Introduction
Peat is formed by the decomposition of several plant materials in the waterlogged environments of marshes, bogs and swamps (Spedding, 1988; Zulkifley et al., 2016) and is present on all continents, from sea level to high altitudes (Joosten and Clarke, 2002). Peatlands cover an estimated area of 400 million hectares. However, peats located in tropical and subtropical regions correspond to a small portion of the total: 35.80 million hectares (Andriesse, 1988; IPS, 2008).
The typical characteristics of a tropical climate (high precipitation, high evapotranspiration and high average annual temperatures) directly affect the type of peat formed in this region. Climate has both direct (for example, via hydrology) and indirect (via the vegetation species; trees, containing woody material, are typically present in tropical regions, whereas sedges and Sphagnum moss are found in temperate regions) effects on peatlands. The temperature also influences the rate of oxidation of the material, and since tropical soils frequently have larger sesquioxide contents than soils in temperate regions, their iron and aluminum contents may be higher. The tropical climate region is defined as that located between the Tropics of Cancer and Capricorn. However, the typical characteristics of tropical peat do not necessarily coincide with the geographical limits, especially at high altitudes. Thus, tropical peatlands can be found on the entire African continent and in most of South America (notably Brazil and Uruguay), southern Florida, North Carolina and Southeast Asia (which contains the largest extension, approximately 57%, of tropical peat deposits) (Andriesse, 1988).
Peat is a material with a high organic matter content, consisting of mainly lignin, cellulose and humic substances (which include humic acids, fulvic acids and humin). The presence of functional groups (such as carboxylic acids, phenolic hydroxides and alcohols) associated with these organic compounds imparts a polar character; therefore, peat is a material with high potential for adsorbing metals and polar organic molecules (Couillard, 1994).
Due to its characteristics, peat has been studied over the past decades as a low-cost, efficient and environmentally friendly adsorbent for potentially toxic elements (PTEs) (Babel and Kurniawan, 2003; Bolan et al., 2014; Zehra et al., 2014; Leiviska et al., 2018; Lima et al., 2018). Contamination by PTEs contributes to environmental degradation. Several anthropogenic sources, such as fertilizers, mines, landfills and industrial sites, may result in potential hotspots of PTEs pollution (Orgiazzi et al., 2016; Mohamed et al., 2017). The presence of PTEs as contaminants can create significant environmental risks over time, as these metals are nondegradable and can become mobile and available to biological receptors. These elements may bioaccumulate and biomagnify to critical levels in the food chain, generating grave effects on human health as well as on flora and fauna (Siegel, 2002; Yong and Mulligan, 2003).
Pb, Zn and Cd are PTEs frequently present in areas contaminated by industrial and mining activities (Kasemodel et al., 2016; Marques et al., 2019). Pb is a toxic element that is nonessential and has the potential to accumulate in humans, affecting fundamental biochemical processes; it has the ability to imitate Ca and consequently inhibit Ca-protein interactions (ATSDR, 2007). Cd is another environmental pollutant. It is toxic to plants and organisms at smaller concentrations than Zn, Pb and copper (Cu) are (Adriano, 1986). Studies have provided evidence that Cd is a carcinogenic element and that acute exposure can lead to death in humans and animals (ATSDR, 2012; Santos et al., 2016). Zn is an essential nutrient for plants and animals and it is important for the biological functions of living tissues. However, when present in high concentrations in the environment, Zn becomes toxic to organisms (Kabata-Pendias, 2011). Chronic ingestion of Zn is also dangerous and may have negative effects on the gastrointestinal and hematological systems (WHO, 2001; ATSDR 2005).
The treatment of PTE-contaminated water and soil includes several processes, including adsorption. To date, knowledge is still limited, and a complete understanding of the mechanisms involved in adsorption has not been achieved. Although several studies have explored adsorption by peat from temperate and boreal climate zones (e.g., Coupal and Lalancette, 1976; Gosset et al., 1986; Chen et al., 1990; Brown et al., 2000, Qin et al., 2006; Kalmykova et al., 2008; Koivula et al., 2009; Bartczak et al., 2018; Egene et al., 2018; Hammi et al., 2019), there are little works using tropical peat deposits (Petroni, 2004; Balasubramanian et al., 2009; Abat et al., 2012; Lima, 2017; Santos et al., 2018; Marques et al., 2020). Nonetheless, interest in PTE adsorption by tropical peat is increasing due to its low cost and high availability. In Brazil alone, there are approximately 24,000 km2 of peatlands (WER, 2013), therefore, there are many areas still available as a natural resource. According to Franchi et al. (2006), the use of peat in countries with tropical climate is recent, increasing the interest in these areas.
