Services on Demand
Journal
Article
Indicators
-
Cited by SciELO
-
Access statistics
Related links
-
Cited by Google
-
Similars in SciELO
-
Similars in Google
Share
Earth Sciences Research Journal
Print version ISSN 1794-6190
Earth Sci. Res. J. vol.11 no.1 Bogotá Jan./June 2007
CRUSTAL THICKNESS VARIATIONS AND SEISMICITY OF NORTHWESTERN SOUTH AMERICA
Orlando Hernandez-Pardo1,2, Ralph R. B. von Frese 1, Jeong Woo Kim3
(1)School of Earth Sciences, The Ohio State University, Columbus, OH 43210 USA, FAX 614 2927688.
E-mail: hernandez.135@osu.edu, vonfrese@geology.ohio-state.edu.
(2)Dept. of Geosciences, Universidad Nacional de Colombia, Bogotá, D.C. COLOMBIA
E-mail:ohernandezp@unal.edu.co
(3)Dept. of Geoinformation Engineering, Sejong University, Seoul, KOREA.
E-mail:jwkim@sejong.ac.kr
Manuscript received December 21 2006. Accepted for publication June 19 2007.
ABSTRACT
Any uncompensated mass of the northern Andes Mountains is presumably under pressure to adjust within the Earth to its ideal state of isostatic equilibrium. Isostasy is the ideal state that any uncompensated mass seeks to achieve in time. These pressures interact with the relative motions between adjacent plates that give rise to earthquakes along the plate boundaries. By combining the gravity MOHO estimates and crustal discontinuities with historical and instrumental seismological catalogs the correlation between isostatically disturbed terrains and seismicity has been established. The thinner and thicker crustal regions were mapped from the zero horizontal curvature of the crustal thickness estimates. These boundaries or edges of crustal thickness variations were compared to crustal discontinuities inferred from gravity and magnetic anomalies and the patterns of seismicity that have been catalogued for the last 363 years. The seismicity is very intense along the Nazca-North Andes, Caribbean-North American and North Andes-South American collision zones and associated with regional tectonic compressional stresses that have locally increased and/or diminished by compressional and tensional stress, respectively, due to crustal thickness variations. High seismicity is also associated with the Nazca-Cocos diverging plate boundary whereas low seismicity is associated with the Panama-Nazca Transform Fault and the South American Plate.
Keywords: Crustal thickness, Gravity, Seismicity, Northwestern South America.
RESUMEN
Cualquier masa sin compensar al norte de las Montañas de los Andes se encuentra presumiblemente bajo presión para ajustarse en la Tierra a su estado ideal de equilibrio isostático. La Isostasia es el estado ideal que cualquier masa sin compensar busca a través del tiempo. Estas presiones interactúan con los movimientos relativos de las placas adyacentes para producir terremotos a lo largo de los límites entre las placas. Al combinar los estimados de la gravedad de MOHO y discontinuidades de la corteza con catálogos sismológicos históricos e instrumentales, la correlación entre terrenos isostáticamente anómalos y la sismicidad ha sido establecida. Las regiones delgadas y gruesas de la corteza han sido cartografiadas desde la curvatura original cero de los espesores estimados de la corteza. Estoslímites o bordes de las variaciones de espesor de la corteza fueron comparadas con discontinuidades de la corteza inferidas de anomalías magnéticas y gravimétricas, y los patrones de sismicidad que han sido catalogados en los últimos 363 años. La sismicidad es muy intensa a lo largo de las zonas de colisión de Nazca-Norte de los Andes, Caribe-América del Norte y el Norte de los Andes-Sur América y se encuentra asociado con esfuerzos tectónicos regionales compresionales que localmente han aumentado y/o disminuido por esfuerzos compresionales y tensionales respectivamente, debido a las variaciones de espesor de la corteza. La alta sismicidad se encuentra asociada con el límite de placas divergente de Nazca-Cocos, mientras que la baja sismicidad se encuentra asociada con la falla de transformación de Panama-Nazca y la placa suramericana.
Palabras claves: Espesor de la corteza, Gravedad, Sismicidad, Noroeste de Sur America.
