Introduction
Complexity of fracture systems may vary broadly in basalts. These rocks have different fracture patterns depending on the morphology and thickness of the lava flow (DeGraff & Aydin, 1993; Thordarson & Self, 1998; Bondre et al., 2004; Vye-Brown et al., 2013). In addition, a significant number of fractures of tectonic origin may be present in these rocks (Scheidegger, 1978; Puffer & Student, 1992; Fernandes et al., 2016).
Abrasion is the predominant erosion process in rocks with few fractures, while rocks with submetric spacing of fractures favor plucking. (Hancock et al., 1998; Whipple et al., 2000a). Abrasion is produced by the removal of particles from the rock surface by the impact of sediment carried by the flow, while plucking is a process of extracting blocks bounded by joints, only by hydraulic forces. Thus, cooling joints in basaltic riverbeds typically provide an erosive response involving plucking (Whipple et al., 2000b; Lima & Binda, 2013; Lima & Flores, 2017). Although differences in fracture style are not significant for long-term river erosion through plucking, these differences can influence bed morphology on the scale of reaches.
Two features of fracture systems are critical in determining differences in erosive intensity (Scott & Wohl, 2019). The first is fracture density, which determines the size of rock blocks susceptible to plucking and macroabrasion (Whipple et al., 2000b). The second is fracture direction, which can favor the plucking process depending on its relationship with the channel flow direction (Dubinski & Wohl, 2013).
The fracture density has been recognized as a determining variable of the type of erosion (Hancock et al., 1998; Whipple et al., 2000a). However, the fracture density has not been sufficiently studied as a factor in the variation in erosion intensity within the plucking domain. This gap in the literature is even greater for basalts, partly because of a lack of detailed knowledge of the plucking erosion mechanics in complex fracture systems. The effect of the fracture direction on river erosion has been further studied in terms of the preferential direction imposed on drainage channels (e.g., Pelletier et al., 2009). Regarding the effect on the erosion intensity, some studies (e.g., Miller, 1991; Dubinski & Wohl, 2013) have shown that the fracture direction determines the plucking efficiency, and greater exposure of a fracture face to the flow facilitates block sliding.
In this study, the effect of variability in fracture style of basalts on plucking erosion in a bedrock river reach was analyzed in detail. The studied reach presents fracture variations due to the internal zones of common basaltic flow from the Paraná Volcanic Province. Moreover, the area is influenced by tectonic fractures imprinted over cooling joints. Thus, it was possible to evaluate the effect of density variation and fracture direction on plucking behavior in a relatively small area. These two factors that in part control the relationship between river erosion processes and volcanic rocks, will be important to understand other areas because continental flood basalt provinces worldwide are similar (Jerran & Widdowson, 2005).
Study Area
The studied river is in the Paraná Volcanic Province (South America), which is one of the largest igneous provinces of continental basalts on Earth (Frank et al., 2009). This volcanism was dated between 137 and 127 Ma (Turner et al., 1994) and about 90% of the rocks are basalts and andesites (Piccirillo et al., 1988; Lopes, 2008).
The area is a bedrock reach of the Bananas River, which is a tributary of the Jordão River (Figure 1), which in turn is a tributary of the right branch of the Iguaçu River in southern Brazil. This bedrock reach is a knickzone, that is, a reach with a higher slope situated between reaches with lower relative slopes comprising a sequence of small knickpoints and rapids (Figure 2). The bedrock reach has a variable width of 45 to 90 m and a length of 400 m. This area was selected because of the excellent exposure of bedrock, especially during the winter low flows.
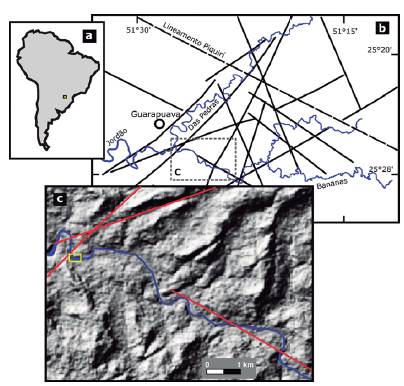
Figure 1 (a) Location of the study area in the regional context and (b) in relation to the main drainage and main structural lineaments, according to the Serviço Geológico do Paraná (2013). (c) Shaded relief map with some lineaments (red lines) and the study area (yellow box).
