1. Introduction
The eastern margin of the Qinghai-Tibet Plateau (QTP) comprises two main geometrical blocks (Fig. 1). One is the triangular-shaped Songpan block, which is bounded by the Xianshuihe and Kunlunshan faults to the north and south, respectively, and sandwiched between the Qinghai-Tibet internal block and Sichuan Basin. The other is the rhombic-shaped Sichuan-Yunnan block, which has the Xianshuihe-Xiaojiang and Jinshajiang-Honghe fault systems as its eastern and western boundaries, respectively. The QTP's eastern margin is the focus of studies on eastward lateral extrusion of the former's crustal material (Tapponnier et al., 1982).
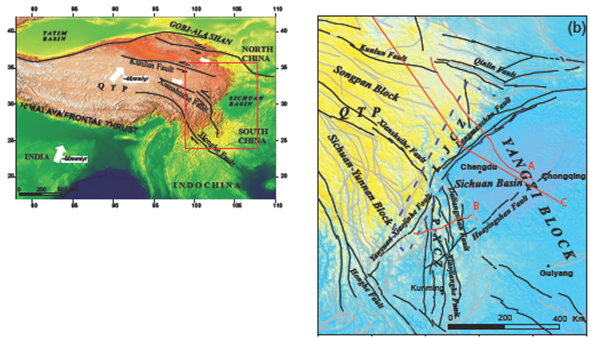
Figure 1 Schematic diagram of the location and geotectonic of the QTP's eastern margin. (a) Sketched tectonic map of the Qinghai-Tibet Plateau (QTP); (modified from Tapponnier et al., 2001); Red box is the location of the study area; (b) Tectonic and topographic map of the eastern margin of the Tibetan Plateau; Red lines A and B show LMT measuring lines; Line C indicates the cross-section of residual gravity; L-J C Z=Longmenshan-Jinpinshan construction zone, surrounded by the blue dashed box; P-X C Z=Panxi construction zone.
Over the years, researchers have produced tomographic imaging results for the QTP's crust-mantle structure (Wang, 2007; Wu et al., 2009; Wang et al., 2005; Zhang et al., 2012), as well as geophysical detection results on a semi-molten, low-velocity, and high-conductivity layer in the region's middle and lower crust (Sun et al., 2003; Xu et al., 2007; Zhao et al., 2008; Wang et al., 2009, 2013, 2014). With these results as the basis, Royden et al. (1997) proposed a lower-crustal channel flow model to explain the rapid uplift movements at the QTP's eastern margin. This model has been widely proven and recognized. The results of deep geophysical detection indicate that the crustal and upper mantle structures of the QTP's eastern margin are significantly different from that of the Sichuan Basin and South China block, which are located at its periphery. Depth gradients for the Mohorovicic (Moho) surface, crustal resistivity, and seismic wave velocity are greater in the border areas between the QTP and its surrounding blocks. The distribution of crustal material at the border areas is also inhomogeneous. This is especially the case for the Songpan block and interior of the Sichuan-Yunnan block, where there is an extensive distribution of a low-velocity, high-conductivity viscous layer within the lower crust in many regions. The physical structure of this layer is distinct from that of the brittle upper crustal layer. Located to the north of the Xianshuihe fault zone, the Longmenshan zone was impeded by Sichuan Basin's crust, which has greater rigidity. This resulted in middle-lower crustal accumulation, as well as rapid crustal thickening and significant uplift of the ground surface, leading to the formation of the soaring Longmen Mountains.
Clark and Royden (2000) proposed that the rigid Sichuan Basin had obstructed the migration channels for the plateau material, resulting in its diversion. This directional change for material flow has a significant relationship to the active tectonics at the QTP's eastern margin. At the region south of the Xianshuihe fault zone, crustal strength is relatively weak. Consequently, channels for material flow in the middle-lower crusts are wider (Clark et al., 2005).
The results of deep geophysical detection can provide substantial evidence for asthenospheric material flow within the lower crust. However, disagreements remain over the understanding of many fundamental theoretical issues relating to the QTP's eastern margin. A consensus has yet to be reached on matters such as structural properties of the Earth's crust at the QTP's eastern margin and the characteristics of its crustal movements, uplift mechanism of the eastern margin, and the characteristics of activities at the regional fault zones. Substantial amounts of observations at natural seismic stations and magnetotelluric (MT) probing of the deep structure were carried out at the QTP's eastern margin following the 2008 Wenchuan earthquake. Although the findings are remarkable and have been recognized, limitations remain when these are applied to research on the QTP's complicated deep structure and mechanism of dynamic uplift. This is because of the limited number of natural seismic stations, and the scattered bathymetric profiles did not adequately cover the vast expanse and complex geology and geomorphology of the QTP's eastern margin.
