Servicios Personalizados
Revista
Articulo
Indicadores
-
Citado por SciELO
-
Accesos
Links relacionados
-
Citado por Google
-
Similares en SciELO
-
Similares en Google
Compartir
Earth Sciences Research Journal
versión impresa ISSN 1794-6190
Earth Sci. Res. J. v.12 n.2 Bogotá jul./dic. 2008
A NEW APPROACH ON THE TECTONOMETAMORPHIC MECHANISMS ASSOCIATED WITH P-T PATHS OF THE BARROVIAN-TYPE SILGARÁ FORMATION AT THE
CENTRAL SANTANDER MASSIF, COLOMBIAN ANDES
Castellanos A. Óscar M.1, Ríos R. Carlos A.2 and Takasu Akira3
1Programa de Geología, Universidad de Pamplona, Colombia. ocastellanos@unipamplona.edu.co
2Escuela de Geología, Universidad Industrial de Santander, Colombia.
3Geosciences Department, Shimane University, Matsue, Japan.
Manuscript receiver: May 30th, 2008. Accepted for publication: August 24th, 2008
ABSTRACT
Metamorphic pressure and temperature trajectories are generally used as tools to interpret the tectonic history of deformed belts of rocks that record past activity along active plate margins. The pre-Devonian metamorphic sequence of the Silgará Formation forms part of the ancient basement of the central Santander Massif, including pelitic schists, Siliceous schists and intercalation of amphibolites accompanied by marble. This metasedimentary unit has been affected by Barrovian type metamorphism under middle-pressure/high-temperature conditions. This paper presents on the relationship of tectonic processes to pressure-temperature paths development. Detailed petrologic analysis of pelites and related rocks has been used to constrain the P-T history of this region in the eastern colombian cordillera. Mineral assemblages and Geothermobarometric calculations indicate metamorphic temperatures and pressures of 400-600 °C and 4.0-6.5 kbar, respectively.
Key words: central Santander Massif, Silgará Formation, Barrovian type metamorphism, pelitic rocks, petrologic analysis, geotermobarometry.
RESUMEN
Las trayectorias de presión y temperatura de metamorfismo generalmente son usadas como herramientas para interpretar la historia tectónica de cinturones de rocas deformadas que registran antigua actividad a lo largo de bordes de placa activos. La secuencia pre-Devónica de la Formación Silgará forma parte del antiguo basamento que aflora en la región central del Macizo de Santander, incluyendo esquistos pelíticos, esquistos silíceos con intercalación de anfibolitas acompañadas por mármol. Esta secuencia metasedimentaria ha sido afectada por un metamorfismo tipo Barroviense en condiciones de presión intermedia / temperatura alta. Este artículo especula sobre la relación entre los procesos tectónicos y el desarrollo de trayectorias de P-T. Un análisis petrológico detallado de las pelitas y rocas relacionadas ha sido usado para deducir la historia de P-T de esta región. Las paragénesis minerales y los cálculos geotermobarométricos indican temperaturas y presiones metamórficas de 400-600 °C y 4.0-6.5 kbar, respectivamente.
Palabras clave: región central del Macizo de Santander, Formación Silgará, Metamorfismo tipo Barroviense, rocas pelíticas, Análisis petrológico, geotermobarometría.
Introduction
The geological evolution of the Colombian Andes (Figure 1) has been interpreted as a composite margin made up of successively accreted terranes and oceanic island arc sequences from Paleozoic to Miocene ages (Weber et al., 2002), and the most important events took place during Devonian-Carboniferous and Cretaceous times (Restrepo and Toussaint, 1988). The Colombian Andes are divided into three mountain ranges, which from east to west comprise the Eastern, Central and Western Cordilleras, showing different tectonic histories and configurations. According to Cooper et al. (1995), the first two correspond to modern and ancient fold-thrust belts, respectively, and the last one represents an accreted arc. The Eastern Cordillera splits by the southern termination of the left-lateral strike-slip Santa Marta - Bucaramanga fault and the NW-SE trending Santander Massif into two branches that form the Perijá Range in the west and the Mérida Andes in the east.
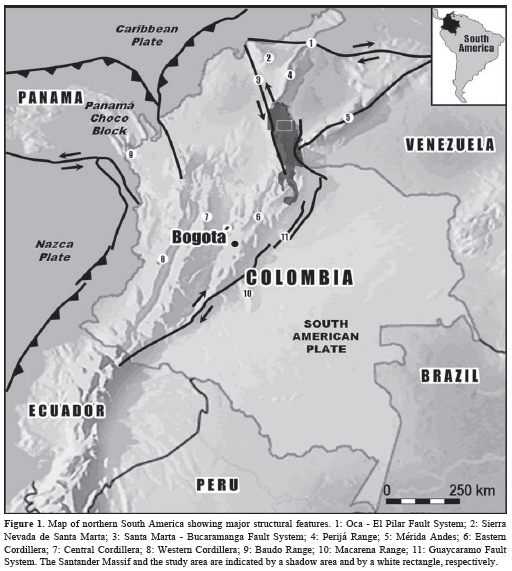
Ward et al. (1973) did a detailed study on the regional geology of the Santander Massif. The Santander Massif exposes its metamorphic basement, which is composed by deformed and metamorphosed rocks that have been tectono-stratigraphically divided into three metamorphic units (Bucaramanga Gneiss Complex, Silgará Formation and Orthogneiss) and cut by intruSive bodies the emplacements of which record Caledonian to Jurassic magmatic events (Goldsmith et al., 1971; Banks etal., 1985; Boinet et al., 1985; Dörr et al., 1995; Restrepo-Pace, 1995; Ordoñez, 2003; Ordóñez and Mantilla, 2005). The Bucaramanga Gneiss Complex is a sequence of high-grade metamorphic rocks (up to the upper amphibolite facies), which is mainly composed of pelitic gneisses with minor amounts of amphibolites and orthogneisses, and in part migmatitic.The Silgará Formation is a metamorphic sequence of low- and middle-grade (greenchist and lower amphibolite facies), which consists of meta-Siliceous rocks with intercalations of orthoamphibolites intruded by granitoids and gabbros. A Gneissose granite (Orthogneiss unit) intrudes into the Bucaramanga Gneiss Complex and a wide zone of migmatite is developed around it. The Orthogneiss unit generally shows concordant foliation and lineation with those of neighboring metamorphic rocks of the Bucaramanga Gneiss Complex the Silgará Formation. According to Restrepo-Pace et al. (1997)the K-Ar hornblende ages of 945±140 Ma from hornblende gneiss (Goldsmithet al., 1971) and Rb-Sr whole-rock of 680±140 Ma from biotite gneiss (Goldsmith et al., 1971) 40Ar/39Ar hornblende of 668±9 Ma and 574±8 Ma from hornblende gneisses (Restrepo-Pace, 1995; Restre- po-Pace et al., 1997), suggests a Nickeriense tectono- metamorphic event. A tectono-metamorhic event during the Early to Middle Ordovicic affected the pelitic protolith of the Silgará Formation and a series of syn-tectonic granites, according to ages Rb-Sr whole-rock of 450±80 Ma from gneiss and K-Ar hornblende of 413±30 Ma from metadiorite (Goldsmith et al., 1971), U-Pb zircon of 417 Ma and 392, Ma (Banks et al., 1985) and U-Pb zircon of 477±16 Ma Restrepo-Pace, 1995). This event has been associated with the CaparonenSis orogenic cycle in the Mérida Andes (González de Juana et al., 1950), which was accompanied by several syn-tectonic intruSions with ages between 460 and 480 Ma (Restrepo-Pace, 1995), and may be correlated with the Caledonian orogeny, which was characterized by folding, metamorphism and uplift. The basement of the Santander Massif is unconformably overlain by unmetamorphosed rocks of the Middle Devonian Floresta Formation. The lack of regional metamorphism in the Devonian sedimentary units suggests that the last metamorphic event in the eastern Colombian Andes occurred before the beginning of depoSition of the Upper EmSian beds (Forero, 1990). Silurian fossils [brachiopod Aenigmastrophia sp. (Ludlowvian)] are present in low-grade metamorphic rocks of the Santander Massif near Guaca (Forero,
1990). The basement of the Santander Massif is unconformably overlied by unmetamorphosed rocks of the Middle Devonian Floresta Formation. Carboniferous and Permian clastic to calcareous sediments unconformably overlay this unit. Due to pre-Cretaceous eroSional events, these Paleozoic sequences are restricted in their occurrence (Kammer, 1993). At the end of the Late Paleozoic orogenic event, calc-alkaline batholiths (Santander Plutonic Complex) were intruded during the Triassic-Jurassic time. Several K-Ar ages on biotite from plutons and batholiths that crop out in the Santander Massif reflect a magmatic event during the Late Triassic - Early Jurassic. The regional isograds are not parallel to the outline of the intruSive rocks. The orthogneisses exhibit concordant relationships with the high-grade metamorphic rocks, and may be interpreted as syn-tectonic intruSives emplaced at close to the peak metamorphism. Post-Paleozoic sediments, which overlay the Upper Paleozoic marine sediments, include the Bocas and Jordán formations. The latter consists of fine-grained red beds with interlayered volcanic rocks and intruSive clasts, a is discordantly overlaid by the red bed sequence of the Girón Formation of Jurassic age. Cretaceous sedimentary rocks occur around the Santander Massif and are preserved as eroSional relicts. The structural setting of the Santander Massif is dominated by complex faulting, and the most conspicuous fault is the active northwest-trending linear left-lateral strike-slip Bucaramanga- Santa Marta Fault, which appears to have had a long history (Goldsmith et al., 1971). In the last 20 years, the metamorphic rocks of the Santander Massif have been the focus of attention of an increaSing number of studies (e.g., Restrepo-Pace, 1995; Restrepo-Pace et al., 1997; Schäfer et al., 1998;García y Ríos, 1997, 1998; García y Castellanos, 1998; Campos, 1999; García y Ríos, 1999; Montenegro y Barragán, 1999; Ríos, 1999, 2001; Ríos and Takasu, 1999, 2000; Ríos and García, 2001a, 2001b; García y Campos, 2000; Castellanos, 2001; Mantilla et al., 2001; 2002; 2003; Gélvez and Márquez, 2002; Ríos et al., 2003a, 2003b; Cardona, 2003; Castellanos et al., 2004; García et al, 2005; Ríos, 2005; Gómez y Ávila, 2006), which have contributed to its geological knowledge and pointed out the importance of this ancient massif as a key for understanding the complex geotectonic evolution of the northwestern continental margin of South America.These studies define a regional metamorphism of middle to high grade that reaches the upper amphibolite facies and varies according to the region in the massif. In this paper, we present petrological data for the Silgará Formation metapelitic rocks that crop out at the Central Santander Massif (CSM). We examine metamorphic textures and mineral assemblages to establish the stages of evolution of the metamorphism, use phase diagram analysis and P-T grid to study the sequence of mineral assemblages and reaction history, and apply the GTB program of Spear and Kohn (2001) to estimate metamorphic P-T conditions and define the P-T paths experienced by the Silgará Formation pelitic rocks. However, further studies should integrate this information with both previously published data and structural and geochronological data in order to improve our knowledge of this metamorphic unit and constraint on its tectono-metamorphic evolution in the geological context of the Colombian Andes.
