Introduction
The Jiyang Sub-basin is a secondary structural unit in the Bohai Bay Basin, which contains rich oil and gas resources; therefore, it has significant potential for exploration and development (Pang et al., 2003; Li et al., 2006; Jin et al., 2009). Recent studies investigating hydrocarbon accumulation in the Jiyang Sub-basin have focused on Palaeogene and Neogene sand-mudstone reservoir systems and associated Cenozoic source rocks that include multilayer organic-rich argillaceous strata (Zhu et al., 2005, 2007; Zhang et al., 2016). However, the deeper Carboniferous-Permian (C-P) coal measures have received little attention (Hou et al., 2008; Zhang, 2012). Owing to the presence of faults and surface unconformities, the formation of a petroleum system consisting of Cenozoic oil and gas reservoirs from Palaeozoic source rocks remains problematic unless structural conditions are favorable (Zhang et al., 2004, 2009b). Previous studies have shown that coal-measure source rocks have a high potential for gas generation, and coal-derived gas has thus become a key research focus for future oil and gas exploration and development in China (Dai, 2007, 2009; Yang and Liu, 2014; Liang et al., 2015).
Previous works have demonstrated that C-P coal-measure source rocks from the Jiyang Sub-basin possess favorable characteristics for secondary hydrocarbon generation because of their complex, multi-stage tectonic history (Zhang et al., 2005; Hu et al., 2006, 2008). Variations in the tectonic thermal evolution during different periods have impacted organic matter maturity, while secondary hydrocarbon generation is common in the study area (Li et al., 2001; Li, 2006; Fan et al., 2008). However, to date, few specialized quantitative studies have investigated hydrocarbon generation thresholds and the relationship between subsidence rates and the evolution of vitrinite reflectance (VR ) in the Jiyang Sub-basin.
This study initially reconstructed the burial and thermal histories of C-P coals using PetroMod software modeling. Secondary hydrocarbon generation for C-P coals was evaluated in terms of these events in an attempt to understand the relationships between burial, thermal history, and the evolution of hydrocarbon generation. A series of threshold depths and favorable areas were then proposed based on geographical differences as well as the delay effect in secondary hydrocarbon generation, and the quantitative relationship between VR and the subsidence rate was discussed according to changes in VR and burial depths. The aim of this study was to confirm secondary hydrocarbon generation occurred and establish a relationship between burial history and the evolution of source rock maturity in the Jiyang Sub-basin.
Regional geological setting
The Jiyang Sub-basin is located within the southern part of the Bohai Bay Basin, China (Fig. 1a). Affected by multi-stage tectonic activity, this sub-basin has a complex structural framework (He et al., 1998; Zong et al., 1999). The residual Carboniferous and Permian strata in the Jiyang Sub-basin is distributed from northeast to southwest, matching the regional tectonic framework, and the Jiyang Sub-basin is bounded by the Chengning uplift to the northwest and the Luxi uplift to the south (Fig. 1b). The basic tectonic framework includes an extensional opening in the northeast and convergence in the southwest.
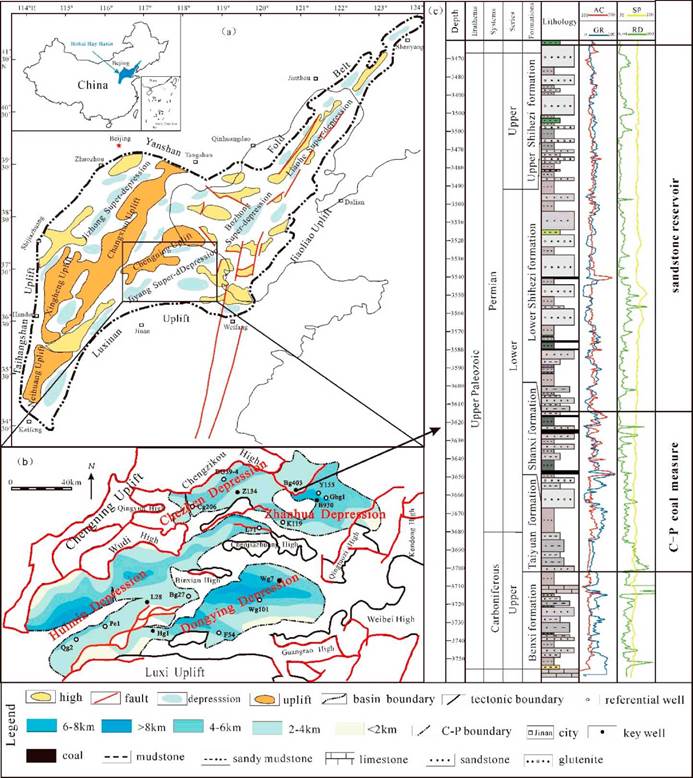
Figure 1 a. Tectonic framework of the Bohai Bay Basin, China (Han et al., 2019). b. Burial depths of Carboniferous-Permian (C-P) coal-bearing sequences within the Jiyang Sub-basin (Li et al., 2004). c. Typical lithologic and electric logs of C-P strata, including coal measures and overlying interbedded sandstones and mudstones.
