Introduction
Volcanic reservoirs present a firm heterogeneity and poor physical properties. Tectonic fractures originated from tectonism constitute the main seepage paths and reservoir spaces in the volcanic reservoirs. The study of the characteristics, controlling factors, and formation mechanism of tectonic fractures in volcanic rocks play a crucial role in oil and natural gas exploration and development. As several authors point out, the study regarding the growth characteristics and the division of the development phase in tectonic fractures constitutes a problem to tackle with (Nelson, 2001; Larsen, Grunnaleite & Gudmundsson, 2010).
The Zhongguai area is located at the Northwestern margin of the Junggar basin. The Carboniferous system is one of the primary hydrocarbon-bearing sources in the region with the main reservoir rocks being volcano ones. Several years of exploration at the Zhongguai area were needed to achieve a breakthrough series of oil and gas exploration in the Carboniferous volcano rock reservoir. Commercial oil flow was obtained in many testing wells, in which H019 well emitted a daily oil mean of 105.96 t/d and a (daily) gas mean of 2. 14 χ 103 m3 in the Carboniferous oil test, a reasonable prospect for a gas and oil exploration in the area of volcanic rocks. However, from current tests of oil and gas production, the variation of the single well productivity becomes larger (a unique well oil production from 0.1 to 105 t/d), displaying the typical properties of a fractured reservoir, with the fracture effect in the production control being significant. The frequent tectonic alteration experienced in the area contribute to relatively developed fractures. It is worth mentioning that fractures at different stages play a crucial role in the regulation and controlling of hydrocarbon migration and accumulation. Also, fractures become essential in high yields (Fan & Qin, 2016).
In this paper, the authors have analyzed the characteristics of the fracture's development and carried out a preliminary qualitative division of fracture formation times by combining structural analysis and core observation in the study area with imaging logging interpretation. Additionally, they have contributed a quantitative division of fracture development phases by an acoustic emission experiment of rocks and a fracture filling inclusion (Warren et al., 2017; Yang et al., 2018; Fu & Liu, 2017).
Geological Characteristics
Zhongguai area is located at the conversion parts of the fault belts of Ke-Wu and Hongche, in the Northwestern margin of Junggar basin. Also, it plunges to that basin from the Hongche one to the Southeast. Three sides of the Zhongguai area face the concave (adjacent to MaHu sag, pcn1Well west sag, and Shawan sag), and it is a favorable directional zone for oil and gas migration and accumulation (Figure 1). Its main form is a wide flat nose paleo-uplift, which is formed under the action of extruding stress field in the Carboniferous to early Permian. It started to hide at the end of Permian; overlap was deposited on a raised in Wuerhe Formation (Upper Permian) and angular unconformable contact with the Jiamuhe Formation of the Permian; the Triassic-Jurassic draped on top of a raise was a smaller bulge. Yanshan movement caused an uplift further, so the tectonic pattern formed to single southeast tilting, and upwelling area tilted eventually in the Himalaya movement period.
Volcanic rock in the research area buries deeply. Composing the basal part of the basin, which is mainly developed by the interaction between continental-oceanic volcanic rock and environment, primarily includes volcanic lava (andesite and basalt), pyroclastic rocks (volcanic breccia and tuff), and few intrusive rocks (granite), with tuff and andesite being widely distributed. In that area, the volcanic rock reservoir physical property is reduced. The average porosity range fluctuates from 5 to 7 %. Its matrix permeability value is generally less than 1χ10-3 μm2, with a mean value being only equal to 0.61χ10-3 μm2. As such, it corresponds to a low reservoir not only in porosity but also in permeability.
Since Carboniferous, the tectonic fracture of such a reservoir has been growing more and more due to frequent tectonic movements leading to advantageous accumulation places. Also, the infiltration channel of the reservoir in this area exhibits a significant influence on oil and gas enrichment and development effect. Therefore, research on layer fracture characteristics, formation stage, and flow characteristics of the volcano rock reservoir in that area becomes crucial for volcanic rock reservoir exploration and efficient development.
Development Characteristics of Structural Fractures
The core observation regarding 596, H56A, H019, and 16 wells suggests that Carboniferous volcano rock reservoir in Zhongguai area could be divided into four types of fractures, namely, diagenetic, structural, weathering, and solution ones. First, tectonic fractures are mostly developed and widely distributed in various types of volcano rocks. According to the statistics of tectonic fracture dips, it holds that fractures can be divided into two categories: a class of high angle of inclination > 60° seam and straight seam for about 70 %. Another kind of dip is at 30° - 60° oblique fractures, accounted for about 20 %. The high angle fractures are mainly shear ones, characterizing the joint surface flat rules, stable occurrence, visible scratches, mineral filling, and so on (Figure 2-a, Figure 2-b). Oblique fractures mainly include shear and tensile ones. The different characteristics among the fractures of an intersection intersperse with others, leading to a connected seepage system. In this way, the development and distribution of the volcano rock in Carboniferous reservoirs play a crucial role in controlling.