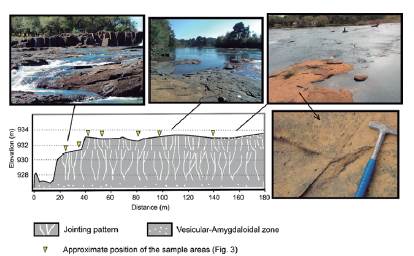
Figure 2 Longitudinal profile of the studied reach and selected field views. Jointing pattern are not to scale. Note that the positions of the sample areas are approximate because the profile was outlined more to the right side of the channel while the sample areas were located near the left side.
The studied reach is approximately 80 m upstream from an intersection with a structural lineament (N45E), which is approximately 18 km long (Figure 1c). Another lineament (N70E) is situated about 500 m downstream of the study area. Considering that these lineaments may represent fault lines, fracturing of the Bananas River bedrock may have been affected by the stress associated with these faults or other regional structures (Figure 1b). One possible effect of this stress is an increase in the fracture density of the basalts near these lineaments.
The basaltic flows in the region surrounding the study area are of the simple type (Walker, 1971), and are characterized by large lateral extensions and large thicknesses (30 m on average; Nardy, 1995). The flows have thick massive basalt cores with relatively thin zones at the top (0.3-3.0 m) consisted of amygdaloidal basalt filled with quartz, chalcedony, and zeolites (Arioli et al., 2008; Serviço Geológico do Paraná, 2013). Locally, in the Bananas reach, the outcrops share these regional morphological characteristics. The upper part is a vesicular-amygdaloidal zone with approximately 3 m thick. The lower part consists of massive basalt with an outcrop thickness of approximately 6 m (Figure 2). The flow structure is similar to that described by Thordarson & Self (1998) for what they called proximal sections in the Roza Member of the Columbia River Basalt Group. Unlike the pattern widely described in the literature, in which hexagonal columns are well developed, the basalts in this area are not columnar with well-defined geometry. In the core of the lava flow, the main joints have well-developed curvilinear faces that produce columns of variable horizontal dimensions, with maximum value less than 1 m. The curved joints tend to be tapered at the base. The main joints intersect with other joints, many of which are short, while others are longer and straighter.
According to Piccirillo et al. (1988), the central region of the Paraná Volcanic Province (and study area) occupies a transitional zone between southern (basalts TiO2 < 0.2%) and northern regions (basalts TiO2 > 0.2%) based on geochemical and petrographical criteria. The rocks exposed in the riverbed and surrounding area are dark gray with mesoscopic fine-grained phaneritic to aphanitic texture. The low density of vesicles viewed in the exposed riverbed (Figure 2) is related to the base of that basaltic flow zone.
Methods and Materials
To evaluate the influence of fracture characteristics on river erosion in the studied area, the survey was designed to measure the density and direction of fractures along the bedrock reach. These two objectives required two approaches to data collection, one based on field observations and the other on remote sensing.
In the field, seven sample areas (Figure 3) were photographed vertically with a 14.3 megapixel digital camera at an average height of 1.6 m. Consequently, the average diameter of the circular areas was 1.34 m. The photographs were digitally analyzed to enhance the fractures and measure the directions and densities. Although the bedrock reach is approximately 400 m long, the sample areas were only 120 m long (Figure 3). Despite the low flow, river water and sediments covered the lower portions of the bed, so field photographs could not be made in this part of the reach.
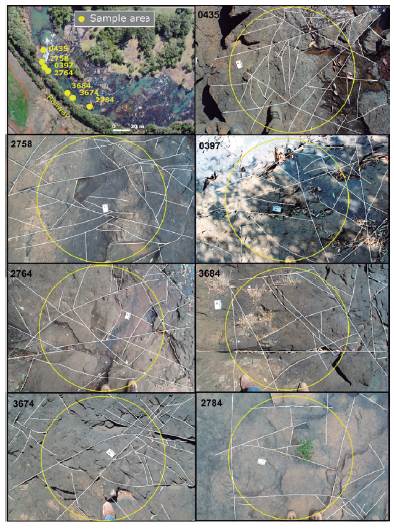
Figure 3 Locations of riverbed sample areas (first box) and field photograph with digitally enhanced fractures (white lines), the circle (yellow) was used as a scanline for the calculations (see text for details). The scale card on the riverbed is 8 cm long.
Fracture density was calculated using the circular scanline technique (Mauldon et al., 2001), which was adapted for use in field photography. In each photograph, a circle was drawn with an average diameter of 1.34 m, along which the number of intersections with fractures was counted (Figure 3). The density (D) was obtained using:
where n is the number of fractures intersected by the circle, and R is the radius of the circle.