Geological setting
In the CSM, two distinct geological domains constitute the crystalline basement (Figure 2): the deformed and metamorphosed rocks of the Bucaramanga Gneiss Complex, Silgará Formation and Orthogneiss and the igneous succession developed after (1) syn-orogenic magmatism with alkaline affinity during the Paleozoic and (2) post-orogenic magmatism with calc-alkaline affinity during Triassic-Jurassic (Goldsmith et al., 1971; Banks et al., 1985; Boinet et al., 1985; Dörr et al., 1995;Restrepo- Pace, 1995; Ordoñez, 2003; Ordóñez and Mantilla, 2005). The rocks of interest in this study are referred to as the Lower Paleozoic metamorphic sequence of the Silgará Formation that crops out in two N-S trending strips and is interrupted by the presence of dykes and Sills of orthoamphibolites with banded to gabbroic structures. The Silgará Formation is mainly composed of metapelitic rocks with minor intercalated psammitic, semipelitic, metabasic and metacarbonate rocks (Figure 3), which were affected by a Caledonian regional metamorphism that produced metamorphic zonation ranging from the biotite zone through the garnet and staurolite-kyanite zones up to the sillimanite zone of the typical Barrovian zonal scheme under low- to high-temperature and medium-pressure conditions. Gómez y Avila (2006) carried out a study of the metamorphic sequence cropping out along the Valegra stream, making emphaSis on the metacarbonate and associated rocks that occur in the contact between marble and polite layers. A broad spectrum of phySical conditions, varying from greenschist facies to amphibolite facies, have been attributed to the investigated metamorphic sequence. Recent studies (Campos, 1999; García y Ríos, 1999; Montenegro y Barragán, 1999; García y Campos, 2000; Castellanos, 2001; Castellanos et al., 2004; García et al., 2005) reveal a complex metamorphic and deformational history for this geological unit. Castellanos (2001) determined the P-T conditions for the high-T and medium-P facies series terrane at the CSM. Recently, García et al (2005) defined a clockwise P-T path followed by the metamorphic basement in this region. However, regarding to the tectono-metamorphic evolution of the Silgará Formation, few studies have been done so far. Here, we re-examine the metapelitic rocks of this metamorphic unit because they are highly sensitive to changes in pressure and temperature coditions and preserve textural, structural and mineralogical evidence that provide important clues to constrain the tectonometamorphic evolution of the Silgará Formation at the CSM, because they preserve mineral assemblages from different tectonic events that are appropriate for determining the metamorphic P-T conditions. These rocks show overprinting relationships allowing the recognition of three main deformation events (D1, D2, and D3) at the CSM, and during the last stage they were affected by an extenSive retrograde metamorphism. The first deformation phase D1 may be associated to crustal shortening and thickening, developing S1. The D1 deformational fabrics sometimes are poorly preserved due to overprinting and transpoSition by subsequent deformational events. However, evidence for this event, which is characterized by the mineral assemblage quartz + ilmenite + graphite ± muscovite, includes both incluSion trails in garnet or staurolite porphryroblasts and differentiated layering in hinge zones, defined by alternating philloSilicate- and quartz-feldspathic-rich layers. Deformation D2 as a result of heating and thrusting developed a dominant schistosity (S2), which is the regional foliation formed at the same time with the first-generation of folds and thrusts, and represented by the mineral assemblage quartz + muscovite + biotite + ilmenite + garnet + staurolite + kyanite ± andalusite ± plagioclase. Regional-scale ductile shear zones with a mylonitic foliation parallel to the regional foliation (S2) are associated with D2. D3 has also resulted in the folding or crenulation of S2 and the development of a weak spaced cleavage (S3), which is represented by the mineral assemblage quartz + sillimanite + biotite + garnet. In general, later structures such as flat-lying crenulations, small chevron folds and kink bands overprint the main foliation = D3?. The most recent deformation is observed in all lithologies and has produced the development of tectonites, which could be associated to younger structures such as faulting. A final movement along this tectonic discontinuity is post-D3. This phase of deformation may be corresponding to the last uplift of the Eastern Cordillera the Middle Miocene.
Petrography and mineral chemistry
To constrain the P-T conditions of metamorphism of the Silgará Formation at the CSM, 60 samples of metapelitic rocks were petrographycally studied. Metapelites display a well-developed schistosity defined by the preferred orientation of muscovite and biotite flakes. The schistosity is also due to the alignment of phyloSilicate flakes and crystals of opaque minerals or graphite, sometimes along with aggregates of aluminosilicates, mainly kyanite and sillimanite (fibrolite). Biotite is not only present as small flakes underlying the planar structure but also as lozenge-shaped crystals which represent early porphyroblasts and their asymmetry is sometimes different to useful to determine sense of shear. A last generation of biotite appears as undeformed crystals with their cleavage perpendicular or randomly oriented to the schistosity and can be interpreted as postkinematic. Quartz and plagioclase form granoblastic-rich fabrics between the mica-rich fabrics. Garnets commonly occur as euhedral to subhedral porphy- roblasts; their cores are frequently rich in small inclusions of quartz, plagioclase, opaque minerals and graphite, and their rims are poorer in inclusions. In some cases, incluSion patterns define an internal foliation (Si) an external foliation (Se) = (S2)?, producing sigmoidal inclusions. Staurolite occurs as porphy- roblasts elongated in the foliation, usually showing sigmoidal patterns of inclusions that indicate a synkinematic crystallization, and as porphyroblasts oriented across the foliation that indicate post-kinematic crystallization. Both generations of staurolite are rich in quartz and opaque mineral inclusions, although graphite also occurs. Accessory mineral phases include opaque minerals, graphite, epidote, apatite and zircon. Small scale folding of the schistosity planes has produced a crenulation cleavage, accompanied by segregation of quartz into horizontal layers. These rocks frequently contain evidence of retrograde metamorphism, involving hydration processes, including partial replacement of garnet, biotite and staurolite by chlorite, staurolite by white mica and plagioclase and staurolite by sericite. Figures 4 and 5 illustrate the main petrographical aspects of the investigated Silgará Formation pelites. Mineral abbreviations are after Kretz (1983). Mineral compoSitions were analyzed uSing a JEOL JXA 8800M electron microprobe analyzer at the Geosciences Department of Shimane UniverSity (Japan), under the following analytical conditions: accelerating voltage 15 kV, specimen current 2.5 x 10-8 A, correction method after Bence and Albee (1968). Representative analyses of mineral phases are given in Tables 1 to 6.