The Mogi-Guaçu river basin, located in the northeast of the state of São Paulo (Brazil), and drainage area of approximately 15,000 km2, is an example of an area with extensive peatland that can be easily exploited (PERH, 2005). The Mogi-Guaçu peat deposits are found in the extensive flood plains associated with the meandering river system (Franchi et al., 2006). These peatlands originate from predominantly the remnants of grasses or, alternatively, woody plants (Franchi, 2004).
Brazil, like many other developing countries, struggles with PTE contaminations (Reis et al., 2019; Trevizani et al., 2019; Fernandes et al., 2020). As regards Mogi-Guaçu river basin, some studies have shown the presence of Pb, Cd, and Zn (among others PTE) in high concentrations in water, sediments, and living organisms; wherein, their concentrations can vary according to the period of floods (Tomazelli and Martinelli, 1999; Tomazelli, 2003; Silva, 2005; La Serra, 2015). In addition, the Mogi-Guaçu river basin Committee (SIGRH, 2020) establishes that the basin is classified as industrial, with emphasis on agribusiness (including sugarcane and orange), pulp and paper industry, slaughterhouses, among others. The agriculture is considered an important source of PTE for soil and water bodies, which can occur through fertilizers, pesticides, and soil amendments (Silva et al., 2016; Shanshan and Yanqing, 2020); however, the effluents of the pulp and paper industry stand out due to the presence of Pb, Cd, Zn and Cu in their composition (Frizzo et al., 1996). PERH (2005) confirmed the high demand of water for industrial activity in the Mogi-Guaçu river system. These pointed factors could increase the risk of contamination of the region by PTE in a short or long-term.
Some studies have shown good results regarding the adsorption of metals to Brazilian peats from various river basins in the states of Sergipe, Bahia, São Paulo, Rio de Janeiro and Santa Catarina (Santos, 1998; Lamim et al., 2001; Franchi, 2004; Petroni, 2004; Crescêncio Junior, 2008; Batista et al., 2009; Cerqueira et al., 2012; Oliveira et al., 2015; Carvalho, 2015; Marques et al., 2020). However, studies are necessary to evaluate the adsorption capacity of peat from the Mogi-Guaçu river basin, since this is a strategically located area (industrial area with high potential for Pb, Zn and Cd contamination) containing peatlands with easy access, which would facilitate the use of and reduce the acquisition costs associated with this reactive material.
Because of this lack of knowledge, the aim of this study was to comparatively assess the Pb, Zn and Cd adsorption capacities of two Brazilian peats collected in two cities from the Mogi-Guaçu river basin: Cravinhos (already commercialized in the country) and Luis Antônio. This study also intends to verify whether the adsorption results are compatible with those of other peats (including tropical and temperate ones), as well as those of other biosorbents. Studies such as this might encourage the use of locally available adsorptive materials and might also provide a promising direction for the development of low-cost and ecofriendly technologies for soil and water remediation, especially in tropical contaminated areas.
Materials and methods
Field sampling
Peat samples for this study were sourced from the Mogi-Guaçu river basin (São Paulo State, Brazil) in two different cities: Cravinhos (peat C) and Luis Antônio (peat LA) (Figure 1).
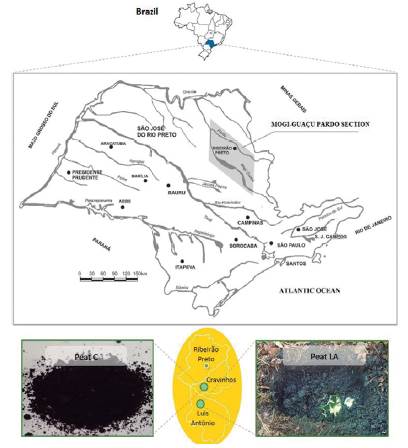
Figure 1 Locations of the peat collection sites in the Mogi-Guaçu river basin: Cravinhos city (peat C) and Luis Antônio city (peat LA). Adapted from Shimada et al. (1981).