INTRODUCTION
The increased number of seismological networks established during the last century led to the discovery that earthquakes are not randomly distributed, but tend to occur along well defined earthquake belts (Shearer, 1999). These belts are largely concentrated along the margins of tectonic plates that shift slowly over geologic time. The relative motions between adjacent plates give rise to earthquakes along the plate boundaries that include spreading oceanic ridges, converging subduction zones, collisional continental plate boundaries, and transform faults along which they shear past each other (Bird, 2003; Turcotte and Schubert, 2002; Cediel et al.,2003). For northwestern South America, the improvement in instrumentation and expansion of seismological networks has led to the production of relatively complete and accurate catalogs of earthquake locations and ground motions.
SPECTRALLY CORRELATED TERRAIN AND FREE AIR GRAVITY ANOMALIES
The isostasy of northwestern South America was investigated considering the topography/ bathymetry data from National Imagery and Mapping Agency (NIMA) from −8°S to 23.5°N latitude and from −90°W to −58.5°W longitude. Surface and bathymetry elevations from the JGP95E terrain data base (Smith and Sandwell, 1994; 1997) were processed to produce the Digital Elevation Model for the water and rock terrain gravity components at 0.5° nodal spacing. Free-air gravity anomalies (FAGA) were estimated from the EGM96 spherical harmonic Earth Gravity Model to degree and order 360 (Lemoine et al., 1998) at 20 km altitude over the 32° x 32° area at 0.5° nodal spacing. The altitude of 20 km was chosen to help minimize the effects of local density errors in the terrain gravity modeling (Leftwich et al., 2005). The terrain gravity effects were modeled in spherical coordinates at 20 km altitude by Gauss-Legendre Quadrature integration (von Frese, 1980). The terrain gravity modeling used densities of 2.8 gm/cm3 for the crust and 1.03 gm/cm3 for oceanic water. Spectral correlation theory was used to analyze the co-registered FAGA and TGE for their anomaly correlations using MatLab (MATHWORKS, 2005). Specifically, the Fourier transforms T and F of TGE and FAGA, respectively, were used to obtain their correlation spectrum (Davis, 1986; von Frese et al., 1997a; Kim et al., 2000) given by:
where CC(k) is the correlation coefficient between the kth wavenumber components F(k) and T(k), and denotes taking the real parts of the wavenumber components. Usually, CC(k) is evaluated from the cosine of the phase difference (Δθk) between the two kth wavenumber components. Using the correlation spectrum between FAGA and TGE, spectral correlation filters were designed to extract terrain-correlated free-air gravity signals. Those wavenumber components showing intermediate to high positive (CCp(k) ≥ 0.3) and negative (CCn(k) ≤ 0.3) correlations were identified. The cut off values for the correlation filter were determined to minimize correlative features between the terrain-decorrelated free air and compensating terrain gravity components. Inversely transforming positively and negatively correlated free-air wavenumber components according to the selected cut off values yielded the terrain- correlated free air gravity anomalies (TCFAGA).
The residual terrain-decorrelated free-air gravity anomalies (TDFAGA) were calculated by subtracting TCFAGA from FAGA, so that FAGA = TCFAGA + TDFAGA (2)
TCFAGA are explained by anomalies associated with the topography while TDFAGA include the gravity effects of sources within the crust (e.g., local bodies) and the subcrust.
CRUSTAL MODELLING
A new crustal thickness model for northwestern South America was developed using the compensated terrain gravity effects (CTGE) that resulted when the TGE were subtracted from the TCFAGA. The CTGE represent isostatically adjusted complete Bouguer anomalies that correspond to the gravity effects of the terrain in isostatic equilibrium. This approach is feasible because 90% of the earth is in equilibrium with the mean global free-air gravity anomaly being zero (Heiskanen and Moritz, 1967). MOHO and related crustal thickness variations were modeled from the CTGE by inversion, assuming the constant nominal density contrast of 0.4 gm/cm3 of the mantle relative to the crust. This methodology has been successfully applied instudies of the mantle-crust interface for East Asia (Tan and von Frese, 1997), Antarctica (von Frese et al., 1999), Greenland (Roman, 1999), Ohio (Kim et al., 2000), and Iceland and the North Atlantic (Leftwich et al., 2005; Leftwich, 2006). Negative CTGE values are located along the eastern Andes Mountains suggesting some degree of partial compensation and thickening of the crust. The CC between the TCFAGA and the CTGE is -0.3377 showing that most of the topography/bathymetry is isostatically disturbed.