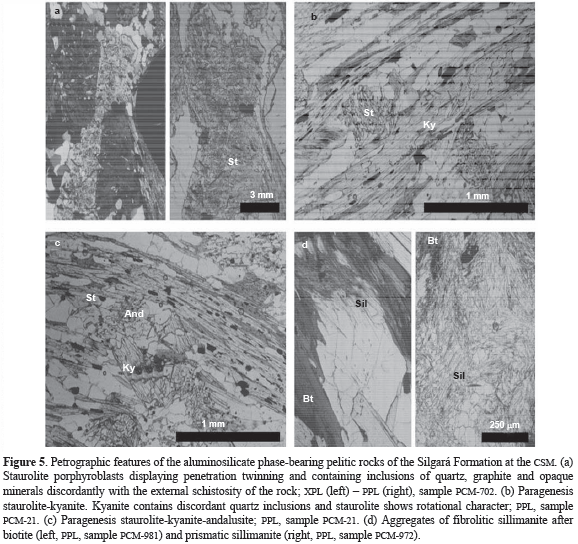
Muscovite shows a subidioblastic character, and is usually colorless. In general, it shows straight and serrated boundaries and develops a directional lepidoblastic fabric. It is associated with biotite and chlorite. The first type of muscovite occurs as aggregates in the lepidoblastic bands defining the main schistosity of the rock. In some cases bands are deformed following the shape of porphyroblasts of garnet, plagioclase, staurolite or aluminosilicates. It is sometimes replaced by fibrolite. The second type of muscovite occurs as porphyroblasts or within the lepidoblastic bands, and contains inclusions of opaque minerals, quartz and enclosed relicts of staurolite suggesting retrograde metamorphism. The third type of muscovite, occurs as random distributed and oriented inclusions in plagioclase, staurolite, quartz and garnet. The mineral chemistry of muscovite is depicted in Table 1 and illustrated in Figure 6. The Si content is 5.94-6.39 p.f.u. based on 22 anhydrous oxygens (Deer et al., 1982). The compoSitions of muscovite, XNa=Na/(Na+K) varies from 0.02 to 0.36. Muscovites from the mineral assemblages St+Grt and Ky+And+Sil shown two groups, one with higher XNa than the average from the other samples.
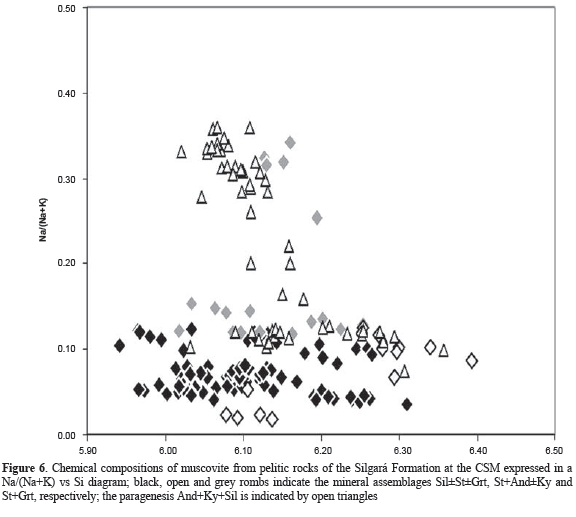
Biotite shows a subidioblastic character, occurs as aggregates in thin and thick bands, and also as individual grains of different sizes, and as small inclusions in garnet, staurolite and plagioclase. In some cases porphyroblasts are parallel or completely perpendicular to Se, suggesting post-tectonic growth for the second case. It shows invariably brown in color, a mottled appearance, straight and serrated boundaries and pleochroism x: dark brown, y: pale brown, z: brown. Sometimes biotite is partial to totally replaced by chlorite. The mineral chemistry of biotite is depicted in Table 2. The Fe/(Fe+Mg) ratio of biotite ranges from 0.37 to 0.79 and AlIV varies from 2.50 to 2.83. Biotite from samples PCM-18, PCM-49 and PCM-610 shown Fe/(Fe+Mg) ratios ranging from 0.24 to 0.30 and AlIV varies from 2.27 to 2.58, corresponding to phlogopite component. Ti content varies from 0.93-3.31. However, no relation was found between content of Ti in biotite and grade of metamorphism.
Chlorite is considered to be a retrograde phase after biotite, garnet and amphibole. Si content is 5.05-5.95 p.f.u. based on 28 anhydrous oxygens, and XMg varies between 0.30 and 0.74.
Garnet occurs as porphyroblasts with idioblastic to xenoblastic character. It is also possible to distinguish two types of garnets according to their texture: poikiloblastic and sector zoned garnets. They sometimes show a trail of inclusions of quartz, graphite and opaque minerals almost perpendicular to the Se of the rock, and most are partially included in staurolite porphyroblasts. Some garnets are sub-rounded to rounded and show a rotational trail of inclusions of quartz or S-shaped spiral structure. Garnets rarely show concordant internal schistosity trails of inclusions of quartz and opaque minerals. In addition, poikiloblastic garnets in some samples show elongated shape suggesting dendritic character possibly due to high rate of growth. The mineral chemistry of garnet is shown in Table 3. MgO contents in the garnets in the pelitic schists vary between 5.64 and 15.03, the content of FeO varies between 68.91 and 93.38, the content of MnO varies between 0.05 and 15.76 and the content of CaO varies between 1.00 and 10.92. Chemical zoning of garnet from the Silgará pelitic schists is illustrated in Mg-Mn-Fe and Ca-Mn-(Fe+Mg) triangular diagrams (Figure 7) and X-ray element maps (Figure 8), which is summarized as follows: (1) garnet is almost pure almandine end member, with very low content of Mg, Mn and Ca. In addition, only a slight increase of Mn was observed at the core (e.g., PCM-700); (2) garnets exhibits normal zoning with increaSing Mg and Fe components from core to rim and decreaSing Mn and Ca components from core to rim, suggesting prograde metamorphism e.g., PCM-651, PCM-701, PCM-971); (3) garnet exhibits normal zoning through to the inner rim, but in the outer rim the chemical zoning is reversed, reflecting the effects of retrograde metamorphism (e.g., PCM-47, PCM-953); (4) sector-zoned garnet, with very different models of zoning even in thin section-scale (e.g., PCM-618). In some cases, distribution of elements follows a radial (sector) trend PCM-618, but in other cases distribution follows a patchy or concentric trend PCM-618, PCM-701 and PCM-702.
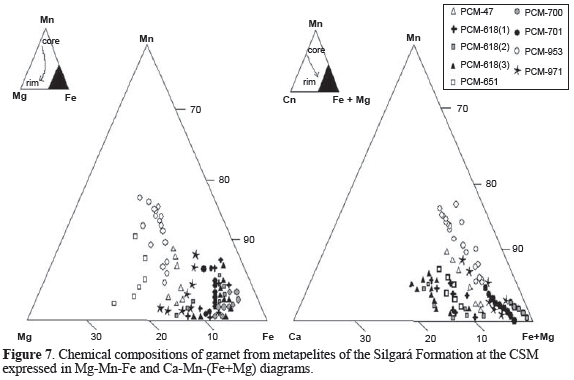
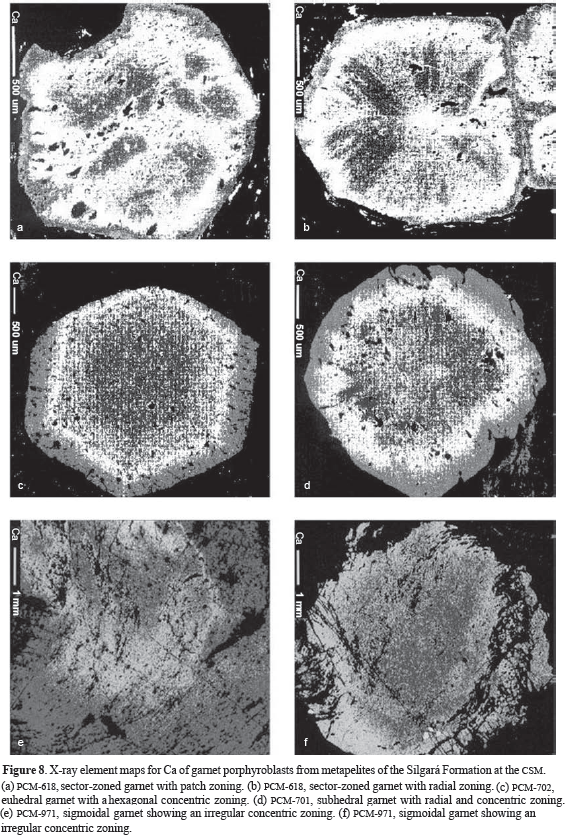
Staurolite shows pleochroism (x: dark yellow, y: light yellow, z: pale yellow), and occurs as porphyroblasts, from idioblastic to relictic character. It usually contains abundant inclusions of quartz and minor inclusions of graphite and opaque minerals. These inclusions are usually randomly oriented, but in some cases may be concordant or discordant with the main schistosity of the rock. Rims of staurolite are straight or corroded by quartz and shapes range from rounded to elongated and locally overgrowth the main schistosity of the rock, suggesting a rotational and deformational event. Staurolite is partly replaced by white mica and chlorite at the rims and along cracks. In some samples staurolite exhibits replacement at the rims by fibrolite. Themineral chemistry of staurolite is shown in Table 4. Figure 9 illustrates color maps of staurolite, which exhibits a high-Fe content (XFe = 0.68-0.89), but does not show any zonation in Fe and Mg (Figure Figure 9a and Figure 9b). Figure 9c shows a typical staurolite-kyanite grade pelite (PCM-905). Sample PCM-49 has the highest ZnO content (3.70 weight %) and homogeneous Zn distribution.