Data from the field sampling areas were first used to determine the fracture directions. Many long fractures with significant erosion control were observed and could not be sampled, because the spacing between them, in many cases, exceeded the size of the circular sample areas. Thus, the bedrock reach was imaged with a remote piloted aircraft (RPA, Phantom 4 Advanced) carrying a camera with a 20-megapixel CMOS sensor. Images were taken at an altitude of 99.8 m and a resolution of 2.46 cm/pixel. The 188 photographs obtained were digitally processed using Agisoft PhotoScan software to generate an overall orthophoto of the area (Figure 3). The orthophoto was used to map the main fractures in the bed. This procedure avoids the inclusion of data on small fractures, i.e., cooling joints or even tectonic fractures, which were surveyed with circular scanlines.
Some tectonic fractures could be identified in the field because they are relatively long, straight and intersect the cooling joints and sometimes cause displacement. In general, it is difficult to differentiate among tectonic and non-tectonic fractures in the field, particularly small fractures, and the irregular pattern of cooling joints in the lava flow type present in the study area. In this study, differentiating the nature of minor fractures was not considered because their effect on erosion was assessed indirectly from fracture density. Instead indications of tectonic participation in fracturing of volcanic rocks can be better evaluated from the preferential directions expressed in the fracture system, which we do following the methods of other authors (Scheidegger,1978; Rogers et al., 1996).
The survey data were analyzed to evaluate the relationships between (1) fracture direction and direction of the main erosion axes, (2) channel flow direction and direction of main erosion axes, (3) variability in fracture density and possible controls, and (4) fracture density and erosional characteristics (typology and intensity). To analyze the first relationship, it was assumed, based on field observations and aerial image examination, that erosion produced step-pool features in which the main axes are located where the bed is deeper. Many pools have an elongated shape, which served to define the erosion axes. The general directions of these erosion axes were traced using images from RPA. To analyze the relationship between fracture density and erosional characteristics, we qualitatively evaluated the spatial distribution of the type and intensity of erosion processes (abrasion and plucking) based on field observations and RPA imaging.
Results
Fracture Directions
The main fracture directions differed among sample area (Figure 4) and a more detailed analysis revealed some peculiarities. First, the lineament directions (N45E and N70E) did not correspond to the main direction offractures in any of the sample areas, except in area 0435 where the direction N50-60E is remarkable. Another peculiarity involved fractures with main directions close to North (~20°), which appeared in four areas. Directions close to East-West occurred in three of the seven areas. In addition to the presence of preferential maxima in most areas the fractures exhibit a broad range of directions, except for the upstream area (2784), in which the number of fractures was smaller and concentrated in two distinct directions.
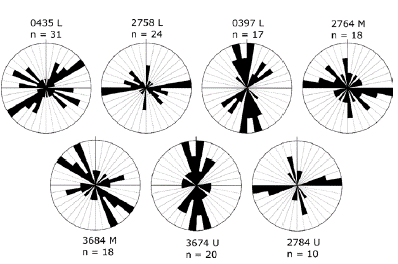
Figure 4 Fracture directions in the sample areas of the studied reach. The four-digit numbers identify the sample areas (see Figure 3); letters L, M and U indicate the Lower, Middle and Upper segments of the reach, respectively, according the river flow direction (see Figure 6); and (n) is the number of fractures. The diagrams are arranged sequentially from downstream to upstream.
Unlike field sampling, the high-resolution RPA images allowed us to observe the major fractures (i.e., the longest ones) in the bed (Figure 5). The directions of these major fractures have four maxima, one oriented NW (5070) and three oriented NE (10-20, 50-70, and 80-90). If only the main and secondary maxima of the circular samples (Figure 3) are observed, there is some level of correspondence with the RPA survey. All circular sample areas had some maxima that corresponded to the RPA survey. Areas 2758 and 2764 presented the highest correspondences with three maxima each one.
Erosion Axes versus Fracture Direction
The main erosion axes of the studied reach have two well-marked maxima at N00-10E and N80-90E (Figure 6). The N80-90E direction coincides with the major maximum of the bed fracture directions (Figure 5). By contrast, the directional axes at N00-10E are close to another directional maximum of fractures oriented N10-20E. The number of erosion axes with a NW direction is lower than the number with NE directions; however, there is a small concentration around the N50-70W direction, which coincides with one of the main fracture directions (Figure 5).
