (PPE) was worn when collecting the samples. Personal protection Tyvek coveralls (SIRCHIE, Youngsville, NC), Insta-Gard surgical cone masks (Cardinal Health, Waukegan, IL), and nitrile gloves (Kimberly Clark, Irving, TX) were worn during every sample collection to eliminate sample contamination as well as provide protection when handling decomposing matter.
GC-MS Method and Ramping Determination
Cablk, Szelagowski and Sagebiel's GC-MS method was used as the starting point for the method development used in this study [Cablk 2012]. The method was manipulated by adjusting the ramping and hold times until the optimal chromatogram was observed with individualized peaks and high resolution. The actual GC-MS method used had an overall runtime of 25.5 minutes, it started at 40°C and ended at 250°C. The ramping can be visualized in Table 1. The carrier gas used for the duration of the study was Helium (99.999& purity) at a 1.0 mL/min flow rate with a PSI of 7.1 and the instrument was operated in split-less mode. The results of each sample run were compared week to week to determine how the VOCs changed over the course of 4 weeks, as well as how they changed due to the initial decomposition period after removal. Compound identification was based on the NIST 2017 Mass Spectral Reference Library.
Decomposition Time Optimization
To determine the time frame in which viable decomposition VOCs were obtainable, pork meat models were placed and analyzed. Wire cages were built, and placed around each meat sample, the cages were secured to the ground with rebar in order to eliminate scavenging but allow for invertebrate activity, see Figure 2 for visualization.
A total of four meat models were placed and each sample was allowed to decompose for a set amount of time (120, 240, 288, and 336 hours) before being removed and sampling the soil underneath. All four decomposition periods resulted in detection of common decomposition VOCs. This timeframe was used to determine the decomposition periods selected for the actual study. This meat model study also allowed for optimization of the chosen GC-MS method, as well as the sample equilibrium time and fiber exposure time. In all four decomposition periods, ketones were the most abundant type of compound found. The data indicated that longer decomposition timeframes, before removal of the sample, more ketones were able to be detected, as well as overall compounds. It can also be seen that the number of ketones detected is proportional to the number of overall compounds detected for each of the four decomposition periods.
Pork Meat Model
A pork meat model was allowed to decompose and then analyzed for a period of 6 weeks in order to prove the sampling method worked before placing pig cadavers. Three pork meat models were placed for each chosen decomposition time period, for a total of nine individual meat models. The decomposition time periods chosen were 14, 17 and 21 days, due to increasingly cold weather conditions the length of decomposition was extended. Nine pork meat models in total were placed in nine individual cages. Each decomposition time interval was spaced at a minimum of 6 feet apart. The meat models were allowed to decompose for their allotted time frame, then the meat was removed and properly discarded. The pork was purchased immediately before placement to insure it was stored at temperatures that would combat the start of decomposition processes. The pork samples were all within 0.5 lbs. of each other in order to maintain consistent results in terms of decomposition and odor production, this also meant that the surface area in contact with the soil was similar as well. The weights of each meat model as well as their placement and removal dates can be seen in Table 2.
Table 2 Meat model weight, placement and removal dates.
Two samples of approximately 8-10 grams of soil each were taken from the areas directly underneath the meat models, for a total of six samples for each decomposition interval. The area underneath each model was determined to be the most fruitful area for collection of decomposition VOCs. The two samples were collected in a straight line, with approximately 4 cm between each sample location. Each of the meat models were weekly sampled for a total period of six weeks, as well as the control area for a total of 21 samples each week. During each sampling period weather conditions such as the temperature and humidity were recorded, as well as the soil moisture and pH for that particular sample location. Each individual sample was collected in a sterilized 15mL glass vial then wrapped in parafilm to prevent VOC seepage during the equilibrium period. Samples vials were allowed to equilibrate for 24 hours at room temperature in the laboratory and then the SPME fiber was exposed for 24 hours at 40°C. After exposure, the SPME fiber was retracted back into the SPME holder until the sample was injected into the GC-MS for analysis.
Sus scrofa Trial
Pig cadavers were then used to replicate the meat model in order to complete the study using a more acceptable human analogue (Matuszewski et al 2019). Three pig fetuses were placed at the study site for each chosen decomposition time period. The decomposition time periods chosen were 14, 17 and 21 days, to align with the pork meat model study discussed above. The three pig fetuses were each placed into individual cages to deter scavenging, but still allow for insect activity during the decomposition process. Each decomposition time interval was spaced at a minimum of 6 feet apart. Each of the pigs were allowed to decompose for their allotted time frame, then the pig was removed and properly discarded. The pigs were kept frozen until placement, they were then thawed to room temperature and immediately placed in their cage. The same type of cages that were constructed for the meat model were used for the three pig fetuses as well (wire mesh and rebar). The weights of each pig as well as their placement and removal dates can be seen in Table 3.