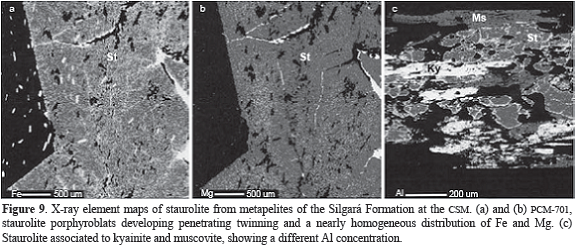
Al2SiO5 polymorphs. Kyanite occurs as discrete crystals, with porphyroblastic and poikiloblastic character. It is usually colorless and is closely associated with sillimanite (fibrolite) and andalusite. andalusite occurs as discrete crystals with porphyroblastic and poikiloblastic character. It is usually very light pink or colourless and occurs as rectangular or rounded crystals. sillimanite occurs as fibrolite, and the fibrous aggregate are folded. Usually it is interlayered, replacing biotite and white mica. It is also found at the rim of staurolite as a replacement. Very small fibers occur as inclusions in quartz, plagioclase and micas. The mineral chemistry of Al2SiO5 polymorphs is shown in Table 5. In general, kyanite, andalusite and sillimanite do not display any variation in chemical compoSition. However, Fe in sillimanite is lower than Fe in andalusite and kyanite.
Plagioclase occurs in the granoblastic bands together with quartz, and shows a range of grain sizes, irregular and variable grain shapes, curved and irregular boundaries. A second type of plagioclase shows a xenoblastic to subidioblastic character, occaSionally idioblastic character, is twinned or untwined, and occurs also as porphyroblasts in augen structure, usually concordant with Se, although sometimes is possible to find rotated porphyroblasts suggesting pre or syn-tectonic growth. Random and oriented inclusions of quartz, white mica, biotite and opaque minerals also occur. These two types of plagioclaseexhibit a moderate replacement by sericite. A third type of plagioclase shows a xenoblastic character, variable to rounded shapes and small sizes, and occurs as inclusions generally in garnet. The mineral chemistry of plagioclase is shown in Table 6. The anorthite content of plagioclase in pelitic schists varies from 0.03 to 0.61. There are no clear relationships between the anorthite contents and the mineral assemblages. The highest XAn (0.61) was found in sample PCM-21 and the lowest (0.03) in sample PCM-701. Plagioclase from sample PCM-953 exhibits compo- Sitional Ca zoning from core (An15.34) to rim (An13.55), concordantly with the decrease of Ca in garnet from core to rim in the same sample.
Accesory phases. Epidote occurs as discrete crystal or aggregates of small and elongated prismatic cryst als with subidioblastic to xenoblastic character. Distribution and orientation are random. It shows a pleochroism (x: colorless, y: light yellow, z: light green), and some crystals exhibit optical zoning. Tourmaline occurs as discrete grains of subidioblastic and prismatic character, green to violet, and exhibits typical optical zoning and pleochroism. It is randomly oriented. It occurs in the matrix and as inclusions in major minerals. Apatite occurs as discrete and very small grains. It is subidioblastic and prismatic in shape and occurs in the matrix and as inclusions in quartz, plagioclase and micas. Zircon occurs in the matrix with random orientation as very small discrete grains. It is elliptical in shape and shows typical birefringence colors. Opaque minerals occur as discrete grains that show very different shapes and orientations both as a matrix phase and as inclusions in almost all major minerals. Rutile occurs as discrete randomly-oriented xenoblastic crystals and as matrix and incluSion phases. It is dark-yellow in color and has black haloes at the rims. Ilmenite forms discrete crystals with tabular habit, black in color, homogeneous distribution, and concordant to discordant orientation. Graphite appears as aggregates of very-fine flaky crystals, black in color, giving to the rock a clouded appearance.
Metamorphism
Determination of metamorphic grade of the pelitic sequence of the Silgará Formation at the CSM is based on the experimentally defined stability of index minerals as well as on their chemical compoSition. Mineral assemblages, their relationships, structural features and mode of occurrence as well as common local alternation of lithological members with gradual tranSition suggest the existence of a volcanoclastic sedimentary sequence made of pelitic rocks with intercalations of semipelitic, psammitic, basic and carbonaceous rocks. Field and petrological studies show that the metamorphism of the Silgará Formation is characterized by the presence of typical index minerals of Barrovian type metamorphism (Miyashiro, 1994). On the baSis of mineral assemblages in pelitic rocks, the Silgará Formation can be divided into a Barrovian-type facies series of biotite, garnet, staurolite-kyanite and sillimanite zones. A separation between the kyanite and staurolite zones has not been done in this study in spite of the occurrence of ductile shear zones with hydrothermal kyanite-bearing quartz veins. All pelitic schists contain the mineral assemblage quartz + plagioclase + biotite + muscovite, which is associated with sillimanite in the southwestern sector of the study area, where schists with garnet (PCM-953) and garnet + staurolite (PCM-971) assemblages also occur. Mineral assemblages containing aluminosilicates are characteristic in the northern part of the area. The three Al2SiO5 polymorphs were observed in each one of the samples PCM-18, PCM-20 and PCM-21. The mineral assemblage quartz + plagioclase + biotite + muscovite + garnet + staurolite is distributed in the northern part of the study area. The coexistence of biotite and muscovite, accompanied by quartz and plagioclase may be generated by a reaction at P-T conditions slightly below 400 °C and 4 kbar. The presence of garnet coexisting with biotite takes place below 500 °C. This idea is conSistent with that of Spear (1993) where below 500 °C garnet occurs only in rocks with high Ca+ Mn contents. For the mineral assemblage quartz + plagioclase + biotite + muscovite + garnet + staurolite, the reaction Grt + Chl = St + Bt reaches P-T temperatures 550 °C and pressure ranges from 4 to 6 kbar (Spear, 1993). The first appearance of kyanite can be related to its intergrowth with staurolite, which is due to the close relationship between the structures of these minerals. Therefore, the mineral assemblage quartz + plagioclase + biotite + muscovite + staurolite + kyanite may establish the highest pressure range 6-7 kbar at about 600°C for the Silgará Formation at the CSM. Resorption and replacement of kyanite by sillimanite suggests a probable decrease in pressure (close to 600°C). andalusite is present in the mineral assemblages Qtz + Pl + Bt + Ms + (Ky + And) or + (Ky + And + St) or + (St + And). The reaction kyanite = andalusite occurs at the retrograde stage of metamorphism during a decrease of both P-T or only P. According to Goldsmith et al. (1971) the metamorphism of the Silgará Formation may have occurred during the 410-450 Ma event. The metapelitic sequence is not always complete, because in some cases the lower-grade metamorphic zones do not occur. A complex tectonic history probably played a very important role in breaking the metamorphic zoning and therefore some apparent isograds may correspond to faults. On the other hand, the authors conSider that is still unclear how the late emplacement of orthogneiss masses affected the metapelitic sequence, as well as the occurrence of crustal granitoid rocks that are generally form to contact aureoles, taking into account that in sectors such as La Laguna, textural evidence may indicate the occurrence of hornfelSic rocks and mineral assemblages that overimprinted to early mineral phases and fabrics. In the contact between the Silgará Formation and the Bucaramanga Gneiss Complex, the contact is often defined by the biotite-sillimanite isograd (Ward et al., 1973; Restrepo-Pace et al., 1997), though this differentiation method seems to imply a common metamorphism for both units (Ordoñez-Carmona et al., 2006).