In this study, an analysis of the deep physical structure of the QTP's eastern margin was attempted. It was done via high-resolution apparent density imaging of Bouguer gravity anomalies within the study region, combined with long-period magnetotelluric (LMT) sounding results. The density and electrical parameters were then used for a comprehensive analysis of the profound structural differences and their geotectonic implications.
2. Tectonic background
QTP's eastern margin refers mainly to the region between the East Himalayan syntaxis and West Qinling orogenic belt (Fig. 1). Its northern side is connected to West Qinling, which is bounded by the East Kunlu fault system. The Longmenshan tectonic zone forms its eastern boundary, where it is adjacent to the Yangzi block. The Sichuan-Yunnan block constitutes the bulk of its southwestern section. Convergence of the Indian and Eurasian tectonic plates, which commenced at approximately 50 Ma, resulted in the closure of the Neo-Tethys Ocean and the QTP's rapid uplift.
These processes had profound impacts on the geological and geomorphological changes that occurred at the QTP's eastern margin. On the one hand, northward advancement of the East Himalayan syntaxis generated robust extrusion forces towards the east, forming nappe structures in an eastward direction. On the contrary, the QTP's rapid uplift and gravitational effect caused the material in the upper section of the lower crust to creep and spread towards the east, generating horizontal extrusion forces. Under the combined action of both forces, the Longmenshan-Jinpingshan nappe tectonic zone was eventually formed and positioned, resulting in an approximately 3,000 m difference in terrain elevation between the two sides. Separately, a large-scale arcuate strike-slip fault system was formed at the eastern margin. This indicates the presence of a principal horizontal stress field, which overcame the confines of the original tectonic system to form a new geotectonic unit. Specifically, this refers to the southeast-east divergence of the Songpan block, and the south-southeast lateral slipping of the Sichuan-Yunnan block.
The Honghe fault, which is the boundary between the Indochina and Sichuan-Yunnan blocks, underwent dextral strike-slip movements at approximately 13-15 Ma (Tapponnier et al., 1990). For the Xianshuihe fault, which divides the Songpan and Sichuan-Yunnan blocks, sinistral strike-slip movements occurred at approximately 15-20 Ma (Xu et al., 2006). The maximum horizontal sliding distance between the two faults was 100 km (Wang et al., 2000; Zhang, 2008). This critical mode of movement not only affected the eastern margin's geology and geomorphology but also had constraining effects over the occurrence of seismic activities.
3. Physical structure
3.1. Apparent density structure
Data on the apparent density structure were acquired from the studies by Xu 2006, Xu et al. 2007 and Yang et al., 2008. Using their findings as the basis, we developed a set of high-speed imaging techniques based on apparent density and multi-scale separation of gravity anomalies (Li et al. 2015). The main thought process was as follows: first, anomalies were separated by interpolating and splicing Bouguer gravity anomalies using various cutting radii to obtain the residual gravity anomalies for different dimensions. Second, the iterative downward extrapolation method proposed by Xu (2006) was used to obtain residual anomalies for the various dimensions, thereby improving data resolution; and finally, extrapolation depth was defined as the depth of the field source, and residual anomalies for that depth were subjected to apparent density inversion. The resultant apparent density has better capabilities regarding lateral resolution because unlike traditional density inversions, the proposed method carries out inversion based on gravity anomalies located nearer the field source.
The Bouguer gravity anomalies were separated with cutting radii at increments of 10 km, which gave the residual gravity anomalies for the various cutting dimensions. Iterative downward extrapolations were carried out using the latter to obtain the residual apparent density distribution for the QTP's eastern margin at depths of 10-100 km (Fig. 2). The middle-lower crusts of the Songpan and Sichuan-Yunnan blocks located at the QTP's eastern margin were found to contain a low-density material, which is distributed extensively along the western side of the Longmenshan-Jinpingshan tectonic zone. This material accumulated was has been circulated along a northeast orientation, reaching vertically upwards from the upper mantle to the lower crust and forming an open density gradient zone with the high-density Yangzi block in the eastern section.