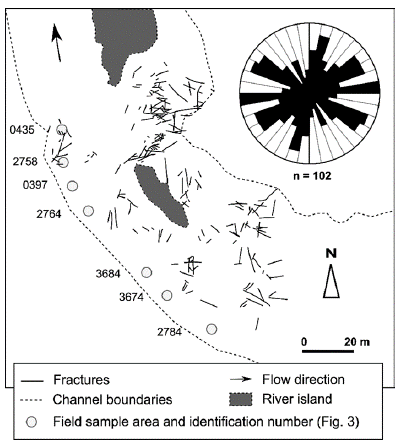
Figure 5 Fractures in the bedrock reach, according to the RPA image survey and the respective rose diagram.
In the studied reach, the river channel develops a wide curve, changing the flow direction, which in turn can influence which fracture directions facilitate erosion. To verify this hypothesis, the direction of the erosion axes was evaluated separately in three channel segments (Figure 6). In fact, each segment has a different direction distribution. In the upper segment, erosion axes with a direction of N60-70E are predominant. In the middle segment, the main direction is N80-90E, whereas in the lower segment, the main direction is N10-20E. The lower segment is distinguished from the others by the almost absolute predominance of a single erosion axis direction. Each of these major directions of the erosion axes coincides with the major directions of bed fracture (Figure 5). In the upper segment, even the minor directions of the erosion axes correspond to major fracture directions.
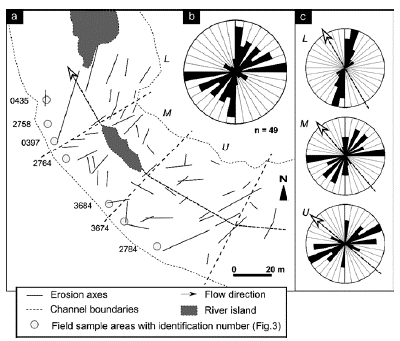
Figure 6 (a) Main erosion axes in the studied river reach, (b) general distribution of directions, and (c) distribution by channel segments (L: Lower, M: Middle, U: Upper) according to the change in flow direction, indicated by an arrow in a dashed-line.
The overall channel flow direction changes in each segment of the reach. It ranges from 50 NW in the upper segment to 40 NW in the middle segment and finally 30 NW in the lower segment. The smallest angle between the general direction of flow in the channel and the main direction of the erosion axes in each segment is 40-60°. In the upper segment, where three erosion axes dominate, the angle with respect to the main direction is 60°, that with respect to the secondary direction is 40°, and that with respect to the third direction is 50°. Although there are differences in the angular relationship between erosion axes and channel flow direction, there is a consistency in the values around 50°.
As noted earlier, some fracture directions surveyed in the circular areas match the maxima obtained from the RPA (Figures 4 and 5), while others do not. Unmatched directions are related to minor and multidirectional fractures, which may be tectonic or cooling joints. These fractures are not related to the directions of erosion axes but contribute to overall erosion by plucking. An example of these distinct roles is evidenced in sample area 3684 (Figure 7). In this area, a conjugate system of tectonic fractures is well-defined. The N85W main fracture act as a control on the erosion direction. The river flow direction at this site is approximately N40W, which result in an angle of 45° to the main fracture. Minor and long joints are crossed by the main fracture, producing small blocks ready for plucking. In fact, erosion by plucking produced a small step in the bed. Here and in other places in the study area the main fracture is opening and facilitates hydraulic wedging (Hancock et al., 1998), which occur as clasts are trapped in fractures, forcing displacement of blocks.
Fracture Density
Table 1 presents the fracture density calculated from measurements made in the seven sample areas. The areas appear in the order of their location along the analyzed reach, that is, from downstream to upstream (Figure 3). Data analysis reveals that fracture density tends to decrease in the upstream direction. The more upstream area (2784) is on the amygdaloidal basalt. At the other end of the analyzed transect, by contrast, the fracture density of area 0435 is three times higher than area 2784. Sample area 0435 is situated on a massive basalt and is closest (~80 m) to the lineament N45E which river crosses.
Table 1 Fracture density in Bananas River sampling areas.
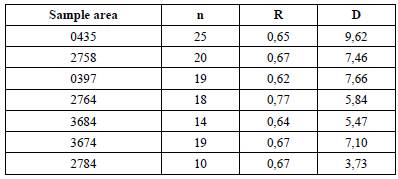
n: number of fractures that intersect the circumference of the sample area; R: sample area radius (meters); D: fracture density according Mauldon et al. (2001), D = n/4R.