Areas where the TCFAGA values are excessively negative or positive are more prone to seismic activity than areas where the TCFAGA values are closer to zero (Song and Simons, 2003). This paper analyzes and compares seismic data from the Advanced National Seismic System (ANSS) and the Red Sismológica Nacional de Colombia (RSNC) catalogs with crustal thickness estimates from gravity anomalies by understanding improved large-scale dynamic models of earthquakes and tectonics. Gravity anomalies along trenches, continental converging margins, crustal discontinuities, mountain ranges, and cratonic areas are compared with their seismic signatures from earthquake data collected over the last 363 years.
ZERO CURVATURE OF CRUSTAL THICKNESS VARIATION
The terrain-correlated free-air anomalies (TCFAGA) in Figure 1 highlight regions with isostatically disturbed crustal features. The zero anomalies mark areas of crustal equilibrium so that the positive and negative anomalies reflect the compression and tension of the crust, respectively. Positive and negative TCFAGA values can mark crust that is isostatically too thin (under-compensated) or thick (overcompensated), respectively, and hence under pressure to equilibrate by the compressive inflow or expansive out-flow of crustal material (Artyushkov, 1973). Thus, these anomalies can reflect lithosphere in subsidence or uplift (Kim et al., 2000; von Frese et al., 1999a), or alternatively dynamic surface topography that is too high or too low, respectively, to be in isostatic equilibrium.
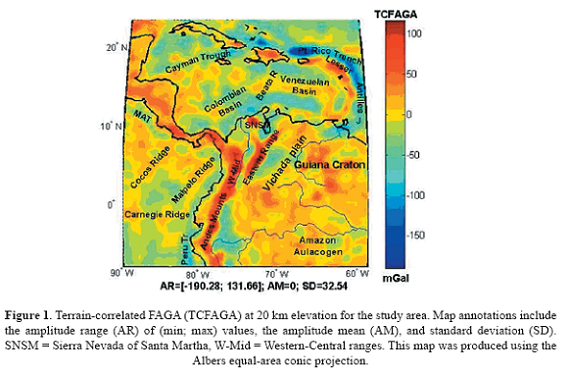
Figure 2 gives the crustal thickness variations for northwestern South America obtained by adding the gravity MOHO estimates (Hernandez, 2006). The zero curvature contour that estimates the edges of thickness variations was obtained by calculating the second vertical derivative of the crustal thickness (Figure 2). The use of vertical derivatives has been always a standard method of enhancing high frequency features in potential field data. Second order vertical derivatives were computed using convolution filters and Laplace’s equation (Sheriff, 1994). The intervening yellow and blue regions of Figure 2 reflect the thicker and thinner crustal components, respectively.
SEISMIC DATA COMPILATION
For comparison with the crustal thickness estimates, the regional seismic data of northwestern South America were compiled from the Advanced National Seismic System catalog (ANSS; USGS, 2006). A more local study was also considered using the historic and instrumental seismic catalogs of the Red Sismológica Nacional of Colombia (RSNC; INGEOMINAS, 2006).
ANSS CATALOG
The ANSS catalog is a world-wide earthquake catalog created by merging the master earthquake catalogs from the contributing ANSS institutions and then removing duplicate solutions (USGS, 2006). The ANSS catalog currently consists of earthquake hypocenters, origin times, and magnitudes. The ANSS database was searched for data from 1966 to 2006 with Ritcher magnitudes M of 3-10.
The earthquake epicenters were converted from geographic to Cartesian coordinates in Figure 2 with the proportional symbols for Ritcher magnitudes given in the legend. Only those events with hypocenters from 0 to 60 km depths were considered in this study. The crustal discontinuities interpreted from the TCFAGA in Figure 1 are also superposed in Figure 2.
The seismic events in Figure 2 are concentrated along the plate boundary zones of the Nazca, Caribbean, North American and South American Plates, the North Andes, Panama and Costa Rica Microplates, the Cocos-Nazca spreading system, and the intraplate discontinuities. They are predominantly located in the thicker crustal sections and along the inferred edges of the thickness variations. However, the thicker oceanic sections of the Nazca, Malpelo and Cocos Ridges in the Pacific and the Beata Ridge in the Caribbean are aseismic.