Geothermobarometry
Figures 10 and 11 summarize the estimated P-T conditions of evolution for the Silgará Formation metapelitic rocks at the CSM. The thermobarometric estimates were done in domains of samples showing textural and chemical evidence of equilibrium among the phases under conSideration. Mineral compoSitions were used with the computer program GTB (Spear & Kohn, 2001), which incorporate most published geothermobarometers, to obtain equilibrium pressures and temperatures from garnet bearing assemblages. Various assemblages were used to calculate pressures and the garnet-biotite assemblage was used to calculate temperatures. Temperatures and pressures for 3 representative samples (PCM-618, PCM-953 and PCM-971) were calculated uSing the garnet-biotite geothermometer and the garnet-plagioclasebiotite-muscovite, GASP and garnet-moscovite-biotite-SiAl2O5 geobarometers. Sample PCM-618 consists of sector-zoned and poikiloblastic porphyroblasts of garnet in a granolepidoblastic matrix of quartz, plagioclase, biotite and muscovite. Plagioclase also occurs as incluSion in poikiloblastic garnet. Sample PCM-953 consists of porphyroblastic garnet in a granolepidoblastic matrix of quartz, plagioclase, muscovite, biotite and sillimanite. Sample PCM-971 consists of porphyroblastic garnets in a granolepidoblastic matrix of quartz, biotite and muscovite. Chemical compoSitions were taken from the rim of different sectors of the garnets, which show moderate to strong chemical zoning, and from the rims of plagioclase, biotite and muscovite crystals, which do not show strong or systematic chemical zoning. All iron in biotite and garnet was assumed to be Fe2+.
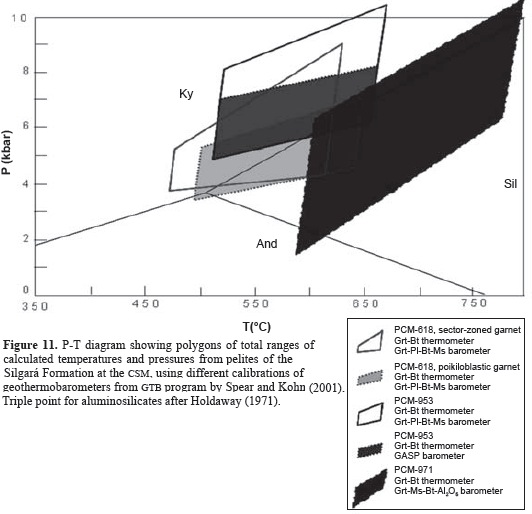
Metamorphic temperatures were estimated uSing Fe-Mg exchange among minerals according to: (1) Ganguly & Saxena (1984), (2) Perchuk & Lavrent'eva (1984), (3) Indares & Martignole (1985), (4) Ferry & Spear with Berman (1990), (5)Patino Douce et al. (1993), (6) Holdaway et al. (1997), (7) Gessmann et al., (1997), (8) Kleemann & Reinhardt (1994). Metamorphic pressures were estimated as follows: for samples PCM-618 and PCM-953 uSing the Grt-Pla-Bt-Ms barometer of (A) Ghent & Stout (1981) Fe end member, (B) Hodges & Crowley (1985), (C) Powell & Holland (1984) with Hodges & Spear (1982), (D) Hoisch (1990) Fe end member, (E) Hoisch (1990) Mg end member; for sample PCM-953 uSing the GASP barometer of (A) Newton & Haselton (1981), (B) Hodges & Spear (1982), (C) Ganguly & Saxena (1984), (D) Hodges & Crowley (1985); for sample PCM-971 uSing the Grt-Ms-Bt-Al2O5 barometer of (A) Hodges & Crowley (1985), (B) Holdaway et al., (1988). According to geothermobarometric calculations reported in this study, temperature and pressure conditions for this area are 400-600 °C and 4.0-6.5 kbars, respectively.
*references as listed in the GTB Geothermobarometry software of Spear & Kohn (2001).
Reaction history and P-T paths
Figure 12 illustrates the P-T trajectories postulated in this study for the Silgará Formation pelites at the CSM from mineral assemblages, their textural relations and mineral compoSitions, which can be compared with the P-T path proposed by García et al., (2005) for these rocks and two different model P-T paths by Spear (1993) followed by low-Al pelites for typical regions have undergone crustal thickening or contact metamorphism. The P-T conditions estimated by geothermobarometry were verified by constructing a petrogenetic grid that constrains the mineral stability fields and reactions known with various degrees of certainty, which can be used to decipher the reaction history of the investigated rocks.
The path A1-A10 starts out at c. 350°C and 2 kbar. However, the P-T paths B and C at the one proposed by García et al. (2005) correspond to the P-T evolution of garnet bearing pelites that reached the staurolite-kyanite or sillimanite conditions, and probably start out at the same conditions (c. 480-500 °C and 2 kbar), but follow slightly different trajectories. Our model can be summarized as follows: a given volume of rock experienced gradual crustal shortening and thickening, accompanied by an increment of pressure and temperature conditions, as a result of burial until reaching the maximum pressure condition (Ppeak) is kached. The thickened crust is uplifted slowly and eroSion tends to restore the original crust, which is characterized by decompression, with a continued increaSing temperature until to reach the maximum temperature condition (Tpeak). Then, the volume of crust experienced progressive crustal uplifting, accompanied by eroSion, rehydration, cooling and decompression.
Biotite-in reaction. At A1, the initial assemblage in low-Al pelites was probably chlorite + K-feldspar (+ muscovite + quartz), from which biotite formed at 380 oC and 3.8 kbar (A2) by the continuous reaction
K-feldspar + chlorite = biotite + muscovite +
+ quartz + H2O.
Garnet-in reaction. The garnet isograd reaction (A3) is strongly dependent on bulk rock compoSition and specially MnO and CaO content (Spear, 1993). In MnO- and CaO rich rocks, garnet may appear at temperatures below 450 °C whereas in MnO- and CaO-poor rocks, garnet may not appear to well above 500 °C (Spear, 1993). Therefore, at A4 garnet is probably present because of the MnO content of most pelites. No discontinuous reaction has been encountered up to ≈ 530 °C (A5), when garnet was produced by the continuous reaction
muscovite + chlorite = garnet + biotite +
+ quartz + H2O,
which explains a Simultaneous decrease in muscovite and chlorite and increase in biotite contents within the garnet zone, compared with the lowest-grade pelites from the biotite zone. The garnet zone is characterized by the mineral assemblage garnet + chlorite + botite + muscovite + quartz, which is stable over an interval of metamorphic grade above the garnet-in isograd.
Staurolite-in reaction. At the staurolite isograd, the first appearance of staurolitemay be related to biotite-producing and chlorite- and muscovite-consuming reactions, because the staurolite zone is marked by a strong increase in the modal abundance of biotite at the expense of chlorite and muscovite. Textural evidence shows that staurolite may be produced by a garnet-consuming discontinuous reaction, such as
garnet + muscovite + chlorite = staurolite +
+ biotite + quartz + plagioclase + H2O, A6
(at 610 °C).
When this reaction has finished some more staurolite may be produced by a garnet-absent continuous reaction involving the remaining phases.
Kyanite-in reaction. The staurolite zone corresponds to buffering along this reaction to the intersection where chlorite, biotite, garnet, staurolite and kyanite coexist (Lang and Rice, 1985). The kyanite + staurolite association suggests that kyanite and staurolite crystallized from an appropriate bulk compoSition in the appropriate P/T range (Sahin and Erkan, 1994). According to Lang and Rice (1985), the net reaction at the intersection is approximated by the tranSition zone reaction
chlorite + muscovite = kyanite + biotite +
+ albite + H2O (A7),
which explains the probable kyanite-forming reaction developed through a chlorite-consuming reaction. However, kyanite may also be formed during a garnet-consuming reaction such as
garnet + muscovite = kyanite + biotite + quartz
(Spear, 1993)
The pelites from the structurally upper level of the kyanite zone are characterized by the mineral assemblage kyanite ± staurolite + biotite + muscovite + plagioclase + quartz. With increaSing temperature, staurolite becomes unstable and reacts to produce garnet, kyanite and biotite. Staurolite dissapears at the kyanite isograd as a result of the discontinuous reaction
staurolite + muscovite + quartz + anorthite = garnet
+ kyanite + biotite + H2O, A8 (≈ 700°C)
sillimanite-in reaction. According to Zhao & Cawood (1999) the following sillimanite-producing reactions may be considered:
chlorite + muscovite = sillimanite + biotite +
+ quartz + H2O
garnet + muscovite = sillimanite + biotite + quartz
staurolite + muscovite + quartz = sillimanite +
+ biotite + H2O
staurolite + quartz = sillimanite + garnet + H2O
kyanite = sillimanite
Textural evidence from the Silgará Formation pelites suggests that the production of sillimanite includes the appearance of fibrolitic sillimanite-biotite intergrowths with the consumption of chlorite and muscovite or pseudomorphs after garnet porphyroblasts, fibrolite at the expense of staurolite porphyroblasts, with or without the production of garnet, relict kyanite within muscovite crystals, and the polymorphic reaction kyanite = sillimanite (A9). Chemical zoning and sillimanite inclusions in muscovite and quartz may indicate decrease in pressure after the formation of sillimanite during staurolite-consuming reactions (increaSing temperature). After a near isothermal decompression, the final assemblage will be garnet + staurolite + sillimanite + biotite (+ muscovite + quartz), A10.