The C-P coal-bearing sequences that occur within the Jiyang Sub-basin likely formed in a peat-swamp environment that included both littoral and paralic transitional facies (Zhang et al., 2009a). Following deposition of these coal-bearing sequences, convergence of the Yangtze and North China plates led to tectonic inversion during the early Yanshan epoch. After uplift and denudation, extension started during the middle and late Mesozoic, while magmatic activity was widespread across the Jiyang Sub-basin (Ren et al., 2002; Yang et al., 2008). Thus, C-P coal-bearing sequences were subject to large changes in temperature due to the hydrothermal influence of magmatic activity (Li et al., 2001, Yang et al., 2008). The embryonic Jiyang Sub-basin formed during the late Jurassic and early Cretaceous when this region underwent a slight uplift, followed by polycyclic alternation of extension and sharp thermal subsidence (He and Wang, 2004). The Jiyang Sub-basin was affected by both the Yanshan (from 205 Ma to 65 Ma) and Himalayan (from 65 Ma to the present) tectonic episodes, leading to the formation of a Mesozoic-Cenozoic rift-depression sub-basin (Zong et al., 1999). Secondary tectonics within the Jiyang Sub-basin, including highs and depressions that formed during the late Mesozoic and Cenozoic, can be divided into southern and northern sections by the Wudi-Binxian-Chenjiazhuang highs (Fig. 1b). The four dominant secondary tectonic units within this region are the Chezhen, Zhanhua, Huimin, and Dongying depressions. The basic tectonic attributes of these depressions include a northern fault and southern overlap (Wang et al., 2002).
It is also worth noting that several C-P strata in the Jiyang Sub-basin were exhumed and eroded during the later phases of Mesozoic-Cenozoic tectonic activity. Residual strata within these coal-bearing sequences are bounded at the north and south and are unevenly distributed in segments of the Sub-basin (Fig. 2). Drilling data and previous studies (Li et al., 2004) showed that the depth of the C-P coal-bearing sequences generally exceeds 2,000 m, with an average between 3,000 and 5,000 m (Fig. 1b). Coal is preserved in the Shanxi Formation (Fig. 1c) and is considered to be main source rock in the coal-bearing sequences. The VRo of the C-P coals shows a scattered distribution and is restrained by C-P residual strata. Areas with coals with high VRo are distributed on the south slope of the Dongying Depression, in the Gubei District in the Zhanhua depression, and south of the Huimin Depression. The VR of coals in these areas exceeds 1.0%, and even exceeds 1.5% in the center of these areas (Han et al., 2017). Several measured VRo values provided by the Geological Scientific Research Institute, Shengli Petroleum Administration (GSRISPA) from different wells are reported in Table 1.
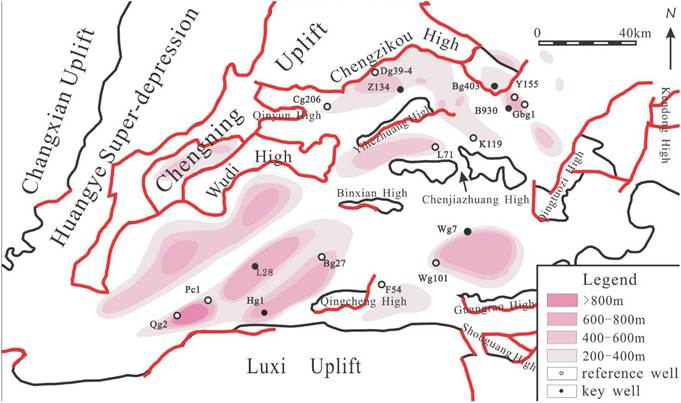
Figure 2 Thickness distribution of residual C-P strata within the Jiyang Sub-basin (unpublished data from the Geological Scientific Research Institute, Shengli Petroleum Administration, China).
Table 1 Measured VR values for Carboniferous-Permian (C-P) coal measures within the Jiyang Sub-basin
Depth (m) | Well | Sample | Mean VRo (%) | Standard deviation | Lithology |
---|---|---|---|---|---|
2890.4 | Gbg1 | Core | 0.82 | 0.03 | Coal |
3014.34 | Gbg1 | Core | 0.74 | 0.03 | Coal |
3032.5 | Gbg1 | Core | 0.83 | 0.05 | Coal |
3325 | Wg7 | Core | 1.14 | 0.15 | Coal |
3636 | Wg7 | Core | 1.32 | 0.09 | Coal |
4305.6 | Bg403 | Core | 6.52* | 0.25 | Coal |
4313.1 | Bg403 | Core | 6.6* | 0.22 | Coal |
4369 | Bg403 | Core | 1.05 | 0.06 | Mudstone |
4473 | Hg1 | Cuttings | 2.19 | 0.14 | Mudstone |
4480 | Hg1 | Cuttings | 2.22 | 0.11 | Coal |
4504 | L28 | Cuttings | 2.23 | 0.14 | Mudstone |
4515 | L28 | Core | 1.09 | 0.08 | Coal |
4539 | L28 | Cuttings | 2.73 | 0.1 | Coal |
Values marked with * are abnormally high because the C-P coal measures were heated by magma. At the same time, VRo values for cuttings are also high because these are mixed with cavings, impacted by contact metamorphism from igneous bodies with high VRo values.