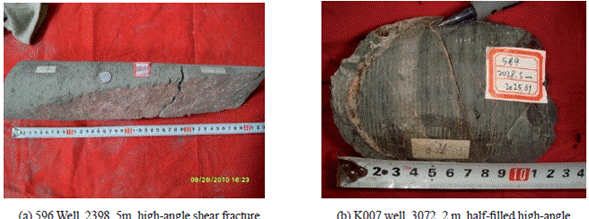
Figure 2 Characteristics of volcano rock reservoir fractures in the Zhongguai uplift Carboniferous development.
According to some hydraulic fracturing experiments and the analysis of the fracture azimuth imaging logging after treatment, the fractures mainly developed are located at NE (45°± 15°), NW (300°± 15°), EW (85°± 10°), and SN (0°± 10°), with NE (45°± 15°) and EW (85°± 10°) being the ones mostly developed, followed by NW (300°± 15°). On the other hand, the worst is close to SN (0°± 10°). It is worth noting that fracture filling material mainly consists of calcite, chlorite, and gypsum; fracture filling degree is high; around 60 % of fractures are wholly filled; half-filled fractures and not filled ones accounted for 40 %.
The imaging logging analysis results point out that fractures are concentrated in the 2-5 strips/m, followed by 0.4-0.6 strips/m, with the overwhelming majority fracture density corresponding to a medium value < four strips/m. The scope of the fracture length change is broad. The long range between 10-30 cm accounts for more than 70%, and the maximum cutting depth can reach 180 cm. Fracture width is in the range of 1-5 mm; in the local core exist open fractures whose fracture width is > 1 cm. Based on the field investigation and statistics of fracture spacing, it holds that the fracture spacing range goes from 0.3 to 0.7 m, and the average spacing equals 0.5 m.
Discussion on Fracture Growth Stages
In recent years, the major technologies in the research on fracture development periods involve (1) geological methods, including analysis of regional construction features, investigation of fractures in outcrop area, outcrop fractures, and fracture characteristic analysis of survey underground (Lee, 2001; Espinosa, 1998); (2) lab test methods, including filling isotope analysis, inclusion thermometry, and rock acoustic emission testing (Lopez-Puente et al., 2005; Tasdemirci et al., 2012; Lankford, 2004; Lausund et al., 2015), as well.
This article study is mainly focused on the core of fracture filling composition and mutual cut features, fracture filling, the homogenization temperature of hydrodynamic inclusions, and rock acoustic emission methods to explore the volcanic fracture formation period of Carboniferous in Zhongguai area.
The fracture character of core reflects the fracture stage
The primary approach to explore the fracture stage involves the core fracture azimuth, the filling ingredients, and the interaction cutting relationship among the fractures to analyse the fracture formation stage.
Results from the fracture filling material composition and the interaction cutting relationships (Figure 3a and 3b) combined with an image logging analysis show that the Carboniferous volcanic rock fracture at the Zhongguai area are mainly divided into four stages. As such, the first stage of fractures is close to both EW (85°± 10°) and NW West (300°± 15°), with the fillings mainly consisting of calcite and chlorite. The second fracture is NE (45°± 15°). Its fillings are primarily gypsum. The third one appears at both EW (85°± 10°) and NW West (300°± 15°), whose fillings mainly consist of calcite. Finally, the fourth phase primarily corresponds to both SN (0°± 10°) and NW West (300°± 15°) to fracture, with no filling practically.
Homogenization temperatures of fracture filling inclusion
In recent years, the analysis of fracture filling inclusion lies in a mature technology allowing researching features of fracture filling inclusion and measuring the homogenization temperature. It is worth mentioning that the temperature filling formation can be obtained to determine both the formation period and the mineral filling stage (Johnson & Cook, 1985).
The fourth stage is almost not filled. The present study is focused on 8 samples and 46 test points of fracture fillings from the first three different cutting stages to test the homogenization temperature, according to the various stages of the temperature distribution of fracture fillings and their morphological characteristics. The three filling times of tectonic fractures in that area can be distinguished in the following terms. The homogenization temperature of the first phase ranges from 75 to 82 °C. The second homogenization temperature lies in the range 92-97 °C, with the third homogenization temperature ranging from 106 to 112 °C. There are, at least, four stages of tectonic fractures under the assumption of fractures from the last period with almost no fillings (Figure 4).