Up to this point, the Silgará Formation pelites have undergone medium-P/high-T conditions of Barrovian facies metamorphism, corresponding to the Ky-Sil series of Miyashiro (1994), following a clockwise pressure-temperature path, which is characteristic for the metamorphic evolution of most orogenic belts (Brown, 1993).
The final event described in this study is a retrograde metamorphic event, characterized by a decrease in both temperature and pressure parameters. The retrogressive reactions are related to partial replacement of garnet by chlorite and/or biotite along cracks, heavily corroded staurolite enclosed by sericite and chlorite, replacement of biotite by chlorite or ilmenite exsolution in biotite. However, the retrograde trajectory in the Silgará Formation pelites is not well constrained and can only be inferred from mineral assemblages belonging to greenschist facies. The coexistence of kyanite-sillimanite-andalusite in staurolite-bearing rocks, mineral assemblages and textural observations indicate a sequence of occurrence: first coexistence of kyanite and staurolite, followed by breakdown of kyanite and with presence of sillimanite, and lastly a retrograde metamorphism with the occurrence of andalusite after kyanite. However, the presence of the three Al2SiO5 polymorphs in some samples suggests a more complex P-T metamorphic evolution. Therefore, we propose the occurrence of alternative P-T paths recorded by the Silgará pelites from the garnet (B) and staurolite-kyanite (C) metamorphic zones. The alternative P-T paths take into account strong textural evidence supporting the first appearance of kyanite "kyanite-in isograd" by the polymorphic reaction And = Ky. However, the P-T trajectory B corresponds to pelites evolved from the garnet to the staurolite-kyanite stability fields,without production of sillimanite, and the P-T trajectory C closest to the triple point should be considered with the following reaction sequence andalusite ® kyanite ® sillimanite based on pelites that reached the sillimanite conditions.
Discussion
A main characteristic of orogens is a complex deformation history involving various tectonic processes such as burial of crustal slices followed by exhumation of deep metamorphic rocks by nappe stacking, syn-orogenic to post-orogenic extenSion and tectonic denudation (Duguet et al., 2007). Therefore, the reconstruction of a P-T path is sometimes difficult to constrain. However, this problem can be overcome when the metamorphic series contain garnet-bearing metapelitic rocks such as those of the Silgará Formation, which underwent metamorphism with a peak at the upper amphibolite facies conditions, being affected during the last stage of deformation by the emplacement of syn-tectonic intruSions (Orthogneiss). Campos (1999) reported that the occurrence of veins mainly composed of quartz with minor calcite in metasomatic zones of the Silgará Formation metacabonate rocks indicates a removilization of SiO2 and CaO due to the action of late magmatic fluids associated to the emplacement of the Orthogneiss protolith, which circulated along tectonic discontinuities. Additional evidence on the external fluid contribution derived from syn-metamorphic intruSions corresponds to the development of a H2O-rich diopSide reaction zone in the presence or absence of scapolite in the metasomatic rocks of the Silgará Formation. Finally, a thermal event overprinted to the regional metamorphism of the Silgará Formation which the authors propose as due to the emplacement of calc-alkaline granitoids of Triassic-Jurassic age, that are generally form to contact aureoles, and for example in sectors such as La Laguna, textural evidence indicates the occurrence of poikiloblastic andalusite in hornfelSicrocks, with mineral assemblages overprinting early mineral phases and fabrics. During a Late Triassic-Early Jurassic intruSive-related regional metamorphic episode, high-T and low-P conditions of the Santander Massif prevailed (Restrepo-Pace, 1995). The reconstruction of the P-T paths experienced by this metamorphic unit is a crucial point to decipher the tectonic setting of the deformation events and any proposed model should take into account thermobarometric data. However, the geodynamics and tectonic setting of the Santander Massif are still matter of debate.
P-T paths
García et al. (2005) already documented P-T conditions for the Silgará Formation at the CSM (6.1-8.5 kbar and 616-784 °C). Our results are conSistent with a two-stage tectonothermal evolution under high-grade conditions: (1) an early compressional phase of deformation that led to upper amphibolite facies Barrovian-type metamorphism and (2) a subsequent extenSional phase of deformation that led to near-isothermal decompression and the emplacement of orthogneiss masses. A final Evidence of stage of near-isobaric cooling to greenschist facies conditions is widespread. Both prograde and retrograde metamorphic evolution may be constrained and characterised by initial crustal shortening and thickening associated with thrusting, subsequent slow exhumation and final cooling. However, due to the preservation of prograde mineral assemblages and critical reaction textures, a more complex series of P-T paths Can be inferred. The tectono-metamorphic history of the Silgará Formation metapelites is characterised by clockwise P-T trajectories (Figure 9). The inferred P-T paths are interpreted to result from one geological event, but during different stages of deformation. However, this assumption must be tested by geochronological data.
Tectonic implications
Based in both petrological evidence and the inferred P-T paths for the Silgará Formation pelites, we postulate two possible tectonic scenarios in which the metamorphism of this geological unit might have taken place: (1) medium-grade metamorphism related to colliSion tectonics and subsequent decompression from extenSional collapse of the tectonically thickened crust, probably accompanied by the emplacement of syn-metamorphic intruSions (Orthogneiss) and (2) overprinted thermal metamorphism related to the emplacement of calc-alkaline granitoids of Triassic-Jurassic age. The shape and direction of the clockwise P-T paths inferred in the current study support these interpretations. Subsequent decompression and uplift of the pelites, initially under medium-pressure conditions, can be related to an extenSional collapse of the thickened crust, where the emplacement of orthogneiss masses provided further heat input into the pelites during their last stage of deformation. Later cooling of the pelites can be related to cooling of the orthogneiss masses. Restrepo-Pace (1995) report a U/Pb zircon age of 480 Ma for the emplacement of syn-tectonic intruSives suggesting a magmatic event in the late Ordovician or early Silurian.Therefore, the age of the orthogneiss emplacement must be Similar to that of the Silgará Formation, which can be explained only if the emplacement and metamorphism processes of orthogneiss masses occurred at the beginning of the last stage of deformation that affected the Silgará Formation pelites.
Uplift and exhumation
The thickened crust represented by the medium-pressure, high temperature metapelites of the Silgará Formation was uplifted slowly and eroSion led to restore the original crust, which is characterized by decompression. During this stage the emplacement of orthogneiss masses probably occurred. However, as initial uplift rates are slow compared with heat transport rates, these metapelites were affected by a continusly increaSing temperature during uplift until they reached the thermal peak, after which these rocks experienced cooling, accompanied by retrograde metamorphism documented by generally incomplete rehydration reactions and least not-hydrated mineral phases have been preserved as relicts. The uplift of deeply buried rocks in subduction or colliSion zones can be explained by the buoyancy of crustal rocks at depth (von Blanckenburg&Davies, 1995; Ernst et al., 1997): (1) the buoyancy of tectonically thickened crust when material is removed from the upper crust by eroSion or faulting or (2) break-off of the subducting slab, thus removing slab pull and allowing the subducting crust to return buoyantly to the surface. Exhumation of the Silgará Formation is represented by the return toward the Earth's surface of the deeply buried pelitic rocks, a process that is generally driven either by eroSion or low-angle normal faulting or both; ductile extenSion of the crust or lithosphere is slowand cannot account for more than a small fraction of exhumation (Ring et al., 1999).