Reconstruction of burial, thermal, and maturity histories: methods and input data
Burial, thermal, and maturity histories were reconstructed by applying a basin modeling technique (using the commercial modeling software package PetroMod, 2010 version). The purpose of this evaluation was to reconstruct thickness and temperature conditions during the geological history of the Jiyang Sub-basin on the basis of observed present-day strata thickness data and the geothermal field (Shi, 2000; Pang, 2003). Input data consisted of formation depths (m) and associated lithologies, numerical ages (Ma), well temperatures (°C), eroded time (Ma) and depths (m), petroleum system element (as source, reservoir, or seal), total organic carbon (TOC, %), source rock kinetics, and the initial hydrogen index (HI). This method yielded reconstructions of burial, thermal, and maturity histories of the target source rocks. Before basin modeling, a back-stripping method (Steckler and Watts, 1978) was used to obtain the eroded thickness in different geological periods. The back-stripping method is based on sediment compaction theory, and decreases in the thickness of strata are a result of increases in burial depth because the overlying load causes a decrease in porosity (Sclater and Christie, 1980). Thus, the basis for this method is the establishment of relatively accurate sandstone-mudstone compaction curves. Because the porosities of sandstone and mudstone affected by their depths, it is possible to establish compaction curves that reflect the relationship between porosity and burial depth. These relationships can be observed in the logging results (Fig. 3). The calculation of eroded thickness in different erosion events are based on sandstone and mudstone acoustic travel time. The values of sandstone and mudstone acoustic travel time reflect rock compaction, i.e. porosity. The amount of erosion can be determined by discerning gap of acoustic travel time and calculating depth difference. A detailed method for calculating erosion amounts in geological periods was proposed by Magara (1976). Initially, the presence of two main erosion surfaces on compaction curves was confirmed by logging observations of the erosion surfaces. One of these was in the Palaeozoic-Mesozoic and the other was in the Mesozoic-Cenozoic. Eroded thicknesses could then be calculated from logging results provided by GSRISPA (Table 2), except for Palaeogene and Triassic erosions. In addition, according to calculations by Du (2005), there is a erosion between the Paleogene and Neogene and the eroded thickness of this period is 150 m. Owing to the lack of Triassic stratigraphic information, this study referred to the result reported by Zhu et al. (2001), which suggests that the erosion amount at this time was 1800 m in the southern Jiyang Sub-basin.
Table 2 Key input parameters for the basin model associated with C-P coal measures in the Jiyang Sub-basin
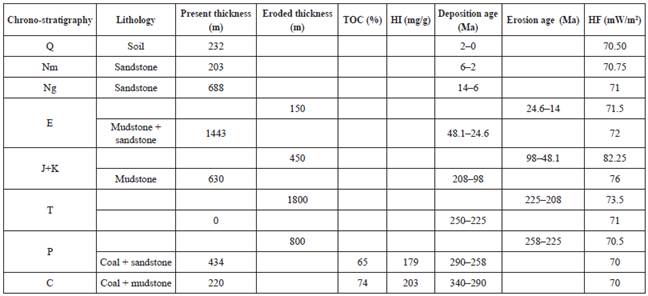
Although the data in this modeling were from different sources, they had a credible matching. This is because the non-well drilling data, like HF value and ages, had a high regional consistency. Additionally, the TOC and HI values were directly obtained from GSRISPA and the coal sampling sites were located in the uplift in the Jiyang Sub-basin. Therefore these coals could represent the low rank coals which were preserved at deep depth in the depression. The detailed input parameters used for this model are listed in Table 2. The chronostratigraphic units are based on results from actual logging, while event ages and heat flow (HF) values were taken from the work by Du (2005). The designated land surface temperature for this work was 14°C and was considered to have been constant throughout geological history. The EASY%Ro VRo evolution algorithm of Sweeney and Burnham (1990) was applied to model thermal maturation. The detailed modeling procedure using PetroMod 1D was reported in previous studies (Yu et al., 2018, 2020). The modeling results obtained correlate very well with the current measured temperature and VRo data (Fig. 4b).
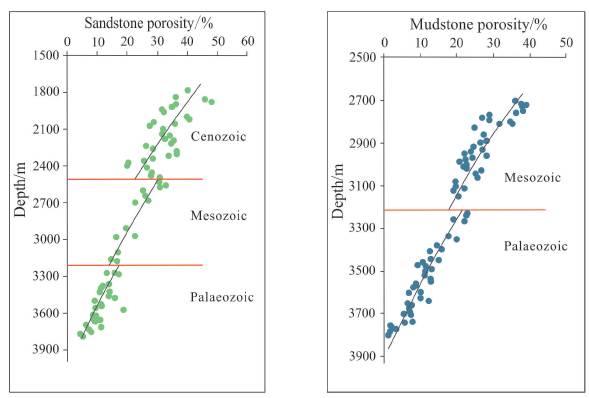
Figure 3 Mudstone and sandstone porosities for different depths from well Wg7 in the Jiyang Sub-basin. The unconformity between Triassic and Jurassic strata cannot be discerned in that Triassic strata were missing in well Wg7.