Two samples of approximately 8-10 grams of soil each were taken from each of the three designated areas of the cadaveric region, located directly underneath the pigs, for a total for six samples for each decomposition interval. The diagram of the three designated areas, of the cadaveric region can be visualized in Figure 3. Two samples were taken from each cadaveric region (region a, b and c). Each of the decomposition sites were weekly sampled for a period of four weeks. A control área was also sampled from each week, for a total of 21 samples each week, and 84 samples overall. Control samples were taken each week to monitor volatile odor patterns over time. During each sampling period, weather conditions such as the temperatura and humidity were recorded, as well as the soil moisture and pH for that particular sample location.
Each individual sample was collected in a sterilized 15mL glass vial then wrapped in parafilm to prevent VOC seepage during the equilibrium period. Samples vials were allowed to equilibrate for 24 hours at room temperature in the laboratory and then the SPME fiber was exposed for 24 hours for a heated extraction at40°C.After exposure the SPME fiber was retracted back into the SPME holder until the sample was manually injected into the GC-MS for analysis. The samples were run on the GC-MS immediately following the 24 hours fiber exposure period.
All generated data was analyzed using Chemstation software (Agilent Technologies, Santa Clara, CA) and the National Institute of Standards and Technology mass spectral library (NIST 2017) for compound identification. Compounds known to be products of the column or sampling process were not included in the analysis. For statistical analysis, multifactorial analysis of variance (ANOVA) was used for high frequency detected compounds.This specific statistical analysis was able to determine if there was a statistically significant difference between compound abundance and the time of collection after cadaver removal, as well as the effect between compound abundance and the time of contact between the cadaver and the soil. This analysis determined how the mean of the peak area for the target decomposition VOC depended on both post-removal collection time and remains surface contact time. Means were used to compare the differences, and Tukey's honestly significant difference (HSD) test was applied to compare the mean values. The significance level for the ANOVA analyses was p <0.05.
Results & Discussion
Pork Meat Model
Samples were collected weekly for each surface-contact time (14, 17, and 21 days) after removal of the meat and a blank, collection times included 0, 168, 336, 504, 672 and 840 hours post removal. During each sample collection temperature, humidity, soil pH and moisture were recorded. A total of 108 samples were collected during the six- week sampling period yielding a total of 118 different compounds being detected among the 3 decomposition contact periods.
A compiled chemical class odor ratio of detected compounds across the decomposition intervals studies revealed that the highest number of ketones were detected in the samples collected immediately following removal of the decomposing meat. As the presence of ketones faded, the presence of alkanes increased as the post removal time increased. Figure 4 shows the number of compounds detected in each functional group over the six-week sampling period. As can be observed, the number of detected volatiles decreased as the soil collection time increased post-meat removal. From the zero hours post-removal variable, it can be inferred that the longer contact time between the putrefactive meat source and the soil surface does not have a profound effect on the number of detected volatiles as does the time of soil collection post-removal. This was anticipated because the residual odor left by the meat source should have been highest at this point, as it was the closest measurement to the point at which the remains were present on the soil surface.
Environmental conditions such as temperature, humidity and soil moisture can influence the number of VOCs detected in the sample. These parameters directly affect the breakdown of proteins and carbohydrates as well as microorganisms present in the soil (Iqbal et al. 2017). Figure 5 shows the average temperature, humidity, pH and moisture during the set sampling times. While the pH and moisture content of the soil did not highlight a marked fluctuation, it can be seen that the average temperature and humidity did fluctuate each week. The increase in humidity during hours 0, 336, and 504 could be responsible for the increase in number of VOCs found in those samples. Figure 6 shows the number of VOCs detected during each set sampling time frame, along with the recorded temperature and humidity at the time of sample collection. Increased soil moisture at the surface level may cause certain chemical classes such as hydrocarbons to recede into the soil where water is not accumulated, allowing them to be present for longer periods of time. Previous studies have corroborated that hydrocarbons are a dominant chemical class in soils from burial sites (Brasseur et al. 2012). Furthermore, higher humidity can also impact conditions for microbial and insect activity which in turn affects the number and abundance of detected VOCs (Forbes et al. 2014).
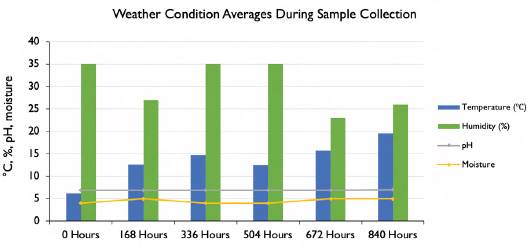
Source: Own elaboration.
Figure 5 Graph showing the varying weather and environmental conditions during the six-week sampling period.