Acoustic emission experiment of rocks reflects the fracture formation period
The formation of rocks experienced stress effects in the long run with a memory function of stress. In the early sixties, it was confirmed by Goodman that rocks also exhibit a "Kaiser" effect. The acoustic emission test is supported by the fact that there exists a Micro Griffith fracture generated by the ancient tectonic stress field in the underground rock stratum. Only if the force exceeds the stress intensity, the fracture can extend and lead to a plurality of different Kaiser effect points. More recently, the continuous evolution of technology expands its applications to fault evolution history, fracture stage by matching and ensuring the intensity of fracture formation stress fields (Kresse et al., 2013; Meyer & Bazan, 2011).
The present study consists of an acoustic emission experiment regarding the Kaiser effect involving 12 pieces of Carboniferous volcanic rocks from the Zhongguai area. The experimental results suggest that our sample generally presents five obvious Kaiser effect points. One of them stands as a result of the tectonic stress effect, whereas the others highlight that rock experienced four micro-fracture events along its geological history. Combined with regional tectonic evolution characteristics and research results, it was observed that the first fracture development stage, a product of the middle Hercynian tectonic movement, with a paleotectonic stress of 49.6 MPa. The second fracture development stage is a product of the late Hercynian tectonic movement and presents a paleotectonic stress of 39.9MPa. The third fracture development stage is a product of the Indosinian tectonic movement, whose paleotectonic stress is 34.1 MPa. Finally, the fourth fracture development stage is a product of the Yanshan tectonic movement, and its paleotectonic stress is 26.5 MPa (Figure 5).
Seepage Characteristics of structural fractures
Fractures in low porosity and low permeability reservoirs are crucial to reforming the reservoir physical property and also concerning the accumulation of oil and gas. Fractures tend to control the hydrodynamic seepage network of reservoirs (Wu, 2015). From the Carboniferous porosity-permeability relationship diagram in the Zhongguai area, it can be observed that the correlation between porosity and permeability is not good. The correlation coefficient is only 0.4257 (Figure 6), with the difference between the porosity of the development samples and the porosity of the fissureless samples being quite poor. However, the permeability of the fracture development samples stands from 3 to 5 orders of magnitude higher than the permeability of the fissureless samples.
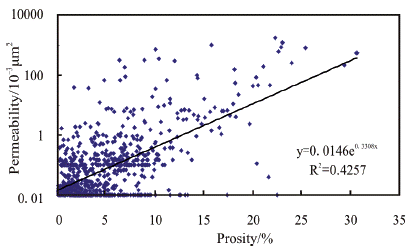
Figure 6 Reservoir porosity and permeability correlations of the Carboniferous volcanic reservoir at the Zhongguai Area.
For instance, the permeability of the vast majority of the fissureless sample at the work area lies in the range going from 0.01χ10-3 μm2 to 1χ10-3 μm2. The fracture development samples, such as andesite cores at 3366 m, developed fractures in 598 wells. The penetration rate in the tested samples is as high as 354.14χ10-3 μm2, illustrating that the presence of fractures has a significant influence in the improvement of both the Carboniferous volcanic reservoir property and hydrocarbon accumulation. As such, drilling in the fracture development areas will result in better oil testing results. Fractures on the longitudinal direction are often more extended, though the fracture interval on the lateral is larger and the drilling rate of the vertical well is low. Therefore, directional drilling is typically used in the drilling process to improve the fracture rate of the drilling.