After the low-density material of the northern Longmenshan transition zone had been obstructed by the cold, rigid, and high-density block in the east, it made a noticeable NNE directional change in the lower crust at 40 km depth, before extending northwards and crossing over the West Qinling tectonic structure. Mo et al. (2006), Yu et al. (2009) believed that a lot of Cenozoic volcanic rocks formed with material originating from the mantle were related to this low-density material.
For the Sichuan-Yunnan block, the low-density material spread out in an arc along the northeast-southwest orientation within the middle crust at 20 km depth. After being bounded by the Panxi tectonic structure at 50 km depth, it extended in a northeast-south-southwest direction at the western end and a southeast direction within the Daliangshan region at the eastern end. This could be due to a weakening of structural density in the middle-lower crusts of the Panxi tectonic structure. Within the middle-lower crusts at the eastern end, the low-density material crossed the weakened Panxi tectonic structure to reach the Daliangshan tectonic region. Zhao (2008) interpreted the MT data and postulated that the low-density, the high-conductivity material might be the channel flow of the middle-lower crusts.
3.2. Electrical resistivity structure
There were two issues for consideration in respect of MT survey: (i) the limited signal frequency band (1/320-2000 s) of data acquired with MT equipment (V8); and (ii) inadequate understanding of the electrical structure of the region's mantle. MT and LMT observations were separately made at each measuring point when acquiring electrical data to address these issues. Both sets of data were then merged, and the resultant data set was subjected to inversion (Wang et al., 2013, 2014). The outcome was the deep electrical structure for cross-sections A and B shown in Figure 1.
Cross-section A has a total length of approximately 620 km and straddles the Songpan block, the Longmenshan tectonic zone, and the Sichuan Basin. Electrical imaging (Fig. 3a) indicates that the Songpan block's lithosphere has a lower resistivity than that of the overall structure. Vertical segregation of the lithosphere is as follows: high-resistivity upper crust; low-resistivity middle-lower crusts; top of the upper mantle, which has weak characteristics of high resistivity; and bottom boundary of the lithosphere at 130 km depth, where resistivity becomes low again. This resulted in an alternating high-low-high-low layout.
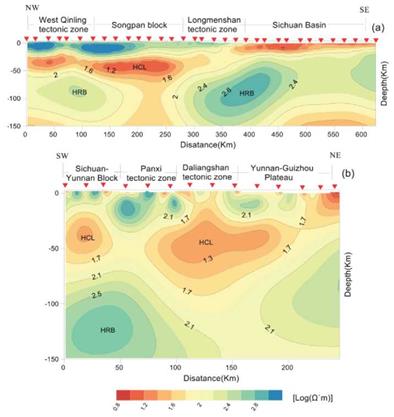
Figure 3 Resistivity model (a) and (b) derived by two-dimensional inversion of the merged MT-LMT data. HCL: high-conductivity layer; HRB: a high-resistivity body; Red Triangle: Measuring station.
Lithospheric resistivity is significantly high at the Yangzi block (Sichuan Basin). Vertical segregation of the lithosphere, which is thin in the east and thick in the west, is as follows: low resistivity for the middle-upper crusts, high resistivity for the middle-lower crusts, steadily high resistivity before reaching the bottom boundary of the lithosphere, low resistivity again at the lithosphere's bottom edge.
The Longmenshan tectonic zone is sandwiched between the Yangzi and Songpan blocks. The middle-lower crusts of its transition zone has the characteristic of prominently high resistivity that extends downwards into the lithosphere's upper mantle.
Cross-section B, with a total length of approximately 240 km, straddles three tectonic zones: Jinpingshan, Panxi, and Daliangshan. The middle-lower crustal structure at the eastern and western sides of the Panxi tectonic zone have significantly high conductivity, with its deep structure showing a trend of linkage to the Panxi tectonic structure. The resistivity of the middle-upper crusts is prominently high. For the Daliangshan tectonic zone, the lithosphere's upper mantle exhibits the weak characteristic of high conductivity. In contrast, the lithosphere of the Panxi tectonic zone has relatively high resistivity.