Peat C is exploited for commercial use since its modified form (as liquid humic acid) is widely used as a fertilizer for local crops. The peat C is extracted and placed in piles in the field (Figure 2) to subsequently proceed to air drying (in covered area) and to modification (Marques et al., 2020). Peat C collection area by the company covers a few square kilometers in the municipality. For this study, the company provided samples without any treatment or chemical modification, as research intention. Peat LA was collected in November of 2017 at kilometer 40 of highway SP-255 in Luis Antônio city (21° 35'53.2 "S and 47° 57'03.3" W).
Peat sample preparation
Peat C is a commercialized peat, and after receiving it from the company, the samples were sieved through 2 mm mesh, dried at 50°C for 48 h and then homogenized. Peat LA was first dried at room temperature, homogenized in a porcelain mortar, sieved through 2 mm mesh, and subsequently dried at 50°C for 48 h. Both peats were stored at room temperature prior to analysis. Some physicochemical properties of both peats were published by Raimondi et al. (2019) and are summarized in Table 1. Table 1 also shows additional characteristics to those presented by Raimondi, such as specific surface area and pore volume, which are important properties for adsorption studies. According to Raimondi et al. (2019), both peats have similar characteristics, and the results indicate that these materials are favorable for PTE ion retention. Most of the characteristics of the two peats were similar, but peat C had a higher organic matter content, and specific surface area, besides a lower cation exchange capacity (CEC).
Metal adsorption experiments
Batch adsorption experiments were carried out to evaluate the adsorption capacities of the peats. These procedures were based on and adapted from the methodology used by Roy et al. (1992), Soares and Casagrande (2000) and ASTM D4646 (2016). One gram of the tropical peat samples was added to a Falcon tube containing 50 mL of a single metal solution. This peat/solution ratio (1/50) was used because it is the most favorable condition according to the preliminary step presented in Lima (2017) and Raimondi et al. (2019), studies that characterized both tropical peats from the Mogi-Guaçu river basin.
In the batch adsorption assays, eight initial concentrations of the three ions (Pb2+, Zn2*, and Cd2+), which ranged from 0.10 to 2.36 mmol L-1, were used. The most concentrated solutions of each metal (1.05, 2.36 and 1.45 mmol L-1 for Pb2+, Zn2* and Cd2+, respectively) were prepared by dissolving the corresponding chloride salt (PbCl2, ZnCl2 and CdCl2H2O) in deionized water. The remaining concentrations were obtained by diluting the concentration solutions in deionized water. The pH of the initial suspensions was not adjusted, and the pH of the solutions ranged from 4.0 to 6.0 depending on the peat and the PTE analyzed. The suspensions were agitated on a horizontal shaking table (Novatécnica, NT 155) at an agitation rate of approximately 120 rpm. After being agitated for 24 h (contact time determined according to ASTM D4646 (2016), the samples were centrifuged and filtered through Unifil filter paper (weight of 80 g m-2 and particle retention of 4-12 um). The Pb2*, Cd2+ and Zn2+ concentrations in the filtrates were determined by an atomic absorption spectrometer (PerkinElmer PinAAcle 900F). The instrument standard curves had three points at different concentrations (dilution of the respective PerkinElmer standards - ISO 9001 Purity Certification - in deionized water), and correlation coefficients higher than 0.995 were utilized. The solutions were diluted according to the instrument's quantification limits (0.448 mg L-1 Pb2+, 0.006 mg L-1 Zn2*, and 0.018 mg L-1 Cd2+), and the maximum value indicated by the manufacturer for a linear fit zone of the equipment (10.00 mg L-1 Pb2+, 0.75 mg L-1 Zn2+, and 1.00 mg L-1 Cd2). Batch equilibrium tests were performed in triplicate at room temperature (approximately 25°C).
The efficiency of metal removal by both peats was calculated as a percentage, as shown in Equation 1:
The ions adsorbed by a mass of material at equilibrium (q) in mmol kg-1 was calculated by Equation 2:
In Equations 1 and 2, C 0 is the initial concentration of ions in solution (mmol L-1), C is the ion concentration after adsorption equilibrium (mmol L-1), Vis the solution volume (L), and m is the mass of peat (kg).
The Langmuir (types I and II) and Freundlich (linearized forms) isotherm models were chosen to investigate the mechanism of target metal adsorption. According to Febrianto et al. (2009), both Langmuir and Freundlich isotherms are widely used to describe PTEs adsorption by biosorbents (which include peat).