Comparatively few earthquakes are concentrated in the thinner crustal regions with relatively negative TCFAGA. The Guiana Craton and the interior of Nazca and Caribbean oceanic Plates also lack significant seismic events and are seismically quiescent. To account for the intraplate earthquakes, gravity and magnetic anomalies that infer crustal discontinuities are useful to analyze deformation in the plate interior.
RSNC HISTORICAL AND INSTRUMENTAL SEISMIC CATALOG
The RSNC catalog was created by merging the master earthquake catalogs from the Observatorio Sismológico del Sur Occidente (OSSO), Centro Regional de Sismología Para America del Sur (CERESIS), Instituto Geofísico de los Andes, Red Sismológica Nacional, and regional institutions of northwestern South America and the Caribbean (INGEOMINAS, 2006). This catalog currently consists of earthquake epicenters, origin times, and magnitudes. The RSNC database compiled data from 1643 to 1991 for Ritcher magnitudes from M = 3.0 to M = 10.
The RSNC catalog contains an enormous number of events. Thus, the events were sorted by magnitude for the minor earthquakes (M = 3 to 4), moderate earthquakes (M= 4.1 to 5.0), and strong earthquakes (M > 5.0) as shown in Figures 3, 4, and 5 respectively.
Minor and moderate seismic events in Figures 3 and 4, respectively, are concentrated along the Pacific subduction zone and intracrustal discontinuities of the North Andes Microplate. The concentration of events in the northern part of the eastern cordillera is known as the “Bucaramanga Nest.” Few events are recorded for the Sierra Nevada of Santa Martha, Guiana Craton, and the northwestern flat lands of Colombia at the north of the Andes Mountains. At the continent, areas with thicker crust are more seismically active, while areas of thinner crust are less seismically active. An exception is the Sierra Nevada of Santa Martha with a thick crust and low seismic activity.
Major earthquakes in Figure 5 are located along the converging Nazca-North Andes and South America-North Andes continental boundaries. The major earthquakes are located further landward from the Pacific subduction zone relative to the minor and intermediate earthquakes that are concentrated closer to the coastline. The few seismic events of the Guiana Craton are mostly localized along local crustal discontinuities and are not associated with crustal thickness variations.
RECENT SEISMICITY IN THE RSNC CATALOG
The RSNC started operating since 1992 and has accumulated accurate instrumental information that consists of earthquake epicenters, hypocenters, origin times, and magnitudes. The epicenters were sorted by magnitude for minor events (M= 3 to 4) in Figure 6, moderate events (M= 4.1 to 5) in Figure 7, and major events (M > 5.0) in Figure 8.
The minor and moderate earthquakes in Figures 6 and 7, respectively, define seismic corridors oriented along the major crustal discontinuities of the North Andes Microplate. The Sierra Nevada of Santa Marta also shows more intensive seismic activity than previously recorded by the older data. Major earthquakes in Figure 8 are located along the major crustal discontinuities and plate boundary zones of the North Andes Microplate. In general, seismic events are associated within thicker crust, and therefore can be associated with tensional stress (Artyushkov, 1973).
Hypocenter depths from the modern RSNC were sorted into shallow earthquakes (from 0 to 10 km) in Figure 9, intermediate earthquakes (10 km to 30 km) in Figure 10, deep earthquakes (30 km to 70 km) in Figure 11, and very deep earthquakes (70 km to 300 km) in Figure 12. The tendency of the deepening hypocenters to migrate landward of the Pacific subduction zone is indicative of the oceanic plate subducting under the Andes at an angle that is less than vertical.
DISCUSSION
Gravity and topography are related to seismic activity by the frictional behavior of plate boundary zones and crustal discontinuities. When two plates push against each other, friction between the plates builds up causing the plates to lock. The continued build up of tectonic stress also causes the plates to deform, creating variations in topography and gravity. In addition to deforming the plates, the rocks of the sticking point can break when the stress build up exceeds the breaking strength of the rocks. The resulting strain released at the locking point produces the ground shaking of an earthquake. For northwestern South America, plate tectonic stress plays out notably in the ocean-continent, and ocean-ocean and continent-continent plate collision zones, and also along oceanic spreading ridges and transform faults. In the next sections, these plate boundaries and associated earthquakes zones areas are further analyzed.