4. Discussion on tectonic implications
4.1. Discussion on uplift mechanism
There are two major views regarding the mechanism for Cenozoic uplift at the QTP's eastern margin. One is the plate extrusion model, which postulates that the crustal uplift process was caused by deformation of brittle material within the crust that arose from the thrust nappe effect (Tapponnier et al., 1986, 2001). However, the model's assumptions are not supported by the absence of a Quaternary foreland basin in front of the Longmenshan fault zone (Burchfiel et al., 1995; Jia et al., 2006), as well as a crustal shortening rate that is less than 3 mm/a (Chen et al., 2000).
The other is the lower-crustal channel flow model, which proposes the existence of a low-viscosity asthenosphere in the lower crust of the QTP's eastern margin. The asthenosphere slipped eastwards under the effect of plate movements. When it was obstructed by the Sichuan Basin, the low-velocity, high-conductivity lower-crustal material accumulated in front of the rigid Yangzi structure and was uplifted. This implies that the crustal uplift process was mainly caused by the accumulation of the middle-lower crustal material flow within the QTP's interior (Royden et al., 1997; Clark et al., 2000).
Distribution characteristics of physical properties show the existence of a low-density, high-conductivity material that is extensively distributed within the middle-lower crusts of the QTP's eastern margin. Seismic data analysis revealed that this material has a relatively high Poisson's ratio of > 0.25 (Xu, 2007), which might be related to the crust having been partially molten. This high-conductivity, low-density molten material might be the basis for the material extruded from the QTP's eastern margin, as well as the dynamic basis for the QTP's uplift. The low-density, high-conductivity geologic body in the middle-lower crusts is also weak, easily deformed, and has a fast creep rate, thereby facilitating adjustments to crustal thickness.
As evidenced by seismic data (Gao et al., 2009) and the Moho surface (Fig. 4a) obtained from inversion of the Bouguer gravity interface (Parker 1972), a significant trend of crustal thickening exists for both the Songpan and Sichuan-Yunnan blocks. However, the horizontal gradient modulus for Bouguer gravity anomalies (Fig. 4b) indicates the presence of multiple arcuate tectonic structures aligned in a northwest-southeast orientation within the Sichuan-Yunnan block. The Earth's surface reveals these arcuate tectonic structures to be mostly sinistral strike-slip faults. It's hard to imagine such arcuate tectonic structures being able to explain the overall extrusion of rigid material at the QTP's eastern margin.
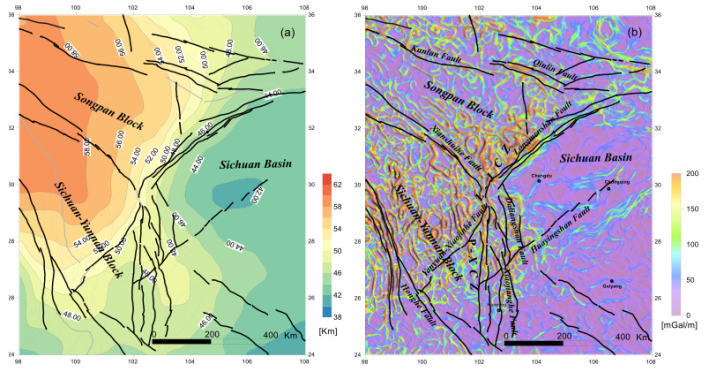
Figure 4 Geotectonic characteristics of the QTP’s eastern margin: (a) isobaths of Moho surface; and (b) horizontal gradient modulus
Driven by the effect of plate movements, the low-density, high-conductivity fluid material that is widespread in the middle-lower crusts of the QTP's eastern margin began migrating eastwards to the Longmenshan-Jinpingshan tectonic zone. It was then obstructed by the Yangzi block's lithosphere, which has the characteristics of high density and resistivity. Consequently, it gathered and accumulated along the front edge of the obstruction, forming an open density gradient zone along the northeast direction. The action of isostatic correction caused the middle-lower crustal fluid to be uplifted vertically and extended horizontally.