The Freundlich isotherm has been used to interpret adsorption onto heterogeneous surfaces or surface sites with different affinities (Bulgariu et al. 2011, Freitas et al. 2018). The Freundlich isotherm is expressed in Equation 3:
The linearized form of the Freundlich isotherm is expressed in Equation 4:
The Langmuir isotherm is based on monolayer adsorption onto homogeneous surfaces and can be used to estimate the maximum adsorption capacity of the surface (Langmuir, 1997). This isotherm is expressed in Equation 5:
The linearized forms of the Langmuir isotherm are expressed in Equation 6 for Langmuir I and Equation 7 for Langmuir II:
In Equations 3 to 7, K f is the Freundlich equilibrium constant (L kg-1), n is the Freundlich exponential coefficient (dimensionless), q m is the adsorption capacity or maximum amount of ions or molecules to be absorbed (mmol kg-1), and K L is the Langmuir adsorption constant related to the binding energy (L mmol-1).
The separation factor (R L ) is a dimensionless constant calculated to predict the feasibility of the Langmuir isotherm (Foo and Hameed, 2010) and is defined in Equation 8:
The adsorption process can be described as a function of RL as follows: RL> 1: Unfavorable; R L = 1: Linear; 0<RL<1: Favorable; RL=0: Irreversible
Additionally, the change in free energy (ΔG) was evaluated from the Langmuir constant. Thermodynamic parameters are often used in environmental engineering to determine whether the process will occur spontaneously (Ho, 2006). The ΔG calculation is presented in Equation 9.
In Equation 9, R is the universal gas constant (8.314 J mol-1 K-1), and T is the absolute temperature (K). The temperature in the experiments was 25°C.
Results and discussion
In the batch adsorption experiments, the adsorption percentage of both peats was calculated as a function of the initial concentration, and the results are shown in Figure 3. The ion adsorption on peat occurred in single-solution systems. The removal percentage of the three ions was dependent on the initial concentration, and higher removal occurred at lower concentrations, with maximum removal reached at the lowest concentration (for example, 100% Pb, 81.4% Zn and 90.8% Cd for peat C).
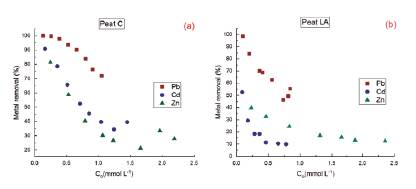
Figure 3 Percentage of metal ion removal versus the initial concentration of Pb2+, Cd2+ and Zn2+ for two natural Brazilian peats: (a) Peat C, (b) Peat LA
Peat C had a higher efficiency than peat LA, and the difference in metal ion removal can be seen in Figure 3. Both peats adsorbed more Pb than Cd and Zn, showing the preference of peat for this cation. In peat C (Figure 3a), the Pb adsorption was above 70% at all concentrations (from 100.0 to 71.9%), while in LA (Figure 3b), the Pb removal ranged from 98.6 to 46.3%. For peat C, the removal order was Pb2+ > Cd2+ ≥ Zn2+. The removal efficiency was very similar for Zn and Cd (81.4 to 27.8% Zn and 90.8 to 34.4% Cd) but was slightly higher for Cd. For peat LA, the removal efficiency followed an order of Pb2+ > Zn2+ > Cd2+, with Zn removal ranging from 39.6 to 12.5% and Cd removal from 52.6 to 9.8%. Figure 3b shows that Cd removal achieved higher percentage (52.6%) over Zn (39.6%) at the lowest initial concentration, however, its removal was generally lower. Therefore, following the order of Pb2+ > Zn2+ > Cd2+.
According to Qin et al. (2006), adsorption affinity is frequently correlated with electronegativity and softness. The preference of peat for Pb2+ can be explained, for example, by the theory of hard and soft acids and bases (HSAB) (Pearson, 1968). According to this theory, "C class cations", such as Pb2+, form stronger complexes with fulvic and humic acids in peat than do "B class ions" (Zn2+ and Cd2+) (Kalmykova et al., 2008). When considering electronegativity, the more electronegative the element is, the more strongly it is attached to the surface (McKay and Porter, 1997). Pb has the maximum adsorption capacity and the greatest electronegativity (2.33) (Table 2). Nonetheless, the order of Cd (1.69) and Zn (1.65) held true for only peat C. However, it is important that the electronegativity of Cd and Zn are similar, as are the removal efficiency results for both metals in the peats. Regarding the order Zn2+ > Cd2+ for peat LA, Liu et al. (2009) reported that the greater ionic radius of Cd may interfere with its access to the adsorbent pores, even though it has a higher affinity for binding sites. In fact, the larger pore volume of peat C (0.025 cm3 g-1) could allow greater access to Cd ions than the LA peat pore volume (0.014 cm3 g-1) could allow.