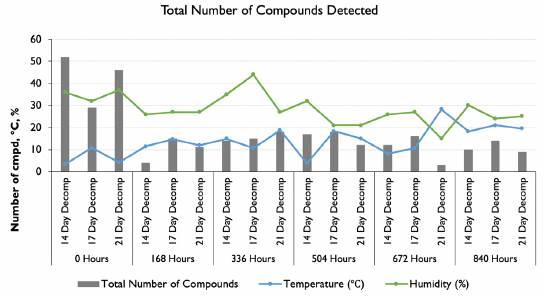
Source: Own elaboration.
Figure 6 Total number of compounds detected during each sampling period and corresponding weather conditions.
The sampling period post-removal with the most abundant VOC detection was those collected immediately after removal (0 hours). This was to be expected as the residual decomposition odor was at its highest, as these samples were taken closest to being in contact with the decomposing tissue models.
As expected, the number of compounds diminished during each sampling period. A table showing the number of compounds from each different functional group found during the 0-hour sampling period can be seen in Table 4.
Table 4 Number of Compounds Detected at the 0 Hour Post-Removal Sampling by Functional Group.
14 Day Decomposition | 17 Day Decomposition | 21 Day Decomposition | |
---|---|---|---|
Alcohols | 5 | 3 | 3 |
Sulfides | 6 | 6 | 7 |
Dienes | 4 | 1 | 0 |
Alkene | 10 | 4 | 8 |
Ketones | 25 | 26 | 28 |
Aldehyde | 0 | 0 | 1 |
Alkane | 5 | 2 | 13 |
Carboxylic acid | 2 | 5 | 5 |
Aromatic | 3 | 1 | 3 |
Nitrogen containing | 4 | 3 | 2 |
Carboxyl | 1 | 0 | 0 |
Other | 6 | 0 | 3 |
Both ketones and alkenes increased as the decomposition period increased. This corroborates previous studies in which ketones are predominantly detected during active and advanced stages of decay (Forbes et al. 2014, Dekeirsschieter et al. 2009). The presence of alcohols, dienes, and nitrogen containing compounds decreased as the decomposition period increased. There was a decrease in alkenes and alkanes during the 17-day decomposition contact but then increased again at the 21-day decomposition contact period.
Ketones were found in large quantities in the immediate post-contact sampling period (0 hours) but quickly diminished by the 504-hour sampling time, this was seen for all three decomposition contact time periods. Figure 7 shows the average abundances of the detected ketones for all three decomposition contact periods and sampling periods 0 through 504 hours post removal. As was observed, the longer the post-contact soil sample took place, a general decrease in overall VOC detection was observed. Hence, regardless of the high presence of the ketone chemical class in the immediate post-contact sampling, it was to be expected for this chemical class to show a general decrease as post-contact collection continued over time. Multiple factors come into play when it comes to decomposition VOC odor profiles. While the 3 decomposition time periods highlighted a comparable abundance of ketones immediately following removal of decomposed tissue, the results highlight the dynamic nature of VOC production across time and the difficulty in achieving predictable patterns.
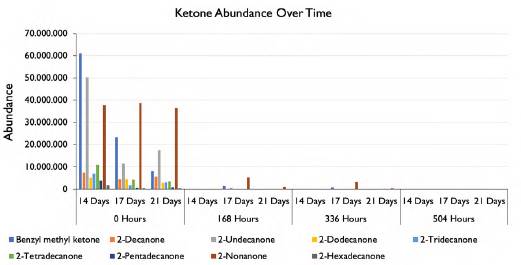
Source: Own elaboration.
Figure 7 Ketone abundance over time in pork meat model, collection time of 672 hours and 840 hours excluded due to no detected ketones.
2-Nonanone and benzyl methyl ketone are the only two ketones that persisted after the immediate post-removal sampling time (0 hours). All the other ketones were not present in the remaining samples for any of the allotted decomposition contact periods studied. The majority of the ketones detected in all three decomposition contact periods had higher detected abundances for the 14- and 21-day decomposition contact periods. However, 2-Nonanone had a higher average abundance detected in the 17-day decomposition contact period.
As discussed previously, there was a large number of ketones detected in all three decomposition contact times at 0 hours post-removal. Ketones persisted longer in the 17- and 21-day decomposition contact periods, as some ketones were still being detected at the 336 hours post-removal sampling time. However, for the 14-day decomposition contact period ketones were only detected at the 0 hours post-removal sampling interval. Dienes and nitrogen containing compounds were only seen in the 0-hour post-removal sampling interval for all three decomposition periods (14-, 17-, and 21 days). For the 14-day decomposition contact period, the presence of alkanes increased until plateauing at the 504 hours post-removal sampling period then declined. For the 17-day decomposition period the highest number of alkanes was seen in the 840 hours post-removal samples. In the 21-day decomposition, the highest number of alkanes detected were present in both the 0 hours post-removal and the 336 hours post-removal samples. The number of sulfides peaked for the 21-day decomposition sample at 168 hours post-removal, but peaked for the 14- and 17-day decomposition samples at 336 hours post-removal. These peaks in certain functional groups can be visualized in figure 8, which shows a comparison of each decomposition contact period at the different post-contact sampling intervals.