OCEAN-CONTINENT COLLISION ZONES
In Figures 2 to 12 the subduction of the Nazca plate under the North Andes microplate is clearly parallel to the Andes Mountains. The collision caused the creation of the Andes Mountains by stacking thrust slices of the crust. The lateral movement of these thrust slices in the welded continent has continued after the collision generating minor, moderate and major earthquakes with shallow, intermediate and deep hypocenter depths defining seismic corridors that are oriented along the subduction zone and the Andes Mountains. The general pattern of the seismicity and focal mechanism solutions of earthquakes are in agreement with the easterly subduction of the Nazca Plate beneath the Andes.
The intensive seismicity along the Middle American Trench (MAT) has a broad pattern reflecting the low angle of inclination of the subducting Nazca and Cocos plates under the Central American Continent. The seismicity along the Caribbean-North Andes plate boundary is relatively low, with few minor (Figures 3, 6, and 9), moderate (Figures 4, 7, and 8), and major (Figures 10 and 11) historical seismic events. This seismicity reflects the predominantly dextral lateral movement of the Caribbean Plate with respect to the North Andes Microplate and South American Plate that may include minor subduction that is incipient when compared with the subduction of the Nazca Plate underneath the Andes Mountains.
OCEAN-OCEAN COLLISION ZONES
Figure 2 shows a broad arcuate seismic zone along the Lesser Antilles. A large number of events occur along the Caribbean-North American plate boundary, which dips to the west away from the underthrusting oceanic plate. Shallow, intermediate, and deep focus earthquakes occur at progressively greater distances from the site of underthrusting. The topographic expression of the trench is largely obscured by thick sediment fill derived from the Orinoco River of Venezuela, but its presence is indicated by the belt of negative TCFAGA in Figure 1. The seismic events are located in thicker oceanic crust associated with the doubling of the collisional oceanic crust. Focal mechanism solutions of the Lesser Antilles reflect regional compressional stress. However, the relatively thicker oceanic crust reflects local tensional stress.
CONTINENT-CONTINENT COLLISION ZONES
The continent-continent collision boundary zone between the North Andes Microplate and the South American plate in Figures 2 to 12 is characterized by intensive seismicity. Minor, moderate, and major historical and recent seismic events in Figures 3 to 8 have occurred along the Llanos Front Fault. Shallow events in Figure 9 can be associated with tensional stress of the sub-Andean thrust belt or fault zone, which is located on a relatively thicker continental crust that implies the local presence of additional tensional stress.
OCEANIC SPREADING RIDGES
Minor and moderate earthquakes occur along the Galapagos spreading ridge and the Panama fracture zone in Figure 2. Focal mechanism solutions indicate regional tensional events associated with plate accretion and strike-slip events where the ridges are offset by transform faults. However, the Panama fracture zone is located on relatively thinner oceanic crust that may be locally associated with compressional stress.
TRANSFORM FAULTS
The Southern Panama Fault Zone constitutes a conservative plate boundary between the Nazca Plate and Panama Microplate, where the plates are in tangential contact and are affected by limited or no subduction or accretion. Therefore, events along this fracture are rare as shown in Figure 2.
CRATONIC ZONE
The intraplate areas of the South American Plate are relatively aseismic. However, a few minor, moderate, major historical and recent seismic events in Figures 3 to 8 have occurred at shallow, intermediate, deep, and very deep hypocenter depths in Figures 9 to 12. These events are located along some of the crustal discontinuities interpreted from the surface FAGA in Figure 1. Their occurrences suggest the presence of continental sutures in the South American plate, with infrequently seismic activity. Although, these earthquakes are rare, they are important because they indicate the nature of directions of stress within the South American Plate. The instrumental seismic events in the above plate boundary zones are analyzed in the next section for their focal mechanisms.
FOCAL MECHANISM SOLUTIONS
Figure 13 gives the compressional stress regime for the study region from stress inversions of the focal mechanisms of the instrumental earthquakes of northwestern South America (Vargas and Duran, 2005). These solutions infer the type of faulting or focal mechanisms from the radiated seismic energy. The Costa Rica-Panama-North Andes converging margins show compressional stress oriented west-east, generating the relative movement of local tectonic blocks of the North Andes Microplate. Shallow earthquakes associated with the Pacific subduction zone show compressional stress oriented NW-SE. Vectors of displacement and compressional stress in southwestern Colombia are oriented west-east. The deep seismicity in the Bucaramanga nest reflects compressional stress oriented NNW – SSE associated with the subducting Caribbean Plate under the North Ande microplate. Complementary examples of global focal mechanisms for the study area can also be obtained from the Harvard Centroid Moment Tensor catalog (Harvard, 2006). These results reflect the inversions of long-period body and surface waves for the source moment tensors and best-fitting double-couple solutions.