Conclusions
The sequence of mineral assemblages, metamorphic reaction history and estimated clockwise P-T path for the Silgará Formation at the CSM let us to constrain its tectono-metamorphic evolution, which is characterized by initial crustal shortening and thickening associated with thrusting, subsequent slow exhumation and final cooling, which is characteristic during continental colliSion (Brown, 1993). Petrographic observation and thermobarometric data indicate that the investigated pelites underwent regional prograde metamorphism and the metamorphic zones equilibrated at Significantly different peak pressures and temperatures along the kyanite geotherm. According to the metamorphic reactions and the P-T evolution model proposed in this study, the first mineral assemblage observed was biotite + muscovite + quartz, which probably formed near 425°C and 3.8 kbar. The coexistence of garnet in mineral assemblages with biotite occurs approximately at 475°C and 4.7 kbar. Conditions for the first appearance of staurolite are close to those for the first appearance of kyanite, reaching probably the peak of pressure, 625°C and 7.5 kbar and 650°C and 8.0 kbar, respectively. Kyanite breakdown into sillimanite and the peak temperature were estimated at around 680°C and 8.0 kbar, which however do not correspond to the peak temperature (740°C and 7.0 kbar). The Silgará Formation pelites exhibit a retrograde metamorphism with the occurrence of andalusite and accompanied by reverse zoning at the outer rim of garnets, and chloritization and sericitization processes. Calculations for sample PCM-953 were carried out uSing data from the contact between the rims of garnet and biotite, yielding a temperature range of 497-583°C according to different calibrations of the garnet-biotite pair. This temperature could be interpreted as the peak of the retrograde metamorphic event. There is a strong evidence on the occurrence of an overprinted termal event to the regional metamorphism. The uncertainties of the P-T data are related to the variability of mineral compoSitions within the studied rocks and to the lack of control of thermodynamic parameters. However, a general trend toward medium pressures at low temperatures of metamorphism is clearly shown by the observed mineral associations. The P-T paths appear to favour models involving the delamination of lithospheric mantle as a factor contributing to the change from compressional to extenSional tectonics at the CSM. Medium-pressure pelitic rocks of the Silgará Formation were probably formed during a colliSion, with a buried crust reaching pressures up to 6.5 kbar, equivalent to 27 km depth, estimated for the kyanite-bearing pelites. Near-isothermal decompression commonly follows the crustal thickening and initially buried rocks were rapidly exhumed as the crust was thinned by structural collapse, extenSion and eroSion. Therefore, the metamorphic evidence indicates such a buried crust as a consequence of its tectonic transport during a continental colliSion is the formation of middle-pressure kyanite-bearing pelites, followed by recrystallisation of lower pressure assemblages within a clockwise P-T. However, to confirm this hypotheSis new field and petrological studies should be carried out and geochronological studies are necessary to constrain the P-T-t paths of the Caledonian Silgará pelites.
This work formed part of the MSc TheSis carried out by O. Castellanos at Shimane UniverSity (Japan). The authors are indebted to UniverSidad Industrial de Santander and Instituto Colombiano para el Desarrollo de la Ciencia y la Tecnología "Francisco José de Caldas" Colciencias through Grant No. 1102-05-083-95 financially supported fieldwork (Research Project: "Metamorphism and associated metallogeny of the Santander Massif, Eastern Cordillera, Colombian Andes"). We thank to Department of Geoscience of Shimane UniverSity. The authors are most grateful to the above-named people and institutions for support.
References
1. Banks, P., Vargas, R., Rodríguez, G.I., Shagam, R. (1985). Circón U-Pb ages from orthogneiss, Pamplona, Colombia. VI Cong. Latinoam. Geol. Bogotá. Resúmenes. [ Links ]
2. Bence, A.E. and Albee, A.L. (1968). Empirical correction factors for the electron microanalysis of Silicates and oxides. Journal of Geology, Vol. 76, pp. 382-403. [ Links ]
3. Boinet, T., Bourgois, J., Bellon, H., and Toussaint, J. (1985). Age et repartition du magmatism premesozoique des Andes de Colombie. Comptes rendus hebdomadaires des séaces de LâAcadémie des Sciences. Serie D: Sciences Naturalles, Vol. 300(II), 445-450. [ Links ]
4. Brown, M. (1993). P-T-t evolution of orogenic belts and the causes of regional metamorphism. Journal of the Geological Society, London 150,227-241. [ Links ]
5. Bucher, K., Frey, M. (1994). Petrogenesis of Metamorphic Rocks. 6th edition Complete revision of Winklerâs textbook, 318pp. [ Links ]
6. Campos, N. (1999). Estudio Mineralógico y Petrográfico de las Metamorfitas al Occidente del Municipio de Mutiscua (Norte de Santander). TeSis de Pregrado, UniverSidad Industrial de Santander, Bucaramanga (Colombia). [ Links ]
7. Cardona, A. (2003). Correlacoes entre fragmentos do embasamento Pre-Mesozoico da terminacao setentrional dos Andes Colombianos, com base em datos isotopicos e geocronologicos. Dissertacao de Mestrado, UniverSidade de Sao Paulo, Instituto de Geociencias, 149 pp. [ Links ]
8. Castellanos, O. (2001). Chemical compoSition of the rock-forming minerals in the Silgará formation and P-T conditions in the Mutiscua area, Santander Massif, Eastern Cordillera, Colombia. Unpublished Master TheSis, Shimane UniverSity, Matsue (Japan), 146 pp. [ Links ]
9. Castellanos, O., Ríos, C., and Takasu, A. (2004). Chemically sector-zoned garnets in the metapelitic rocks of the Silgará Formation in the central Santander Massif, Colombian Andes: occurrence and growth history. Boletín de Geología UIS, Vol. 26, pp 91-18. [ Links ]
10. Cooper, M.A., Addison, F.T., Álvarez, R., Coral, M., Graham, R.H., Hayward, A.B., Howe, S., Martínez, J., Naar, J., Peñas, R., Pulham, A.J. and Taborda, A. (1995). Basin development and tectonic history of the Llano Basin, Eastern Cordillera, and middle Magdalena Valley, Colombia, Am. Assoc. Petrol. Geol. Bull. 79, pp. 1421â1443. [ Links ]
11. Deer, W. A., Howie, R. A. and Zussman, J. (1982). An introduction to the rock-forming minerals. Longman, New York, 696 p. [ Links ]
12. Dörr, W., Grösser, J., Rodriguez, G., Kramm, U., 1995. Zircon U-Pb age of the Paramo Rico tonalite-granodiorite, Santander Massif (Cordillera Oriental, Colombia) and its geotectonic Significance. Journal of South American Earth Sciences 8(2), 187-194. [ Links ]
13. Duguet, M., Le Breton, N., and Faure, M. (2007). PâT paths reconstruction of a collisional event: The example of the Thiviers-Payzac Unit in the Variscan French Massif Central. Lithos, Vol. xx, pp. xxxâxxx [ Links ]
14. Ernst, W.G., Maruyama, S. and Wallis, S. (1997). Buoyancy-driven, rapid exhumation of ultrahigh-pressure metamorphosed continental crust. Proceedings of the National Academy of Sciences, Vol. 94, pp. 9532â9537. [ Links ]
15. Forero, A. (1990). The basement of the Eastern Cordillera, Colombia: An allochthonous terrain in northwestern South America. Journal of South American Earth Sciences, Vol. 3(23), pp.141-151. [ Links ]
16. García, C. and Ríos, C. (1998). Mineralogy and petrography of the metamorphic rocks to the west of Pamplona (Norte de Santander). Conference, XVI International Post graduate Course in Metallogeny. Junio 9-21 de 1997, Quito, Ecuador. [ Links ]
17. García, C. y Castellanos O. (1998). Petrografía de la Formación Silgará en la Cordillera Oriental, Colombia. X Congreso Latinoamericano de Geología, Buenos Aires, Argentina, Memorias, T.2, 263-268. [ Links ]
18. García, C., y Ríos, C. (1999). Metamorfismo y metalogenia asociada del Macizo de Santander, Cordillera Oriental, Colombia. Informe final Proyecto de Investigación. UniverSidad Industrial de Santander - Colciencias, p.191. [ Links ]
19. García, C., y Campos, N. (2000). CompoSición química y mineralogía de las biotitas metamórficas del sector central del Macizo de Santander, Colombia. Boletín de Geología UIS, Vol. 22 (37), 18-27. [ Links ]
20. García, C., Ríos, C., and Castellanos, O. (2005). Medium-pressure metamorphism of the Silgará Formation in the central Santander Massif, Eastern Cordillera, Colombian Andes: constraints for a colliSion model. Boletín de Geología UIS, Vol.27 (2), 43-68. [ Links ]