Discussion
Tectonic Fractures and Cooling Joints
Basaltic rocks have many joints owing to the cooling process of lava. These joints assume various directions depending on their location in the lava flow body or even in a limited area. As shown in Figures 3 and 4, there is a wide distribution of fracture directions in the study area. However, tectonic fracturing is possibly indicated by the presence of some main directions, that agree with some authors (e.g., Rogers et al., 1996).
The presence of tectonic fractures in the study area is directly confirmed by some field observations (Figures 3 and 7); however, considering that the joint directions are distributed among several classes, fractures of tectonic origin may overprint other types of fractures because pre-existing joints may accommodate subsequent tectonic stress (Patrick Muffler et al., 1994; Grant & Kattenhorn, 2004; Moir et al., 2010).
Main Fractures and Erosion
The importance of main fractures to erosion in the studied river reach is highlighted by the strong relationship between the erosion axis directions and the main fracture directions (Figures 5 and 6). The tectonic nature of some fractures with greater pervasiveness and directional continuity makes them preferable for erosion. This also may be true for some long (3-4 m) cooling joints. The more abundant and multidirectional small joints act to control the plucking process because they limit the polyhedral blocks, but not to determine the main erosion direction (Figure 7).
Most of the main erosion axes, as defined in this study, are related to step-pool morphology zones. The erosive evolution of these zones from long fractures can be understood primarily in terms of the higher density of fractures along one direction. Over a long fracture, the number of intersections with other natural basalt joints becomes larger. In addition, tectonic fractures will often have subsidiary (pinned) joints, which increase the density of rock discontinuities along the main direction (Peacock, 2001). As a result, the blocks available for simple plucking and breakage by impacts from sediments in transit (macroabrasion, Whipple et al., 2000a) are potentially smaller than those in other bed areas and erosion is concentrated along this line (Figure 7).
These preferential linear erosion zones may eventually be interrupted owing to local variations in the channel flow direction and/or the presence of basalt joints. Especially at the intermediate levels of the thick basaltic flows of the Paraná Basin Province (Serviço Geológico do Paraná, 2013) and of the studied area, columns with metric dimensions (height and width) delimited by joints with curvilinear planes are common. The river flow can create alternative paths along these joints.
Flow and Erosion Direction
As noted earlier (Results section), the overall flow direction makes an angle of 40-60° with respect to the main direction of the erosion axes in each segment into which the reach has been divided. Furthermore, these axes directions correspond to major fracture directions. The slope of the channel has no discernible effect on this angular relationship. In the lower segment, which has a higher slope, the angle is 40°. In the middle segment, which has a smaller slope, the angle is 50°. The slope of the upper segment is between those of the other two segments; however, the main angular relationship is 60°, although the 40° and 50° relationships also occur in secondary directions. The angular relationship between the flow and fracture directions (erosion axes) within the observed range apparently depends on the variability of the channel flow hydrodynamics, the variability of the interaction between tectonic fractures and cooling joints, and the lithological characteristics.
Considering the large number of variables involved, the only possible conclusion is that the average relationship between the channel flow direction and erosion axis direction is on the order of 50°. Satellite observations of other parts of the Bananas River and other rivers in the region, which run over basalts, confirm the average angular relationship of 50° between the direction of flow and the erosion axes, especially the knickpoint face directions. Miller (1991), who studied rivers in siliciclastic and carbonate rocks, also frequently observed oblique arrangements of knickpoints generated by fractures. Fracturing is much more complex in basalts of the study area than in that sedimentary rocks. The presence of more pervasive and continuous tectonic fractures seems to be essential for establishing the angular relationship with the flow direction, similar to the case in simpler fracture systems.
Why the river flow tends to have this specific angular relationship with the major fractures remains an open question. Although a definitive answer requires further investigation, it is possible to deduce that angles much smaller than 40° between the flow direction and the direction of these pervasive fractures favor the adjustment of the channel along the structures. As several surveys have shown, rivers tend to exploit directions represented by vertical fractures (e.g., Eyles et al., 1997; Pelletier et al., 2009). Flume experiments (Dubinski & Wohl, 2013; Wilkinson et al., 2018) with regular fracturing suggest that an orthogonal arrangement of fractures with respect to the flow direction favors the removal of bed blocks. Although the experimental results presented by George et al. (2015) for blocks with complex geometries indicate that the erodibility is greater when the angle with the flow is 75°, it is worth noting that this is the angle with respect to the central axis and not that with respect to the face of the block, owing to its tetragonal geometry. Correcting the angle to that with respect to the face of the block gives an approximate value of 55°, which is consistent with the average angle obtained for the Bananas River.