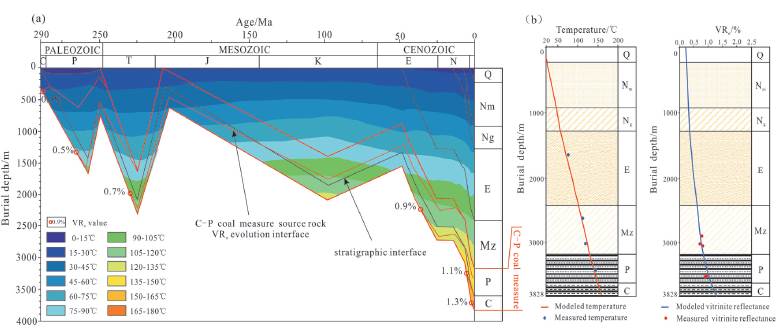
Figure 4 a. Wg7 well modeling results for burial, thermal, and maturity histories. b. Calibration curve for reconstruction of temperature and VRo (Data were obtained from GSRISPA).
The TOC and HI values for C-P coals were directly obtained from GSRISPA. The Permian coal-bearing strata are major reservoirs due to a high adsorption capacity of coal and pore space of sandstone.
Results and discussion
Reconstruction of burial, thermal, and maturity histories
As discussed, the modeling here was based on measured present-day thicknesses and partially eroded thicknesses from well Wg7 and others, as presented in previous works (Zhu et al., 2001; Du, 2005). The depth of C-P coal measures in well Wg7 has a similar depth with their average depth in the study area (3500-4000m). The logging observations and previous studies suggest complete erosion events after the deposition of C-P coal measures and the source rocks has not been affected by a direct magmatic intrusion. The thickness of coal is equivalent to the average value of coals in the study area (10-20m) and the vitrinite reflectance of coals in well Wg7 is about 1.3%, which also represents the deep C-P coals in the Jiyang Sub-basin. Therefore, our modeling results can represent the burial, thermal and maturity histories of deep C-P coal measures in the study area. The modeling results, including burial depth and temperature data (Fig. 4a), allowed for the determination of VRo throughout geological history and suggested that the C-P coals experienced four developmental stages in the Jiyang Sub-basin.
(1) Alternating patterns of subsidence during the Indosinian epoch (from 290 Ma to 225 Ma)
The deepest burial depth recorded during the Indosinian epoch exceeded 2,200 m and the temperature in the center of this depression exceeded 105°C. Modeling results show that the temperature of the primary hydrocarbon generation was 80°C, and that during initial burial to 1,700 m at 260 Ma, the VRo value for the C-P coal-measure source rocks approached 0.6%, which is the primary hydrocarbon generation threshold. During the late Permian, C-P coal strata experienced a short period of uplift and exhumation. Following a period of exhumation (which ended at 250 Ma), re-burial to 2,300 m led to an increase in VRo to 0.75%.
(2) Uplift in the Early Yanshan epoch (from 225 Ma to 200 Ma)
During the early Yanshan epoch, C-P coals were exhumed again by a north-to-south compressional stress field, which resulted in the denudation and stagnation of hydrocarbon generation. At this time, significant hydrocarbon generation did not occur in source rocks despite being within the early gas generation window (VRo = 0.7-0.8%) for humic coals (Price, 1989; Zhao et al., 2005). VRo values remained at around 0.75%, but favorable conditions for secondary hydrocarbon generation nevertheless occurred.
(3) Subsidence and small-scale exhumation during the middle to late Yanshan epoch (from 200 Ma to 40 Ma)
The maximum burial depth throughout the entire Yanshan epoch did not approach that of the Indosinian epoch. The maximum burial depth of around 2,100 m was attained at about 100 Ma, while the maximum temperature remained close to 120°C. These results show that regional differences in the amount of subsidence led to variation in thermal maturation. The VR value reached as high as 1.0% in the depocenter, where source rocks approached peak hydrocarbon generation; however, in specific areas, source rock maturity (heated by magmatism) increased above the window for oil generation (VRo > 1.8%, affected by igneous heat). These results are similar to those reported for the Gudao area in the Zhanhua depression, although the distribution area for secondary hydrocarbon generation is relatively limited (Li et al., 2006; Fan et al., 2008).