The superior efficiency and higher maximum adsorption capacity of peat C in the removal of all three tested ions from solution could be explained by its specific surface area (3.45 m2 g-1 for C and 2.04 m2 g-1 for LA - Table 1) as well as its higher organic matter content (520.4 g kg-1 for peat C and 510.06 g kg-1 for peat LA - Table 1). These characteristics may favor the performance of specific adsorption mechanisms. The higher efficiency of C may also be associated with the functional groups present on the surface of this peat.
The pH variation during the batch equilibrium test was also verified (Figure 4). In Figure 4, the "solution pH" was considered the pH of the metallic ion solution (pH at each initial concentration), "initial pH" was the pH after contact between the peat and metallic ion solution, and "final pH" was the pH after 24 h of the experiment. Batch equilibrium tests were performed without buffering the pH because the resulting initial pH of all samples (between 4 and 6 - Figure 4) was within the range considered ideal for the adsorption of metal ions onto peat and soils, between approximately 4 and 8 (Couillard, 1994; Petroni et al., 2000; Qin et al., 2006; Lim and Lee, 2015). Peats generally have a pH of approximately 4.0 due to the humic acids present, and their structures degrade at pH values higher than 9.0 and below 3.0, decreasing their chelation capacity. In addition, at low pH values, the high concentration of H+ in solution competes with metallic ions in ion exchange reactions, and at high pH values, the formation of metal oxide precipitates is the main active retention mechanism (Couillard, 1994).
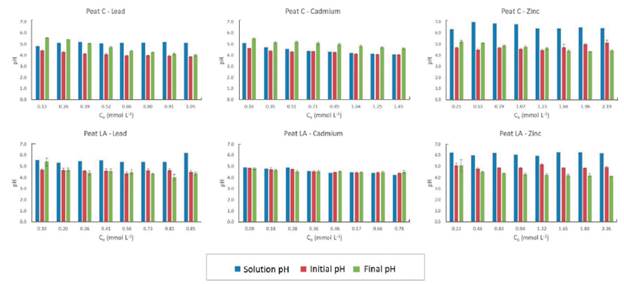
Figure 4 The pH variation variation during the batch adsorption tests of Pb, Cd and Zn ions onto two tropical peats (C - Cravinhos; LA - Luis Antonio)
As shown in Figure 4, in general, the "initial pH" tended to decrease in relation to the "solution pH". The "initial pH" resulted from an equilibrium between the "solution pH" and the pH of the material. This decrease compared to the "solution pH" is mainly due to the acidic properties of the carboxylic and phenolic functional groups present in peat (Bloom and Mcbride, 1979; Sharma et al., 2011). The "initial pH" of peat C was lower than the "initial pH" of LA (comparing the same metal - Figure 4). As an example, the "initial pH" of Pb for C ranged from 4.4 to 3.9 as the initial concentration increased, while in LA, this variation was from 4.7 to 4.5. This slight difference in the "initial pH" of the peats may have resulted from the pH of each peat, because the pH of pHH2O (measured in water) for C was 5.1 and that for LA was 5.9 (according to Raimondi et al., 2019). The difference in "initial pH" may have contributed to the higher efficiency of peat C. pH is one of the main parameters that influences adsorption processes, and at lower pH values, Pb, Zn and Cd tend to be ionized and, therefore, more soluble and available for adsorption. Therefore, the higher efficiency of peat C than of LA may be a result of their properties (such as the specific surface area, organic matter content and surface functional groups, as previously mentioned) and the lower pH values under the initial test conditions.
For both peats and for the three metals analyzed, the "final pH" of the samples (pH after 24 h of the experiment) was between 4 and 6. Koivula et al. (2009) emphasized that the final pH is governed by both the initial pH and the ions present in solution. Their research verified that the final pH decreases as the initial concentration increases and suggested that this occurs due to the ion exchange principle. According to the ion exchange reactions, with the increase in the number of adsorbed ions on peat, a greater number of H+ ions are released in solution, decreasing the pH (Lima et al., 2018). A decrease in the final pH as a function of the increase in the initial concentration can be observed in Figure 4 for both peats, except for Cd adsorption by peat LA, where the pH remained constant throughout the test as a function of the characteristics of this material, with stable ion exchange.