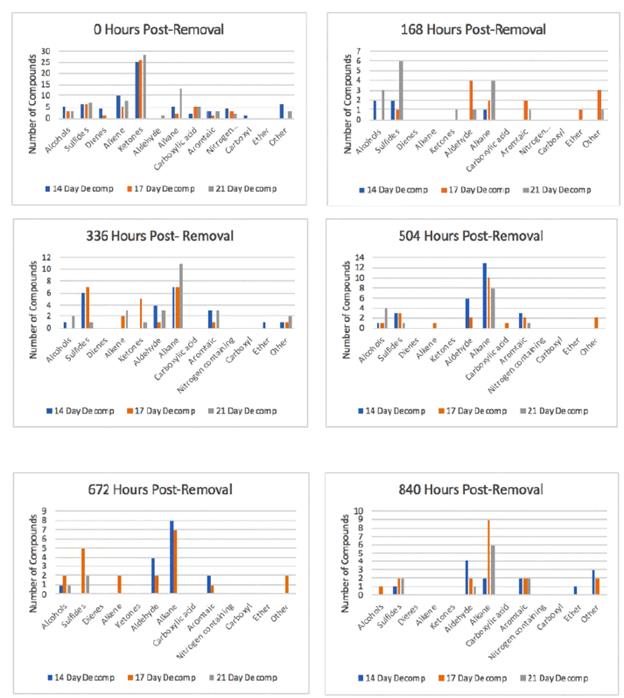
Source: Own elaboration.
Figure 8 Average number of compounds detected per sampling period, arranged by functional groups.
To understand interactions between key volatiles detected from soil samples and study questions, medium to high frequency compounds were selected by identifying those who appeared 5 or more times for each decomposition contact period. Medium frequency were compounds that appeared 5 to 8 times, while high frequency compounds were those that appeared over 9 times. There was a total of 16 high frequency compounds for all decomposition times. The high frequency compounds included two sulfides (disulfide, dimethyl and dimethyl trisulfide), six ketones (benzyl methyl ketone, 2-decanone, 2-undecanone, 2-dodecanone, 2-tetradecanone and 2-nonanone), two aldehydes (nonanal and octanal), five alkenes (decane, 3,6-dimethyl-, dodecane, pentacosane, tetradecane, l-iodo-, and undecane, 5-methyl-), and one aromatic compound (furan, 2-pentyl-). The only two high frequency compounds found in the blank sample that were analyzed each week were two alkanes (decane, 3,6-dimethyl- and dodecane). Decane, 3,6-dimethyl was found in 6 of the 18 blank samples, while dodecane was found in 10 of the 18 blank samples. These are two compounds that could possibly be from the soil, however they were not present in all the soil samples. Table 5 depicts the medium to high frequency compounds found in each decomposition contact time.
Table 5 Medium and high frequency compounds found in each decomposition contact time in the meat-simulation model
14 Days | 17 Days | 21 Days | Compound | Frequency |
---|---|---|---|---|
X | X | X | Disulfide, dimethyl | High |
X | X | X | Dimethyl trisulfide | High |
X | X | X | Benzyl methyl ketone | Medium |
X | X | X | 2-Decanone | Medium |
X | X | X | 2-Undecanone | Medium |
X | X | 2-Dodecanone | Medium | |
X | X | X | 2-Tetradecanone | Medium |
X | X | 2-Nonanone | Medium | |
X | X | X | Nonanal | High |
X | Octanal | Medium | ||
X | X | Decane, 3,6-dimethyl- | High | |
X | X | X | Dodecane | High |
X | Pentacosane | Medium | ||
X | Tetradecane, 1-iodo- | Medium | ||
X | Undecane, 5-methyl- | Medium | ||
X | X | X | Furan, 2-pentyl- | High |
Using a multifactorial ANOVA model, high frequency compounds were evaluated as a function of both meat contact time on soil surface and post-removal soil collection time in terms of abundance of target compounds. These targeted compounds were identified at all decomposition contact periods. For dimethyl disulfide a p-value of 0.0001 was found for peak area by collection time, the p-values for peak area by contact and the interaction between collection time and contact time were not found to be significant, this plot can be visualized in Figure 9. For dimethyl trisulfide a p-value of <0.0001 was found for peak area by contact time and a p-value of <0.0001 was found for peak area by collection time.. The analysis using the compound nonanal produced a p-value of 0.0115 for peak area by contact time and a p-value of 0.0022 for peak area by collection time.