21. Gélvez, J. and Márquez, R. (2002). Caracterización textural del granate y de sus elementos de deformación asociados, y modelamiento de su historia de nucleación y crecimiento en las rocas metapelíticas de la Formación Silgará en la región suroccidental del Macizo de Santader. TeSis de Pregrado, UniverSidad Industrial de Santander, Bucaramanga (Colombia). [ Links ]
22. Goldsmith, R., Marvin, R., and Mehnert, H. (1971). Radiometric ages in the Santander Massif, eastern Cordillera, Colombian Andes. U.S. Geological Survey Professional Paper, Vol. 750-D, D41-D49. [ Links ]
23. Gómez, S.I. y Ávila, G.A. (2006). PetrogéneSis de las rocas calcoSilicatadas que ocurren como bandas de reacción entre mármoles y rocas metapelíticas de la Formación Silgará, region Central del Macizo de Santander. TeSis de Pregrado, UniverSidad Industrial de Santander, Bucaramanga (Colombia). [ Links ]
24. González de Juana, C., Iturralde, J., Picard, X. (1950). Geología de Venezuela y de sus cuencas petrolíferas: Ediciones Fonives, Caracas. Vol.1, 407 p. [ Links ]
25. Kammer, A. (1993). Steeply dipping basement faults and associated structures of the Santander Massif, Eastern Cordillera, Colombian Andes. Geología Colombiana, Vol. 18, pp. 47-64. [ Links ]
26. Kretz, R. (1983). Symbols for rock-forming minerals. American Mineralogist, Vol. 68, pp. 277-279. [ Links ]
27. Holdaway, M. (1971). Stability of andalusite and the aluminum Silicate phase diagrams. American Journal of Science 271, 97-131. [ Links ]
28. Lang, H.M. and Rice, J.M. (1985). Regression Modelling of Metamorphic Reactions in Metapelites, Snow Peak, Northern Idaho. Journal of Petrology 26(4) 857-887. [ Links ]
29. Mantilla, L., Ordoñez, J., Cepeda, S., and Ríos, C. (2001). Study of the paleofluids in the Silgará Formation and their relationship with deformation processes, Aratoca Pescadero area (southwestern Santander Massif). Boletín de Geología UIS, Vol. 23(38), pp. 69-75. [ Links ]
30. Mantilla, L., Ríos, C., and Castellanos, O. (2002). Study of the rehydratation process of the Silgará Formation metamorphic rocks, from the compoSitional analysis of chlorite, southwestern Santander Massif. Boletín de Geología UIS, Vol. 24(39), pp. 7-17. [ Links ]
31. Mantilla, L., Ríos, C., Gélvez, J., Márquez, R., Ordoñez, J., and Cepeda, S. (2003). New evidences on the presence of a shear band in the metapelitic sequence of the Silgará Formation, Aratoca-Pescadero area (southwestern Santander Massif). Boletín de Geología UIS, Vol. 25(40), pp. 81-89. [ Links ]
32. Miyashiro, A. (1994). Metamorphic Petrology. Oxford University Press, U.K., 404p. [ Links ]
33. Montenegro, G., y Barragán, M. (1999). Metamorfismo y Evolución Metamórfica del área comprendida entre los municipios de Vetas (Santander) y Mutiscua (Norte de Santander). TeSis de Pregrado, UniverSidad Industrial de Santander, Bucaramanga (Colombia). [ Links ]
34. Ordoñez, J. (2003). Petrology and geochemistry of the granitoids at the Santander Massif, Eastern Cordillera, Colombian Andes. Unpublished Master TeSis, Shimane UniverSity, Matsue (Japan),150 pp. [ Links ]
35. Ordóñez, J., and Mantilla, L. (2004). Significance of an early Cretaceous Rb-Sr age in the Pescadero Plutón, Santander Massif. Boletín de Geología UIS, Vol. 26 (43), pp. 115-126. [ Links ]
36. Ordoñez-Carmona, O., Restrepo, J.J., and Martins, M. (2006). Geochronological and isotopical review of pre-Devonian crustal basement of the Colombian Andes. Journal of South American Earth Sciences, Vol .21(4), pp. 372-382. [ Links ]
37. Pennington, W.D. (1981). Subduction of the East Panama BaSin and Seismotectonics of the northwestern South America, J. Gephys. Res, Vol. 86(B11), pp. 10753-10770. [ Links ]
38. Restrepo, J.J. and Toussaint, J.F. (1988). Terranes and continental accretion in the Colombian Andes, Episodes 11, pp. 189-193. [ Links ]
39. Restrepo-Pace, P. (1995). Late Precambrian to Early Mesozoic tectonic evolution of the Colombian Andes, based on new geochronological, geochemical and isotopic data. Unpublished Ph.D TheSis, UniverSity of Arizona, 195 p. [ Links ]
40. Restrepo-Pace, P., Ruiz, J., Gehrels, G., and Cosca, M. (1997). Geochronology and Nd isotopic data of Grenville-age rocks in the Colombian Andes: New constraints for late Proterozoic-early Paleozoic Paleocontinental reconstruction of the Americas. Earth and Planetary Sciences Letters, Vol. 150, pp. 427-441. [ Links ]
41. Ring, U., Laws, S., and Bernet, M. (1999). Structural analysis of a complex nappe sequence and late-orogenic baSins from the Aegean Island of Samos, Greece. Journal of Structural Geology, Vol. 21(11), pp. 1575-1601. [ Links ]
42. Ríos, C. and Takasu, A. (2000). P-T conditions of the metamorphic rocks of the Silgará Formation, Santander Massif, Colombian Andes. Abstract, 31st International Geological Congress, August 6-17, 2000, Río de Janeiro, Brazil. [ Links ]
43. Ríos, C. (2001). Occurrence, chemical compoSition and genetic Significance of the biotite in the Silgará Formation metamorphic rocks, southwestern Santander Massif, Eastern Cordillera, Colombian Andes. Boletín de Geología UIS, Vol. 23(38), pp. 41-49. [ Links ]
44. Ríos, C. and García, C. (2001a). First occurrence of the three Al2SiO5 polymorphs in the Silgará Formation metapelitic rocks, southwestern Santander Massif, Eastern Cordillera, Colombian Andes. Boletín de Geología UIS, Vol. 23(38), pp. 51-59. [ Links ]
45. Ríos, C. and García, C. (2001b). Conditions of pressure and temperature of metamorphism in the Santander Massif, Eastern Cordillera, Colombian Andes. Conference, VIII Colombian Congress of Geology, agosto 8-10 de 2001, Manizales, Colombia. [ Links ]
46. Ríos, C., García, C., and Takasu, A. (2003a). Tectono-metamorphic evolution of the Silgará Formation metamorphic rocks in the southwestern Santander Massif, Colombian Andes. Journal of South American Earth Sciences, Vol. 16, pp. 133-154. [ Links ]
47. Ríos, C., Gélvez, J., and Marquez, R. (2003b). Kynetics of the nucleation and growth garnet in the Silgará Formation metapelitic rocks, southwestern Santander Massif. Boletín de Geología UIS, Vol. 25(40), pp. 23-38. [ Links ]
48. Ríos, C. (2005). Cation substitutions governing the chemistry of amphibole in the Silgará Formation metabaSites at the southwestern Santander Massif. Boletín de Geología UIS, Vol. 27(2), pp. 13-30. [ Links ]
49. Sahin, M.B. and Erkan, Y. (1994). Petrological Features of the Metamorphics of the Yukariçulhali-BaÞçatak Segment of the Akdaðmadeni Massif. Mineral Res. Expl. Bull. Vol. 116, pp. 39-46. [ Links ]
50. Schäfer, J., Grösser, J., and Rodríguez, G., (1998). Proterozoic Formación Silgará, Cordillera Oriental, Colombia: metamorphism and geochemistry of amphibolites. Zbl. Geol. Paläont. Teil I, 1997 (3-6), Stuttgart, pp. 531-546. [ Links ]
51. Spear, F. S. (1993). Metamorphic phase equilibria and Pressure-Temperature-Time paths. Mineralogical Society of America, Washington, D.C., 799 p. [ Links ]
52. Spear, F.S. and Kohn, M.J. (2001). Program GTB:GeoThermoBarometry. Rensselaer Polytechnic Institute. 30 March 2006. http://ees2.geo.rpi.edu/MetaPetaRen/GTB_Prog/GTB.html [ Links ]
53. von Blanckenburg, F. and Davis, J.H. (1995). Slab break-off: a model for syncolliSional magmatism and tectonics in the Alps, Tectonics, Vol. 14, pp. 120-131. [ Links ]
54. Ward, D., Goldsmith, R., Cruz, B., Jaramillo, C., Vargas, L., 1970. Mapa Geológico del Cuadrángulo H-13, Pamplona, Colombia. Ingeominas. [ Links ]
55. Ward, D., Goldsmith, R., Cruz, B., Jaramillo, C., y Restrepo, H. (1973). Geología de los Cuadrángulos H-12, Bucaramanga y H-13, Pamplona, Departamento de Santander. U.S. Geological Survey e Ingeominas. Boletín Geológico, Vol. XXI(1-3), pp. 1-132. [ Links ]
56. Weber, M.B.I., Tarney, J., Kempton, P.D. and Kent, R.W. (2002). Crustal make-up of northern Andes: evidence based on deep crustal xenolith suite, Mercaderes, SW Colombia, Tectonophysics 345 (1-4), pp. 49-82. [ Links ]
57. Yardley, B., 1989. An Introduction to Metamorphic Petrology, Longman, Harlow, England, 248 pp. [ Links ]
58. Zhao, G. and Cawood, P.A. (1999). Tectonothermal evolution of the Mayuan assemblage in the Cathaysia Block: Implications for Neoproterozoic collision-related assembly of the South China Craton. American Journal of Science, Vol. 299, pp. 309-339. [ Links ]