On the one hand, the rate of geomorphic uplift since the Quaternary reached approximately 3 mm/a. This vertical uplift resulted in a vertical height difference of more than 3,000 m between the geomorphology of the Longmenshan tectonic transition zone and that of the Sichuan Basin. The amount of uplift for the Sichuan-Yunnan block reached 1,180 m (Zhang, 2004), with many highly-angular thrust nappe tectonic structures appearing in the Longmenshan-Jinpingshan orogenic zone. On the other hand, the middle-lower crustal fluid of the Sichuan-Yunnan block spread extensively towards the southeast and south-southeast directions. Arising from the northeast-north-northeast extension of the Songpan block, the Longmenshan fault zone within the Longmenshan tectonic zone had dextral strike-slip characteristics.
After the 2008 Wenchuan earthquake with a surface wave magnitude (Ms) of 8.0, surface rupture of the central Longmenshan fault took the form of vertical uplift with thrusting circulation, as well as slipping movement in the northeast direction. The maximum amount of dextral horizontal displacement resulting from the movements was 6.2 and 4.9 m, respectively. Average offset along the entire rupture zone was 2-3 m (Xu et al., 2009).
The sinistrally-extruded Xianshuihe fault had an apparent directional change at the eastern section, connecting at an obtuse angle with the north-south aligned Anning River and the Daliangshan fault. As such, the sinistral strike-slip movement of Xianshuihe fault should have been broken down and absorbed by the blame with a near north-south alignment. This would cause east-west extrusion and crustal shortening. However, surface investigations show that the north-south aligned fault did not have any large thrust shortening components. Similar to the Xianshuihe fault, this fault also has sinistral strike-slip shearing, which is its adjustment to the eastward movement of the QTP's crustal material (Zhang, 2008).
This change forced the deep crustal fluid in the Daliangshan tectonic zone to extend towards the south-southeast and southeast directions. This manifested on the surface as the sinistral strike-slip faults in the Daliangshan series, which has similar south-southeast and southeast alignments. Since the crustal strength of the Sichuan-Yunnan block is relatively weak, the middle-lower crusts of the Panxi tectonic zone with their relatively higher conductivities and lower densities provide the open and broad channels for the fluid's southeast extension. Gradual thickening of the Earth's crust thus occurred at the 1,000 m dimension and more, causing surface uplift over extensive areas and formation of the Yunnan-Guizhou Plateau in southwest China.
4.2. Dynamic mechanism of the 2008 Wenchuan earthquake
Given the differing views on the plateau's uplift mechanism, two different models were used to explain seismogenesis of the Wenchuan earthquake, involving shortening and extrusion of the brittle crust versus expansion of the ductile lower crust (Hubbard and Shaw, 2009). After analyzing the physical properties of the crust at the QTP's eastern margin, we believe that its uplift mechanism was mainly caused by the fluid that exists extensively in the middle-lower crusts. A similar perspective was applied to the seismogenesis of the Wenchuan earthquake.
In this study, a cross-section of residual gravity anomalies was selected to analyze the deep crustal structure of the Wenchuan earthquake better. The cross-section passes through the earthquake's epicenter and straddles four tectonic units: the East Kunlun tectonic zone, Songpan block, Longmenshan tectonic zone, and Sichuan Basin. Imaging of the cross-section and its anomalies was done after regularization and downward extrapolation. The image (Fig. 5) reveals the extensive presence of negative gravity anomalies in the middle-lower crusts of the Songpan block and eastern margin of the Longmenshan tectonic zone, at a depth of 20-40 km. At the Longmenshan fault zone, a prominent positive gravity anomaly (enclosed by red dashed lines in Fig. 5) was found at similar depths as the epicenter. The deep structure is connected to the Sichuan Basin's rigid lithosphere. northern section. It was mainly a manifestation of the cumulative effect of the rigid body's shear strain energy, which was produced by the coupled movement of the upper and lower crusts of the Songpan block when the latter extended towards the northeast.
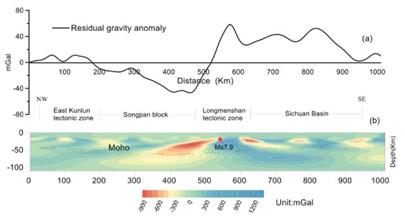
Figure 5 Residual gravity anomalies after regularization and downward extrapolation. The area enclosed by red dashed lines: high-density rock mass.
Based on the apparent density distribution within a similar depth range, the variation in apparent density for the positive anomaly was up to 0.01 g/cm3. Wang (2014) carried out MT cross-sectional imaging that passed through the epicenter and found the defect to be a high-resistivity geologic body. This cold and rigid geologic body was deduced to be located at a depth of 20-40 km and acted as a significant obstruction to the lower crustal fluid from the west, which has a negative gravity anomaly and low density.