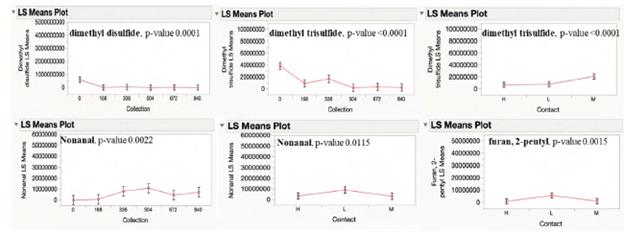
Source: Own elaboration.
Figure 9 AMulti-factorial ANOVA Results for High Frequency Compounds - Meat Simulation Mode.
Furan, 2-pentyl was only found to have significance for the peak area by contact time with a p-value of 0.0015.There was no statistical significance from the ANOVA analysis for dodecane. The results reinforce the notion that the amount of time a decomposing tissue is allowed to come into contact with the soil environment directly affects the amount of compound abundance detected. Furthermore, for previously reported decomposition compounds such as dimethyl trisulfide and nonanal, the post-contact collection time also highlighted a strong relationship with the amount of compound detected [Armstrong 2016, Cablk 2017, Degreeff 2011, Dekeirsschieter 2012, Forbes 2014, Furton 2015, Hoffman 2009, Nizio 2017, Perrault 2014, Perrault 2015, Vass 2004, Vass 2008, Vass 2012].
Sus scrofa Trial
Pig cadavers were used to replicate the meat model with a more acceptable human analogue. Samples were collected weekly for each decomposition contact time (14, 17, and 21 days) after removal of the pig carcass and a blank, collection times included 0, 168, 336, and 504 hours post removal. During each sample collection temperature, humidity, soil pH and moisture were recorded. A total of 84 samples were collected during the four-week sampling period yielding a total of 90 different compounds being detected among the 3 decomposition contact periods. The pigs were mummified due to the decreasing temperatures during the various decomposition contact times. The pigs appeared to have reached the bloated stage of decomposition before becoming mummified.
A compiled chemical odor ratio of detected compounds across the decomposition intervals studies revealed that the highest number of ketones were detected in the samples collected immediately following removal of the decomposing remains. Sulfides were present only within the 0-hour post-removal samples, while the level of alkanes increased after the 0-hour post-removal time period. Alkanes are typically observed in higher abundance in soil from under the body rather than along the cadaver's edge [Dubois 2018]. Figure 10 shows the number of compounds detected in each functional group over the four-week sampling period. As can be observed, the alkane functionality is prevalent across the post-contact sampling period, thereby confirming already established literature results with regards to decomposing tissue contact in soil terrain.
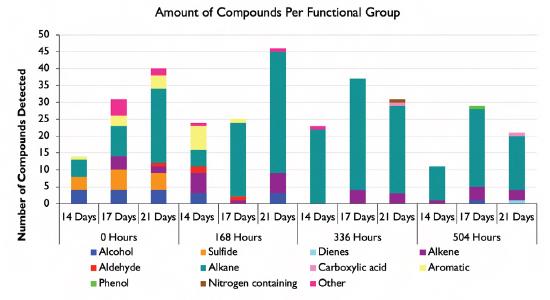
Source: Own elaboration.
Figure 10 Graph showing the number of compounds detected during each sample collection, arranged by functional groups.
Figure 21 shows the average temperature, humidity, pH and moisture during the set sampling times. During the collection period the average temperature was 17.6°C for 0 hours collection, 12.5°C for 168 hours collection, 15.8°C for 336 hours collection and 13.4°C for 504 hours collection. The average humidity during the 0-hour collection was 25%, it was 22.3% for the 168 hours collection, 24% for the 336 hours collection and 32.7 for the 504 hours collection. The soil pH had an average of 7 for the 0 hours collection, 6.8 for the 168 hours collection, 6.9 for the 336 hours collection and 5.8 for the 504 hours collection. The average soil moisture was 4.8 for the 0 hours collection, 5.2 for the 168 hours collection, 4.8 for the 336 hours collection and 3.7 for the 504 hours collection. While the pH and moisture content of the soil did not fluctuate significantly, it can be seen that the average temperature and humidity did vary each week. The increase in humidity during hours 0 and 504 could be responsible for the increase in number of VOCs found in those two samples. Figure 12 also shows the number of VOCs detected during each set sampling time frame, along with the recorded temperature and humidity at the time of sample collection. The VOC profile had the most complex chemical class mixture following immediate soil collection post remains removal. Again, this was to be expected as this was the closest measurement to when the remains were in actual contact with the collected soil surface. However, as the hours of soil collection post remains removal increased, there were still decomposition VOCs detected in the soil matrix, with the alkane functionality having an increased ratio distribution. The majority of compound classes were greatly reduced after removal of carcass from the deposition site, which corroborates with parallel studies in soil residual odor work (Perrault et al. 2015). The notion that there is detectable decomposition VOCs after 504 hours post-remains removal, indicates that some of these alkane type of compounds persists even in a cold, arid climate as that found in the West Texas region.