(4) Rapid subsidence during the Himalayan epoch (from 40 Ma to the present)
The C-P coals developed into their present state as a result of more or less continuous subsidence over the last 50 Ma. The burial depth in the center of the Jiyang Sub-basin commonly exceeded 3,500 m and the highest temperature exceeded 165°C. This means that the burial depth increased by more than 2,000 m over just 40 Ma. At the same time, the geothermal gradient increased substantially (from 90°C to 170°C), and VRo attained a high maturity stage (VRo > 1.3%). This indicates that the VRo distribution areas were also consistent with those of secondary structures (Fig. 5). The distribution of VRo values that reached high maturity (VRo > 1.5%) are approximately annular in the Zhanhua and Dongying depressions; however, in the Chezhen depression, VR values cluster around 1.0%, and form a banded distribution. In contrast, close to the fault zone of the north-south partition in the Huimin depression, VRo values are relatively high (VRo > 1.2%). This maturity level corresponds with the peak for secondary hydrocarbon generation within the Jiyang Sub-basin (Li, 2006; Zhu et al., 2010). The C-P coals in the Jiyang Sub-basin are currently at the peak stage of secondary hydrocarbon generation and thus contain a considerable proportion of gaseous hydrocarbons. These source rocks are promising for exploitation of coal-derived gas if the preservation conditions and gas migration pathways are well matched.
Evolution of hydrocarbon generation in C-P coals
Source-rock kerogen types are determined based on the sedimentary environment. Observations showed that terrestrial forest bogs were widely distributed over the deltaic plain, corresponding to a weak reducing, or weakly oxidizing, environment (Zhang et al., 2009). In this context, higher plant remains tended to form humic organic matter following deposition and coalification. Coal petrography observations identified vitrinite as the dominant source-rock maceral component (>70%), while the inertinite content was less than 30% alongside a limited amount of exinite (Song and Qin, 2005). Organic geochemical results for C-P coal-related source rocks also demonstrated a type III kerogen with a low H/C ratio but intermediate O/C ratio (Yu et al., 2003). Thus, humic kerogen (type III), which leads to the generation of natural gas, forms the main source rock type within C-P coal measures (Ai and Li, 1999). Minimal differences in source-rock maceral content across all depressions imply that C-P coals in the Jiyang Sub-basin are products of a similar depositional setting. However, the cyclic subsidence-uplift pattern indicates that the tectonic framework of this sub-basin is very complex, resulting in the uneven distribution of C-P coals and regional differences in the evolution of hydrocarbon generation.
Relationship between temperature and VRo values in organic matter
Following the deposition of C-P coals in the Jiyang Sub-basin, these units were subject to multiple phases of tectonic activity that resulted in a number of different characteristics during the Indosinian, Yanshan, and Himalayan epochs. Temperature data and curves that illustrate the evolution of VRo for C-P coals in the Jiyang Sub-basin are presented in Fig. 6 and identify four hydrocarbon-generation stages. The divisions between these stages are based on burial history and maturity variations and include the maximum depth for primary hydrocarbon generation, recovery of maturity following extended stagnation, and accelerated increase in maturity due to rapid subsidence. The temperature curve is highly variable but nevertheless conforms to a generally increasing trend, while the VRo curve exhibits a smooth and uniformly increasing trend. The VRo values tend to increase, consistent with temperature, while the temperature decrease led to cessation of VRo evolution. Thus, both decreasing and recovering temperature stages can be considered as stagnation phases in the evolution of hydrocarbon generation. The modeling results also show that the initial temperature for secondary hydrocarbon generation was lower than the maximum temperature for primary hydrocarbon generation (Zou et al., 2000). This was possibly due to the low degree of initial evolution, with VRo only reaching 0.8%. This "stagnation" stage resulted in residual organic matter retaining a high potential for secondary hydrocarbon generation (Gao et al., 2007).
Characteristic features and the delay effect for secondary hydrocarbon generation
As a result of the complex tectonic evolution of the Jiyang Sub-basin, C-P coal-bearing sequences have generally experienced secondary hydrocarbon generation, controlled by temperature and initial source rock maturity (Hunt, 1991; Zou et al., 2000). Two features of secondary hydrocarbon generation characterize the study area. The first is discontinuities in the history of hydrocarbon generation due to changes in temperature that resulted from burial, exhumation, and re-burial. Notable gaps between primary and secondary generation are evident in Figure 6. The second characteristic feature is regional differences in maturity controlled by burial depth across the study area (Fig. 5). Thus, based on the results in Fig. 6, two distinct phases of hydrocarbon generation can be identified encompassing the Yanshan (from 170 Ma to 100 Ma) and Himalayan stages (from 40 Ma to the present). Secondary hydrocarbon generation ceased in the Jiyang Sub-basin between 100 Ma and 40 Ma because burial depths were too shallow at this time and the temperature decreased to less than 80°C. From a spatial perspective, source rocks at different burial depths differ in their hydrocarbon-generating intensity, as indicated by experiments modeling secondary hydrocarbon generation (Jin et al., 2009) because different temperatures lead to differences in organic-matter maturation. The experimental samples used for this study were immature coals from the Jiyang Sub-basin, and a quantitative relationship between VRo and temperature and hydrocarbon productivity was obtained. Thus, using the hydrocarbon productivity modeled by Jin et al. (2009) (Fig. 7) and current VRo values (Fig. 5), the intensity of hydrocarbon generation could be calculated (Fig. 8).