The experimental data were also fitted to isotherm models to understand the adsorption behavior of the Pb2+, Cd2+ and Zn2+ ions onto two tropical peats.
Adsorption isotherms describe the distribution of metal ions between the phases of the solid peat and the aqueous solution and are important elements for obtaining valuable information about the mechanism and nature of the adsorption process (Sposito, 2004; Balan et al., 2008).
The isotherm models fitted to the experimental data were Freundlich and Langmuir (linearized form I and II), and Table 3 provides the parameters of the adsorption models for the three metal ions and their correlation coefficients (R2). The equilibrium adsorption isotherm and fitting of the models to the experimental data are shown in Figures 6, 7 and 8 for Pb2+, Zn2+, and Cd2+, respectively.
Table 3 Isotherm parameters for Pb2+, Cd2+ and Zn2+ adsorption by two natural tropical peats (peat from Cravinhos - peat C - and peat from Luis Antonio - peat LA)
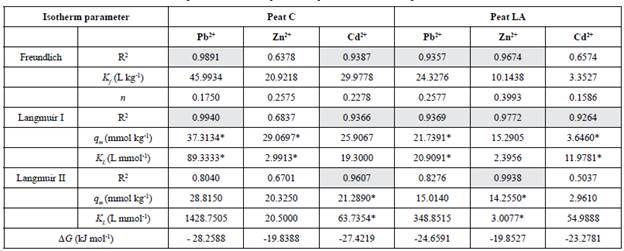
R2 - Correlation coefficients; K f - Freundlich equilibrium constant (L kg1); n - Freundlich exponential coefficient (dimensionless); q m - Adsorption capacity (mmol kg1); K L - Langmuir adsorption constant (L mmol1); AG - Change in free energy (kJ mol1); * represents values considered from the best fit of the Langmuir model; highlighted in gray means R2 above 0.9000
The highest values of the correlation coefficient R2 (above 0.9000) are highlighted in gray in Table 3. As seen from the R2 data, the Langmuir I model fits the data on Pb and Cd for peat C (values of0.9940 and 0.9366, respectively) and Pb, Zn and Cd for peat LA (0.9369, 0.9772 and 0.9264, respectively), and Langmuir II was a good fit of the data on Cd for peat C (0.9607) and Zn for peat LA (0.9938). The Freundlich isotherm model also fit the data for most metallic ions, except for Zn on peat C (0.6378) and Cd on peat LA (0.6574). The correlation coefficient of the Freundlich model was 0.9891 for Pb and 0.9387 for Cd with peat C and 0.9357 for Pb and 0.9674 for Zn with peat LA.
The obtained R2 results (from Table 3) suggest that the Langmuir isotherm model is a better fit for Pb, Cd and Zn adsorption on both tropical peats, which is in agreement with other studies of peats, including those from tropical and temperate areas (Bencheikh-Lehocine, 1989; McKay and Porter, 1997; Franchi, 2004; Qin et al., 2006; Batista et al., 2009; Soukand et al., 2010; Carvalho et al., 2017). Brown et al. (2000) found that Langmuir isotherms were frequently effective in studies modeling the binding of a metal to peat.
For the case of peat C and Zn, the good fit was not obtained for any of the models (Freundlich or Langmuir) (R2 < 0.7000). As can be seen in Figures 3a and 7, the adsorbed Zn increased rapidly with increasing Zn equilibrium concentrations in the isotherm initial stage and then increased slowly, tending to reach saturation condition. According to the system of isotherm classification (Giles et al., 1974), this behavior suggests that data experimental fit in the "L"-type curve (i.e., Langmuir). This finding is similar to the other metals studied. However, the batch equilibrium results for peat C and Zn revealed two points of adsorption capacity associated with the highest initial concentrations that appear to be above this saturation plateau. This may indicate a change in the adsorbate retention mode evidenced by the completion of the first adsorption monolayer and the start of a second adsorption layer. This may have been the cause of the lack of adjustment to the adsorption models.