CONCLUSIONS
Seismicity in northwestern South America is mainly restricted to the plate boundary zones of the Caribbean, North America, and South America Plates and the Panama, Costa Rica and North Andes Microplates. Seismic corridors are oriented predominantly SW-NW, showing their association with compressional stress generated by the convergence of the Nazca and South American plates, and the shortening of the continental crust and mountain building of the Andes Mountains. The seismicity is extremely high along the northern margin of the Pacific subduction zone.
The hypocenter depths show the tendency of the earthquakes to migrate from the coastline eastwards as the hypocenter depths increase, confirming the eastward motion of the Nazca Plate under the North Andes Microplate. The spatial distribution of earthquakes is consistent with crustal discontinuities interpreted from the gravity anomalies. Seismic events are preferentially concentrated in zones of relatively thicker continental crust. This crust is inherently under tension by virtue of its enhanced thickness, but it is also being subjected to overwhelming compression from plate subduction forces. The analysis of the isostatic state of compensation from terrain and gravity anomalies of northwestern South America help to differentiate probable earthquake zones. Integrating the local stress of the crustal thickness variations with regional tectonic stresses may help to predict local ground motions. The focal mechanisms reflect regional stress and more local stress from the thickness variations of the crust.
REFERENCES
1. Artyushkov, E.V. (1973). Stresses in the lithosphere caused by crustal thickness inhomogeneities. Journal of Geophysical Research. 78. 7675-7708. [ Links ]
2. Bird, P. (2003). An Updated digital model of plate boundaries. Geochemistry, Geophysics Geosystems G3. 4 (3). 1-52. [ Links ]
3. Cediel, F., Shaw, R. P., and Caceres, C. (2003). Tectonic assembly of the Northern Andes Block. The Circum-Gulf of Mexico and Caribbean Hydrocarbon habitats, basin formation and plate tectonics: AAPG Memoir. 79. 815-848. [ Links ]
4. Geosoft (2006). Geosoft Oasis Montaj: Software for Earth Sciences Mapping and Processing. http://www.geosoft.com. [ Links ]
5. Harvard, (2006). Centroid Moment Tensor Catalog (CTM). http://www.seismology.harvard.edu/CMTsearch.html. [ Links ]
6. Hernandez, O. (2006). Tectonic analysis of northwestern South America from integrated satellite, airborne and surface potential field anomalies. PhD Dissertation, Department of Gelogical Sciences. The Ohio State University, Columbus, OH. USA. 177 pages. [ Links ]
7. INGEOMINAS, (2006). Red Sismológica Nacional de Colombia (RSNC) Catalogo de sismos. [ Links ]
8. Kim, J.W., von Frese, R.R.B., and Rae, K.H. (2000). Crustal modeling from spectrally correlated free-air and terrain gravity data A case study of Ohio. Geophysics. 65 (4). 1057-1069. [ Links ]
9. Shearer, P. M. (1999). Introduction to Seismology. Cambridge University Press, New York. 259 pages. [ Links ]
10. Song T. R. A. and Simons, M. (2003). Large trench parallel gravity variations predict seismogenic behavior in subduction zones. Science, Reports. 301 (5633). 630-633. [ Links ]
11. Turcotte D.L. and Shubert, G. (2002). Geodynamics, second edition. Cambridge University press, New York. 456 pages. [ Links ]
12. USGS, (2006). Advance National Seismic System (ANSS). http://earthquake.usgs.gov/research/monitoring/anss/ [ Links ]
13. Vargas C. A. and Duran, J. P. (2005). State of stress of northwestern South America. Earth Sciences Res.J. 9 (1). 41-49. [ Links ]
14. von Frese, R. R. B. and Tan, L. (1999). Antarctic crustal modeling from spectral correlation of free-air gravity anomalies with the terrain. Journal of Geophysical research. 104, B11, 25- 275-25,296. [ Links ]