Moreover, both the fracture system and the period in that area have been analyzed. It holds that the development situation and the lithology (tectonic stress, tectonic position, and thickness) are closely related. The fracture opening at different periods and distinct ranges in the underground reservoir are inconsistent, the difference of permeability is significant, and it is closely associated with the field direction of the current stress. The present principal stress orientation is close to EW (270°± 10°) with the degree of open fractures being parallel to the predominant stress orientation closely to EW (85°±10°), that is the highest underground. The fracture permeability of that group is the largest, with an effective permeability of 43. 2χ10-3 μm2. Secondly, the average permeability value of fractures that present a sharp angle with the principal stress orientation of NW (300°±15°) and NE (45°±15°) is 18-24χ10-3 μm2. The opening and permeability of SN (0°±10°) are the lowest, with a permeability value equal to 2-10χ10-3 μm2. Further, the hydrodynamic seepage velocity in the fracture closely to EW (85°±10°) in the early development is the fastest, whereas the hydrodynamic seepage velocity in the fracture closely to SN (0°±10°) is the slowest. Notice that both the hydrodynamic seepage velocity and the dynamic response in the NW (300°±15°) and NE (45°±15°) fractures lie between the corresponding values of EW (85°±10°) and SN (0°±10°) fractures. Thus, the fracture permeability anisotropy of different groups in the Carboniferous volcanic rock reservoir is evident. Accordingly, the effect of fractures closely to EW (285°±10°) should be considered firstly in the deployment of development scheme of volcano rock reservoir in this area with the aim to systematically valuate distinct tectonic fracture seepage characteristics. In various stages of oil and gas field development, it holds that the dynamic changes of fracture permeability in different groups are separate from one to others due to the fracture permeability and seepage velocity. In this way, both the fracture opening and permeability closely to EW (285°±15°) is the greatest. Drop speed of hydrodynamic pressure and fracture opening within the reservoir is faster in the process of oil well production. Such a group of fracture seepage effect on oil and gas weakened gradually, while descent speeds of the hydrodynamic pressure and fracture opening of fractures in NW (300°±15°), NE (45°±15°), and SN (0°±10°) fall slower than the one closest to EW (285°±15°). With the oil field development process, the dynamic response of fractures in NW (300°±15°), NE (45°±15°), and SN (0°±10°) will be more and more evident, allowing to adjust the well's pattern.
The fractured volcanic reservoir exhibits less sensitive mineral and fractures developments. To confine pressure changes of fractures in underground reservoirs will arouse fracture opening and permeability changes. Also, its reservoir sensitivity presents typical characteristics of stress sensitivity, being this one of the critical factors leading the volcano rock fractured reservoir yield decrease rapidly.
The stress sensitivity refers to the degree of change of the rock permeability caused by the effective stress change in the rock skeleton. Volcano rocks have the priority since their body deformations belong to the dense medium. The calculation of the effective stress can be performed throughout the following expression from the Double Effective Stress Theory:
In the formula above, denotes the effective primary stress, σ is the overburden pressure, p is fluid pressure, and φ denotes the porosity.
Due to the different rates of fracture occurrence and degree of development, the fractured reservoir stress sensitivity changes. In this way, the considerations that follow should be stated. A total amount of 12 different fracture occurrences of andesite and tuff samples in the area were selected, taking advantage of an automatic core displacement system to test stress sensitivity. In both Figures 7 and 8, a high-angle fracture stress sensitivity experiment is developed for oblique fractures with a different fracture occurrence and a nonidentical degree of stress change sensitivity. Using 20 MPa as the standard, the permeability damage rate of the oblique fractures was equal to 60 % (Figure 7). The permeability damage rate of high-angle fracture is less than 40% (Figure 8). The obtained results illustrate that the fractures from the oblique fracture samples are easily closed when the confining pressure drops. Also, the higher the core loses its permeability, the stronger it is stress sensitivity.
On the other hand, the fractures from the high-angle samples are hard closed when the confining pressure drops. As such, the permeability of the core loss becomes smaller as long as the stress sensitivity is relatively weak. Visibly, for fractured volcano rock reservoir, the pressure change in the process of exploitation may cause severe damage to the reservoir permeability. Accordingly, to be focused on targeted measures of exploitation results entirely necessary in the process of reservoir development in fractured volcano rocks.
Conclusions
The main conclusions in this paper are stated below.
(1) Regarding the structural fractures of Carboniferous volcano rock reservoir being developed at the Zhongguai area. Fractures constitute a crucial factor regarding space and seepage channels in volcanic rock reservoirs. They are mainly of oblique and high-angle-shear types. The locations of such a fracture development are close to EW (270°± 10°), NW (300°± 15°), NE (45°± 15°), and SN (0°± 10°). It is worth mentioning that these intertwine with each other leading to a fracture network system, which is favorable for oil and gas accumulation and high production.
(2) The Carboniferous volcanic rock fracture at the Zhongguai area could be divided into four main stages according to the core observation and the temperature of homogenization of the fracture filling inclusion together with the regional tectonic evolution features.
(3) Structural fractures play a crucial role regarding volcanic rock reservoir seepages and accumulation of oil and gas. Fracture system more abounds in that area. It has been observed that the opening degree and the fracture seepage effect differ from one area to another for different groups. To properly arrange the development of well's patterns, the impact of fracture seepages should be thoroughly considered. In various stages of the development of oil and gas fields, the dynamic changes of permeability in distinct groups are nonidentical. As such, the well's pattern should be timely adjusted.