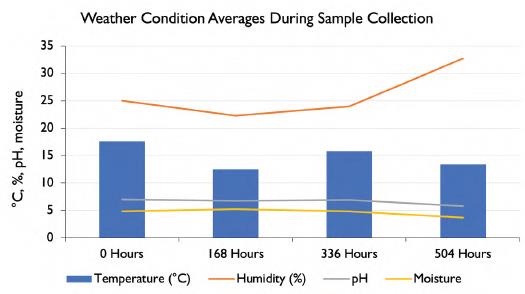
Source: Own elaboration.
Figure 11 Total number of compounds detected in relation to the temperature and humidity.
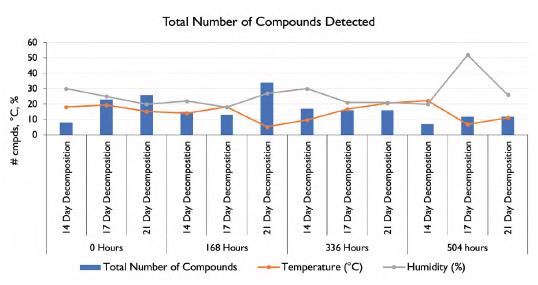
Source: Own elaboration.
Figure 12 Total number of compounds detected in relation to average temperature and humidity.
After the 0 hours post-removal sampling period for all three decomposition contact times the presence of sulfides had completely diminished in the Sus scrofa trials. Alkanes dominated all four sampling periods for all three decomposition periods. The presence of alkane compounds peaked for the 21-day decomposition period at the 168 hours post-removal collection. Perhaps, the most notorious increment of the alkane functionality can be observed in the 336 hours post-removal period, where this functional group was the most detected across all contact times.
To understand interactions between key volatiles detected from soil samples underneath the pig carcasses, high frequency compounds were selected by identifying those who appeared 5 or more times for each decomposition contact period. Medium frequency were compounds that appeared 5 to 8 times, while high frequency compounds were those that appeared over 9 times. Using a multifactorial ANOVA model, only high frequency compounds were evaluated as a function of both carcass contact time on soil surface and post-removal soil collection time in terms of abundance of target compounds. The selected compounds for statistical analysis were only the high frequency compounds detected at all three decomposition contact times. For the pig carcass model, there was only one compound that satisfied this condition = dodecane. There was no statistical significance observed in the one high frequency compound, dodecane. The resulting plots and p-values can be seen in Figure 13. The results imply that with the pig carcass study, the remains contact time with the soil surface and the post-collection time period had no effect on the peak abundance of the selected frequently occurring alkane compound. This could be a direct effect of another external variable such as temperature, which can ultimately have a stronger drive on the abundance of that specific volatile. The model only looked for differences as a function of the tested variables, however, the results demonstrate that even when utilizing a commonly detected volatile, the decomposition odor picture is complex and specific patterns are difficult to establish due to the number of parameters acting simultaneously at a given site. It might well be that other non-frequently occurring compounds might contribute to the residual odor picture to a greater extent.
Meat Model and Sus Scrofa Trial Comparison
For the present study, both a pork meat model and a Sus scrofa model were utilized to determine how residual decomposition odor VOCs persisted and changed in the soil after removal of the cadaver analogue. In both studies, samples were collected weekly starting at 0 hours post-removal. Environmental conditions such as temperature, humidity, soil pH and moisture content were recorded. The study was a qualitative approach to determinewhat compounds were present and their relative abundance at set decomposition contact intervals and sample collections periods post cadaver removal.
As hypothesized, the detected compounds decreased in abundance as the sampling time post removal increased. This corroborates the idea of residual odor diminishing over time. The meat model highlighted that the VOCs present in the soil after the contact period, indeed change in the chemical composition over time. A key example of this would be the presence and abundance of ketones in all three-contact times and how they diminished by the 504hour post-removal collection time. The secondary hypothesis of VOCs being more abundant initially due to the length the cadaver was left in contact with the soil was found to be true for some of the compounds. In the meat model, the ANOVA analysis for dimethyl trisulfide of peak area by contact time had a significant p-value of <0.0001, showing that there was a statistically significant correlation between the peak abundance and contact time. This was also found to be true with nonanal whose p-value for peak area by contact time was 0.0115, and furan, 2-pentyl who has a significant p-value of 0.0015. Not only does this show that cadaver contact-time had a direct effect on peak abundance, but these compounds that were found to be statistically significant are also commonly detected decomposition compounds in the literature [Armstrong, 2016., Cablk, 2012., Degreeff, 2011., Dekeirsschieter, 2012., Forbes, 2014., Furton, 2015., Hoffman, 2009., Nizio, 2017., Perrault, 2014., Perrault, 2015., Vass, 2004., Vass, 2008 & Vass 2012].