Fracture Density Variation
Lima & Binda (2013) found that the average fracture density in the basalts of the Das Pedras River, close to the Bananas River (Figure 1), in the reaches associated with structural lineaments, is 6.05 m/m2. The average obtained for the Bananas River study (6.7 m/m2) is similar (Table 1). Overlap between tectonic fractures and basalt joints occurs effectively, as confirmed by the presence of preferential directions (Figure 5) and field observations (Figure 7). This implies that the fracture density is increased by tectonic effects. As previously described, two major lineaments are close enough to the area to eventually contribute to the generation of fractures. However, the upstream decrease in fracture density (Table 1) is depending on the distance from the structural lineament (N45E) that the channel crosses? The maximum fracture density (9.2) appears in the zone closest to that lineament (Table 1). Sample area 2784 is farthest from the lineament and has the lowest fracture density (3.73). Contrary to what was expected if the study area is associated with a damage zone, there is no maximum direction parallel to the lineament (N45E), although it is possible for joints to form at high angles to the strike of a fault (Kattenhorn et al., 2000).
Fracture density in basalts varies depending on the internal lava flow structure. In fact, area 2784 is on an amygdaloidal zone, whereas the others are on massive basalt. For amygdaloidal zones, fracture densities equal to or greater than those of massive zones have been reported (Faust, 1977; Thordarson & Self, 1998), although lower densities are also possible (Lima & Binda, 2013).
One aspect that favors the damage zone hypothesis is the gradual decrease in fracture densities upstream. Transitions in the pattern of fractures between internal zones of the lava flows are usually abrupt. Moreover, the absence of fractures parallel to the lineament could be masked by the cooling joints which served to accommodate subsequent tectonic stress, as described in the first section of this discussion. Further and detailed analysis will be necessary to describe the nature of the lineament and to define if there is a real relationship with the pattern of fracture density detected in the study area.
Fracture Density and Erosion
Regardless of the causative factor, could the variation in the fracture density affect the erosion processes? Theoretically, variation in fracture density can affect the type of erosion processes that occur and their intensity. Low fracture density, as found upstream in the study area, favors abrasion (Whipple et al., 2000b). The formation of potholes indicates abrasive processes. However, in the studied reach, these abrasive features appear in both upper and lower segments, regardless of the different fracture densities (9.6 and 3.7, respectively). Despite these signs of abrasion, plucking and macroabrasion are the main processes acting along the entire reach.
The increase in fracture density (Table 1), which coincides with massive basalt zone, favors plucking because it decreases the size of the rock blocks. By contrast, the decrease in fractures densities culminates in an amygdaloidal basalt zone (sample area 2784) that is less resistant and more susceptible to weathering (Lima & Binda, 2013). Low fracture density limits the plucking of blocks because they are larger. However, the high susceptibility to weathering and low rock resistance favor macroabrasion (Small et al., 2015). This is observed in the increase of the chipping features of the bed surface (sample areas 3674 and 2784, Figure 3) and in the smoothing of the reach morphology (Figure 2). While erodibility may be the same throughout the reach, bed morphology and slopes may differ.
Conclusions
The study of a bedrock fluvial reach that developed on basalts allowed us to verify the effects of spatial variability in density, typology, and direction of fractures on erosion behavior. Although it does not change the type of dominant process, which in this case is plucking, this variability modifies the intensity and the distribution of erosion in the channel, with implications for bed morphology.
The presence of preferential fracture directions attributed to tectonic activity was verified. Owing to their pervasiveness and length, some of these tectonic fractures control the main axes where bed erosion is concentrated. The small, abundant, and multi-directional basalt cooling joints control the plucking process, but do not the erosion direction.
The general direction of river flow is at an average angle of 50° with the main erosion axes, that is, with the direction of the main tectonic fractures. Although further investigation is required, available field findings and experimental data of the others authors suggest that this behavior is probably related to shear force optimization necessary to perform the plucking process.
In the studied reach, fracture density decreases progressively upstream from 9.62 to 3.73 m/m2, related to distance from a structural lineament transverse to the channel. The decrease in fracture density does not affect the dominant erosion process, i.e., plucking. However, owing to the textural change in the basalt, specifically, a decrease in rock resistance, macroabrasion is favored.