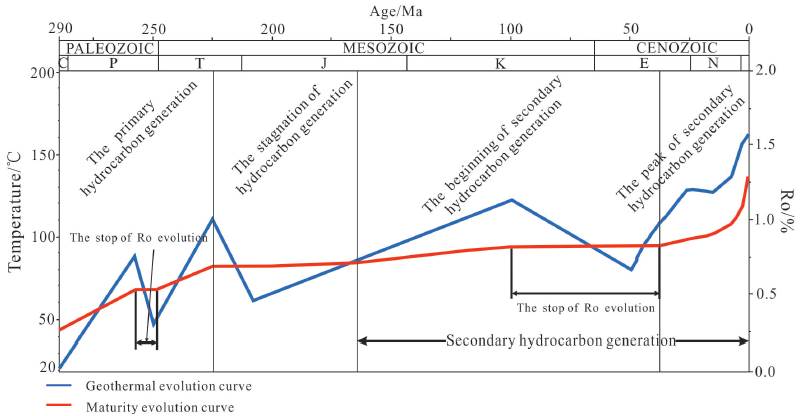
Figure 6 Geothermal and VRo curves depict the evolutionary history of C-P coals in the Jiyang Sub-basin. I: primary hydrocarbon generation, II: stagnation of hydrocarbon generation, III: initiation of secondary hydrocarbon generation, and IV: peak of secondary hydrocarbon generation.
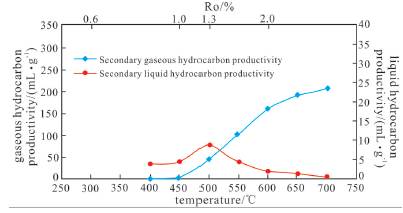
Figure 7 Secondary hydrocarbon productivity of C-P coals from the Jiyang Sub-basin obtained from laboratory simulation experiments (Jin et al., 2009).
Using data for thickness (Fig. 2), burial depth (Fig. 1b), and the distribution of C-P coal-bearing sequences, it was possible to determine that C-P coals from burial depths of more than 4,000 m with a thickness of more than 600 m had the highest hydrocarbon-generating potential (Fig. 8). The largest secondary hydrocarbon intensity of each center exceeded 400 kt/km2.
The maturity evolution history shows that VRo values were approximately 0.75% following the stagnation of primary hydrocarbon generation in the C-P coals (Fig. 6). Previous studies showed that in late Palaeozoic coals of the Bohai Bay basin, secondary hydrocarbon generation included a delay phenomenon (Guan et al., 2003). This delay in VRo with respect to the initial VRo (i.e., the abscissa) for secondary hydrocarbon generation (Fig. 9) conforms to a flexural change rule. In other words, the degree of delay (approximately 0.1% in this case) will be lowest when VRo is about 0.9%. Data showed that during the Yanshan epoch, the delay in the primary maturity of secondary hydrocarbon generation was approximately 0.15%, while during the Himalayan epoch, this delay was approximately 0.1% (Fig. 9) (Qin et al., 2000; Zhang et al., 2002). Thus, notable secondary hydrocarbon-generating maturities (i.e., generated hydrocarbon quantities of more than 10 mg/g) within the Yanshan and Himalayan epochs were 0.9% and 1.1%, respectively.
Threshold depths and favorable areas for secondary hydrocarbon generation in coal-measure source rocks
According to the thermal history, delay effect, and hydrocarbon-generating maturity, the threshold depth for secondary hydrocarbon generation was approximately 2,100 m at a VRo value of 0.9%. These C-P coal-bearing sequences experienced uplift during the middle Cretaceous (i.e., the Late Yanshan epoch), resulting in stagnation of secondary hydrocarbon generation. The source rocks could not reach peak hydrocarbon generation (i.e., VRo value of 1.0%) and thus areas of hydrocarbon generation were limited, for example, to the depocenter during the Yanshan epoch. In contrast, during the Cenozoic, an increase in burial depth resulted in an increase in VRo for C-P coals from 0.9% to 1.5%. A significant transition in the VRo evolution history for C-P coals was observed in the Cenozoic (Fig. 6). This curve suggests that the rate of VRo evolution increased during this stage. When VRo reached 1.1%, the source rocks entered the peak stage of hydrocarbon generation, corresponding to a threshold depth of 3200 m.
By combining the results shown in Figs. 1b, 2, and 5, favorable areas for secondary hydrocarbon generation in the Jiyang Sub-basin could be identified (Fig. 10) on the basis of burial depth (i.e., exceeding 4,000 m), residual thicknesses (i.e., exceeding 400 m), and VRo value (i.e., exceeding 1.0%). According to these data, favorable areas include the Chexi region in the Chezhen depression, the Gubei-Luojia region in the Zhanhua depression, the Yangxin area, the southern slope in the Huimin depression, and the area southwest of the Dongxing depression. This subdivision of favorable areas for secondary hydrocarbon generation is also indicative of current hydrocarbon-generating intensity (i.e. exceeding 200 kt/km2; Fig. 8). High potential for secondary hydrocarbon generation provides the conditions necessary for the formation of gas reservoirs, and these areas may be suitable for coal-derived gas exploration and development.