One of the main unexpected outcomes was that theoretically, there should have been a higher cumulative abundance seen in the initial collection time for the longer contact time (21-days), however this was not seen in either the meat model or the Sus scrofa models. This can be explained by the lower temperature and humidity during the 21-day contact times versus the 14-day and 17-day contact periods during the immediate 0-hour post-removal samples. This experimental result reinforces the idea that temperature and humidity have a stronger effect on residual odor traces than the time a decomposing tissue is allowed to come in contact with a surface [Cockle, 2015]. In terms of cadaver dog operational performance, it is important to note that even though a decomposing remains has been exposed to the soil surface for an extended amount of time, this does not equate to higher content of residual odor in colder climates.
One of the main differences between the meat model and the Sus scrofa models was the duration of the sampling timeline. The meat model sampling lasted a total of six weeks, while the Sus scrofa model only lasted four weeks. Three duplicates of each decomposition contact time were utilized for the meat model, whereas there was only one pig used for each decomposition contact time for the Sus scrofa trial due to limitation on carcass acquisition. When comparing the results of the meat model to the Sus scrofa trial only the first four weeks of the meat model will be compared. Overall, there were more compounds found during each sampling period in the meat model than during the Sus scrofa trial. This could be attributed to a higher ambient temperature when the meat model was being conducted versus when the pig carcasses where left to decompose. As it is readily known in decomposition processes, lower temperatures slow down the putrefaction process sometimes even leading to mummification, yielding to lower delivery of fluids to the surrounding environment.
One major difference between the two models is seen during the 0-hour post removal samples for all three decomposition contact periods. The presence of ketones is only observed in the meat model. Figure 14 depicts chemical class distribution pie charts used to compare the abundance of each functional group present for the meat and carcass models at each sampling time. These pie charts represent the absolute abundance of each functional group. The meat model samples for the 0-hour post removal also had a higher number of functional groups detected than the Sus scrofa soil samples. However, for both the meat and carcass models, aldehydes are only detected in the 21-day decomposition contact period for the 0 hours post removal samples.
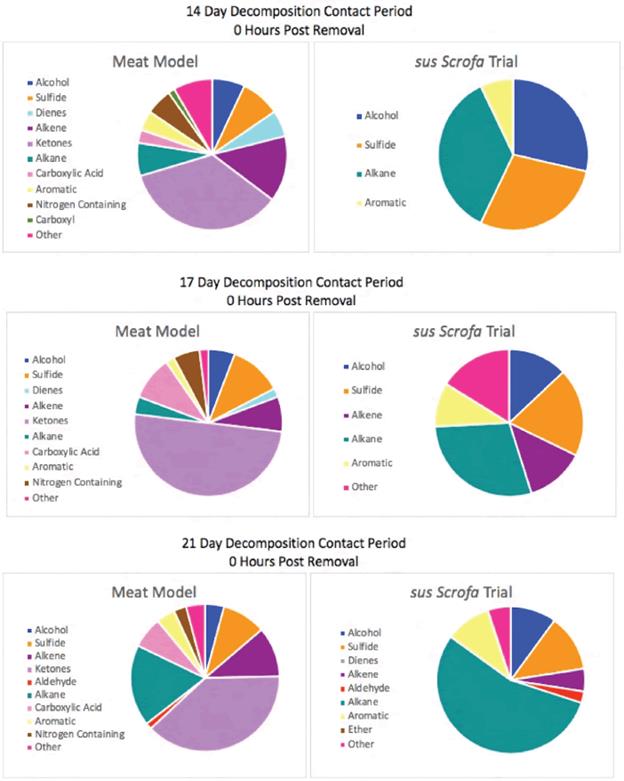
Source: Own elaboration.
Figure 14 Comparison of the functional groups found in meat model and the Sus scrofa model for the 0-hour post removal samples.
During the 168 hours post removal sample the carcass soil samples did not show ketones while the meat model did. The presence of sulfides had completely diminished in the 168 hours post removal samples for the carcass trial however, the sulfides remained in the meat model samples. Aldehydes were present in the 14-day decomposition period for the 168 hours post removal sample for the Sus scrofa trial but they were not detected in the meat model for the same decomposition period. For the meat model, sulfides and alcohols made up the majority of the detected compounds at this sampling hour, while for the Sus scrofa trial the more abundant compounds were found to be alkanes and alkenes. Previous literature has shown that alkanes have been shown to dominate the VOC profile in soils collected from burials [Brasseur, 2012, Perrault, 2015]. This abundance of alkanes and alkenes was seen in the work of Perrault et al. in 2015 as well [Perrault, 2015].