What is the relationship between the unusual characteristic of this deep geologic body and seismogenesis of the Wenchuan earthquake? The China Crustal Movement Observation Network has a GPS network in the study area, which implemented four periods of mobile GPS observations between 1999 and 2007. The results indicate that before the earthquake, horizontal shortening and slip components were within the range of 2 mm/a for an area wider than 200 km and straddling the entire Longmenshan fault zone (Shen et al., 2005; Meade, 2007). This is consistent with the seismic and geological survey results, confirming that the rate of movement for the Longmenshan fault zone was slower.
Separately, the surface rupture zone of the Wenchuan earthquake was inclined up to 70°-80° near-surface. Precise positioning of the aftershocks indicates that the fault's inclination near the hypocenter (15-19 km) was steep (Huang et al., 2008). Retest results for seismic expedition levels further showed that the ratio of coseismic vertical displacement between the fault's upper and lower plates was as high as 8:1 (Wang et al., 2008). It was, therefore, elementary to conclude that horizontal crustal shortening did not contribute significantly to the Wenchuan earthquake.
When the Indian plate collided with the Eurasian plate, the subduction effect caused the middle-lower crustal fluid of the Songpan block to migrate eastwards and then accumulate after being obstructed by a high-density rigid body located at similar depths underneath the Longmenshan tectonic zone. The effect of isostatic movement caused the accumulated crustal fluid to act in two ways. First, it extended in a northeast direction, leading to dextral strike-slip of Longmenshan fault zone's northern section. Second, it was uplifted at the rate of 1.8 mm/a. With this vertical uplift and eastward extrusion, the accumulated fluid and rigid body generated eastward movement and uplift about each other, forming an angular thrust fault on the upper crust.
Simultaneously, strain energy was being produced, and accumulated by the fault. The Wenchuan earthquake occurred when the accumulated strain energy exceeded the rupture strength of the geologic body (Fig. 6). The Ms 7.9 mainshock actually triggered the aftershock at Longmenshan fault zone's northern section. It was mainly a manifestation of the cumulative effect of the rigid body’s shear strain energy, which was produced by the coupled movement of the upper and lower crusts of the Songpan block when the latter extended towards the northeast.
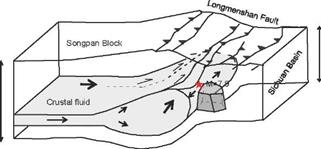
Figure 6 The Conceptual model for deep seismogenesis of the Wenchuan earthquake (Modified from Hubbard and Shaw, 2009).
The epicenters of earthquakes registered at Ms 4.0 and above, which have occurred in this tectonic region since January 1, 2008, were projected onto the density distribution map of the middle-upper crusts at 20 km depth (Fig. 7). It was found that the majority of epicenters are located in such abrupt high-low-density zones, or in areas where tectonic structures converged. Further studies can be carried out to determine whether the Wenchuan earthquake had a similar deep seismogenic process.
5.Conclusion
In this study, the density structure of the deep crust-mantle structure at the QTP's eastern margin was determined based on gravity anomaly data, while its electrical structure was determined using LMT sounding data. The uplift mechanism for the QTP's eastern margin and deep seismogenesis of the Wenchuan earthquake were then analyzed and discussed, based on distribution characteristics of the physical properties.
The findings provide us with the following understanding: (i) A low-density and high-conductivity material is extensively distributed within the middle-lower crusts of the QTP's eastern margin. After combining the material's geophysical evidence with available new tectonic dynamic characteristics, it is deemed to be a molten fluid material located in the middle-lower crust.
(ii) The apparent density distribution features and structure of the electrica cross-section indicate accumulation of the middle-lower crustal fluid material at the western side of Longmenshan-Jinpingshan. This accumulated material is the material basis and key factor behind the tectonics of the QTP's eastern margin, which includes crustal thickening, geomorphic uplift, and formation of thrust nappe structures in the orogenic tectonic zone.
(iii) The deep seismogenic mechanism of the Wenchuan earthquake is closely related to the existence of the ductile crustal fluid at the QTP's eastern margin, the similar uplift mechanism, as well as the unique physical properties of its deep structure.