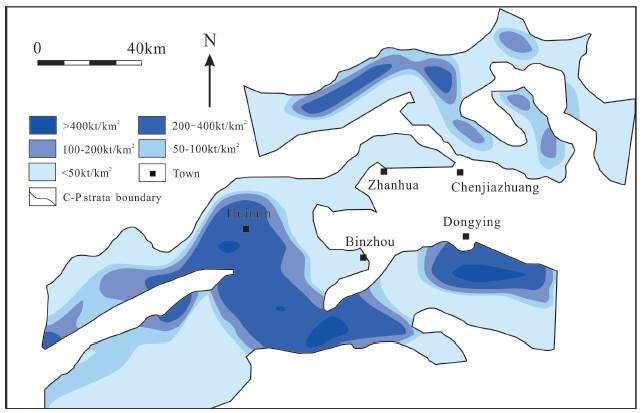
Figure 8 Present-day hydrocarbon-generating intensities of C-P coals in the Jiyang Sub-basin (unpublished data from GSRISPA).
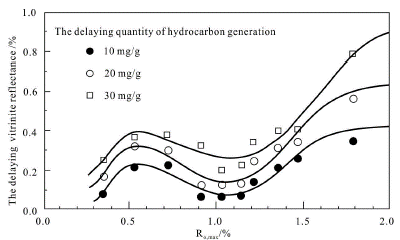
Figure 9 Quantitative plots depicting delayed secondary hydrocarbon generation according to Qin et al. (2000).
Relationship between burial history and the evolution of hydrocarbon generation
During the Indosinian epoch, initial burial depths were as deep as 1,500 m and were controlled by deposition, while the temperature reached almost 90°C, and VRo values exceeded 0.6%. At this time, the VRo value passed the threshold for hydrocarbon generation and increased further to approximately 0.75% as burial depths increased to approximately 2,500 m. During the late Indosinian and early Yanshan epochs, C-P coal-bearing sequences were uplifted until they reached the surface, while the temperature decreased sharply. This resulted in stagnation of hydrocarbon generation and constant VRo values at around 0.75%. Finally, during the late Yanshan epoch, burial depths did not attain the maxima that occurred during the Indosinian epoch, but source rock evolution continued and VRo values approached 0.9%. This period of evolution was the result of a magmatic thermal anomaly that occurred during the Yanshan epoch. Because the overall paleogeothermal gradient during the Mesozoic ranged between 4.33°C/100 m and 4.67°C/100 m (Li et al., 2001), these strata underwent a long period (>100 Ma) of heating. This contributed to an increase in VRo values by approximately 0.15%. During the Himalayan epoch, the basin subsided substantially, which resulted in burial depths exceeding 3,500 m, rapid subsidence rates of 40 m/Ma, and an increase in temperature from 105°C to 165°C. This rapid temperature increase resulted in a corresponding increase in the rate of VR evolution, and VR values increased to their current level of 1.3%. In the center of the Zhuahua and Dongying depressions, the evolution of hydrocarbon generation reached the post-mature stage, but VRo values were generally lower than expected at other areas in the Jiyang Sub-basin. The strata also attained a relatively high temperature; however, the short duration of heating suggests that the VRo value of the source rocks was not as high as it would have been after a longer time at this temperature.
On the basis of the discussion presented above, we observed that burial history determines the evolution of temperature and controls the evolution of hydrocarbon generation in source rocks. The hydrocarbon maturity history was consistent with the burial history. Of the four stages for burial depth and VRo evolution (Table 3), the maturity evolution of the second stage was unchanged and characterized by a decrease in burial depth. Therefore, burial depth and VRo values did not correlate well during this stage. Data showed that the change in VRo with respect to burial depth was 0.24%/km during the first stage and 0.125%/km during the third stage. The third stage could therefore be defined as a recovery stage, characterized by abnormally high temperatures. The fourth stage was characterized by a strong subsidence process and heating conditions but had a relatively low rate of VRo change compared with the first stage. The VRo rate decreased to 0.22 %/km during this period due to the short heating duration. The fourth stage had the shortest time but the highest VRo/time (1.0%/100 Ma), suggesting that the subsidence rate played an important role in the maturation of organic matter.