During the 336 and 504 hours post removal sample collections the only similarity between the meat model and the Sus scrofa trial was the presence of alkanes dominated the samples during this collection time for all three decomposition periods (See Figure 15). As previously stated, the high number of alkanes has been linked to soil samples collected from burial sites [Brasseur, 2012, Perrault, 2015]. Brasseur et al. 2012 found that the main reason for this prevalence of alkanes beneath the carcass is the "leaching and percolation of degradation fluids due to gravity [Brasseur, 2012].
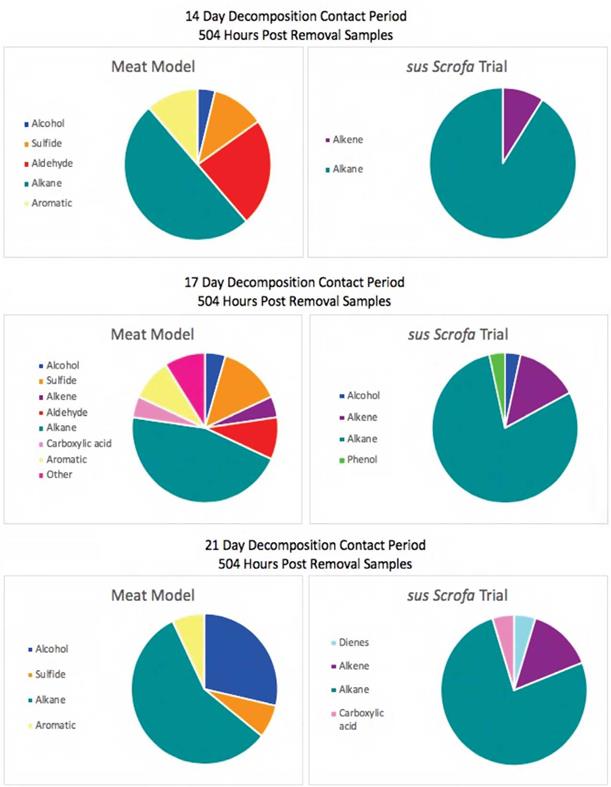
Source: Own elaboration.
Figure 15 Comparison of the functional groups found in meat model and the Sus scrofa model for the 504-hour post removal samples.
While the results of the meat model and the pig carcass model have vast differences between them, they both provided proof that previously established decomposition odor compounds can be detected in the soil post-removal of both a porcine meat model and a pig carcass. This concept had been proven by Alexander et al. in 2015 by having HRD dogs produce positive alerts to soil sample taken from both under decaying cadavers, as well as residual odor soil samples. These certified HRD dogs were able to positively alert on soil samples that had post-cadaver removal periods of up to 667 days with 85.7% accuracy. However, this study did not utilize instrumental analysis of the soil samples to determine the VOCs that were present. While the positive alerts from the HRD dogs does prove that there is detectable residual decomposition odor in the soil, it did not provide any knowledge as to the compounds being detected by the HRD dogs.
The findings of this study also corroborate the work of Perrault et al (2014, 2015a, 2015b). Who conducted similar studies in Australia. However, the environment for those studies differs vastly from the climate in which this study was conducted. The analytical techniques employed also differed, which have a direct impact in the characterization of the collected VOCs. As with those studies, the presence of VOCs greatly diminished after the initial collection period and hydrocarbons or alkanes were the most prevalently observed compounds throughout the time frame under evaluation. It is important to note that our study also focused on much shorter post-removal soil collection times, thereby capturing fresh-advanced decay decomposition stages as opposed to full skeletal remains.
Conclusion
The complex understanding of decomposition residual odor left in soil surfaces after cadaver removal has seen a focus in forensic science research over the last few years. Currently, there is a lack of understanding as to how this odor profile changes over time as a function of environment, scavenging and postmortem intervals. This study investigated the persistence of decomposition odor volatiles in a West Texas environment using both a porcine meat and pig carcass model for analysis over a one-month duration. Both models highlighted an increase in number of detected compounds and a more complex chemical class distribution in soil samples collected immediately post-remain removal, while showing a reduced number of compounds and chemical class types as quickly as the second week of collection. Preliminary results suggest that remains contact time has a more profound effect at warmer temperature than at colder temperatures, which can directly affect both biological and chemical detection of victims and/or clandestine gravesites. These results provide information on an alternate geographical location (arid, cold region) as to the decomposition VOC signature that remains at a deposition site which can be useful to supplement current knowledge for optimized cadaver dog training when no human remains are physically present on site.