Table 3 Relationship between burial depth and VRo. The stages for VRo values are derived from the division of burial history
Stage | 1 | 2 | 3 | 4 |
---|---|---|---|---|
Time | 290-225 Ma | 225-200 Ma | 200-40 Ma | 40 Ma-present |
Epoch | Indosinian | Early Yanshan | Middle-late Yanshan | Himalayan |
Burial depth range (m) | 400-2300 | 2300-800 | 800-2000 | 2000-3800 |
Subsidence rate (m/Ma) | 29.3 | Denudation | 7.5 | 45 |
Temperature range (°C) | 35-115 | 115-55 | 55-110 | 110-165 |
VRo range (%) | 0.3-0.75 | 0.75 | 0.75-0.9 | 0.9-1.3 |
VRo/burial depth (%/km) | 0.24 | 0 | 0.125 | 0.22 |
VRo/time (%/100 Ma) | 0.69 | 0 | 0.09 | 1.0 |
VRo /burial depth = (difference between the maximum and minimum values for VR )/(difference between the maximum and minimum values for burial depth).
Maximum subsidence rates were discerned in the Himalayan epoch, while maximum VRo/burial depths occurred in the Indosinian epoch. This suggests that simple growth in the subsidence rate in the absence of a temperature increase could not significantly promote hydrocarbon generation. Evaluation of the evolutionary progress of C-P coals in the Jiyang Sub-basin indicated that both the subsidence rate and temperature controlled the rate of VRo change (Fig. 11). During the middle to late Yanshan epoch, because C-P coals were heated by magmatic hydrothermal activity, the rate of change in VRo was relatively high (Fig. 11a). This result suggests that temperature played a direct role in the VRo change, and the temperature increase caused by subsidence had a weaker effect on the VRo change compared with magmatic activity. These rocks experienced identical temperature changes (55°C) during the middle to late Yanshan and the Himalayan epochs. However, temperatures during the middle to late Yanshan epoch were controlled by a magmatic thermal anomaly, while temperatures during the Himalayan epoch were controlled by burial depth. As shown in Fig. 11b, when temperature effects were removed, the rate of VRo change was strongly positively correlated with the subsidence rate. This result suggests that the subsidence rate exerted a positive impact on the change in VRo. The evolution of organic matter and increases in VRo are controlled by temperature and time (Ritter, 1984; Ismail and Shamsuddin, 1991). A high subsidence rate indicates a greater depth during the same period, and a greater depth indicates a higher temperature. A higher temperature during organic matter evolution can improve maturity at a faster rate. Thus, a higher subsidence rate contributed to a stronger increase in organic matter maturation.
A previous study also suggested that a higher subsidence rate results in higher organic matter maturation and stronger hydrocarbon generation over a short time (Lei et al., 2014).
The calculated results show that although the maximum change in temperature occurred during the Indosinian epoch, the highest increase in VRo was during the Himalayan epoch (1.0%/100 Ma), suggesting a positive effect of the subsidence rate. Because the subsidence rate remained relatively high between the late Palaeozoic and the present time in the Jiyang Sub-basin, C-P coals were subjected to a relatively greater burial depth over a short time. This resulted in greater heating compared with a normal or low subsidence rate. The resulting elevated temperatures accelerated the maturation of organic matter, which eventually led to greater VRo change over the course of hydrocarbon generation. During the middle to late Yanshan period, the temperature did not return to the maximum temperature of the Indosinian period, but rather, VRo increased by 0.15% at a low rate of increase (0.09%/100 Ma). This suggests that the initiating temperature for secondary hydrocarbon generation was lower than the highest temperature during primary hydrocarbon generation. However, a significant subsidence in the Himalayan epoch caused a higher increase in temperature and VRo. This indicates that a higher temperature accelerated the evolution of hydrocarbon generation. A higher subsidence rate contributed to a stronger heating effect on the organic matter present and this increase in temperature enhanced the evolution of hydrocarbon generation. Therefore, both the subsidence rate and temperature played important roles in the evolution of hydrocarbon generation.
Conclusion
(1) The burial, thermal, and maturity histories of C-P coal measures in the Jiyang Sub-basin were reconstructed using PetroMod modeling. The results show that the C-P coals experienced four stages of hydrocarbon generation: primary hydrocarbon generation, stagnation, initiation of secondary hydrocarbon generation, and peak secondary hydrocarbon generation.
(2) The secondary hydrocarbon generation of C-P coals could be characterized in terms of discontinuous hydrocarbon generation and geographical differences in VRo distribution. The process of secondary hydrocarbon generation could be divided into two phases: the Yanshan stage and the Himalayan stage. Threshold maturities for secondary hydrocarbon generation within these stages were 0.9% and 1.1%, respectively, which were both impacted by a delay effect.
(3) The threshold depth for initial secondary hydrocarbon generation was 2,100 m during the Yanshan epoch, while peak hydrocarbon generation occurred at a depth of 3,200 m during the Himalayan epoch. Observations showed that favorable areas for secondary hydrocarbon generation consist of the Chexi, Gubei-Luojia, and Yangxin areas, as well as the southern slope of the Huimin depression and the southwestern section of the Dongying depression.
(4) The VRo change rate associated with burial depth in C-P coals in the Jiyang Sub-basin was 0.24%/km during the Indosinian epoch, 0.125%/km during the middle to late Yanshan epoch, and 0.22%/km during the Himalayan epoch. A higher subsidence rate contributed to a stronger heating effect on the organic matter and this increase in temperature enhanced the evolution of hydrocarbon generation.