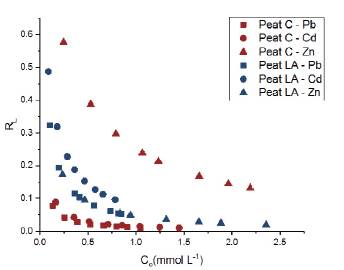
Figure 5 Plot of R versus the initial concentrations (Co) of Pb2+, Cd2+ and Zn2+ for the two Brazilian peats
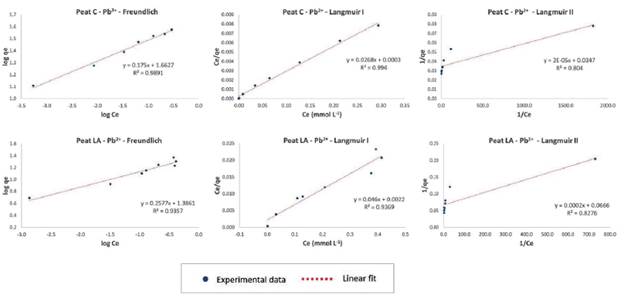
Figure 6 Adsorption isotherms of Pb ions onto Peat C (Cravinhos) and Peat LA (Luis Antonio), using Freundlich, Langmuir I and Langmuir II model fitting linearization
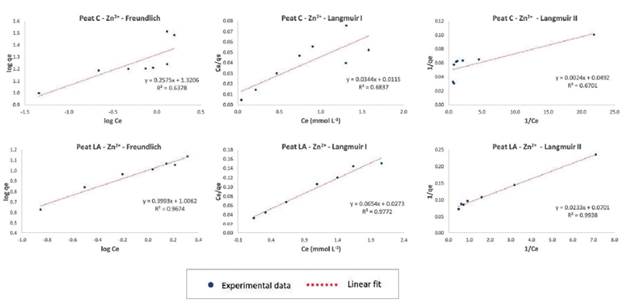
Figure 7 Adsorption isotherms of Zn ions onto peat C (Cravinhos) and peat LA (Luis Antonio) using Freundlich, Langmuir I and Langmuir I model fitting linearization
The Langmuir isotherm model is assumed to represent monolayer surface coverage without interaction between the adsorbed ions, and the existence of a value for q m (maximum adsorption) means the presence of a finite supply of sorption sites (Langmuir, 1997). Table 3 shows that peat C had higher maximum adsorption capacities (37.3134, 29.0697 and 21.2890 mmol kg-1 for Pb, Zn and Cd, respectively) than peat LA (21.7391, 14.2550 and 3.6460 mmol kg-1 for Pb, Zn and Cd, respectively). These q values and all parameters obtained from the Langmuir model were considered from the best fit of the Langmuir model: Langmuir II for the adsorption of Cd onto peat C and Zn onto peat LA and Langmuir I for the others. These considered values are highlighted with an asterisk in Table 3. Table 4 provides the monolayer adsorption capacities of some materials tested as low-cost adsorbents for metallic ions; the peats tested in the present work presented lower values than those in other studies but were still comparable. From Table 4, it is also verified that even among Brazilian tropical peats, the parameter q m varies widely, presenting values ranging from 752.86 mmol kg-1 (Lattuada etal., 2014) to 150.36 mmol kg-1 (Franchi, 2004) for Pb, for example.
Following the Langmuir model, the adsorption capacity increased in the order Pb2+ > Zn2+ ≥ Cd2+ for peat C and Pb2+ > Zn2+ > Cd2+ for peat LA, showing the higher affinity of the peat functional groups for Pb2+ and confirming the preference of peat for this cation, as previously determined. These orders are due to the characteristics of the analyzed organic materials. A similar order was obtained for the removal efficiency of peat LA (Figure 3b). For peat C, the q m values were very similar for Zn and Cd but larger for Zn. The removal efficiency was also similar for Cd and Zn but was slightly higher for Cd. For this sequence, the ion electronegativity may explain the selectivity for the metals (Table 2).
Another parameter obtained from the Langmuir isotherm model is K L , which is related to the binding energies of the adsorption process. The high K L parameters for Pb and Cd suggest stronger interactions with surface groups than those for Zn binding. The K L parameters for Pb, Cd and Zn with peat C were 89.3333, 63.7354 and 2.9913 L mmol-1, respectively, while for peat LA, the values were 20.9091, 11.9781 and 3.0077 L mmol-1, respectively. These values were considered from the best fit of the Langmuir model (Langmuir I or Langmuir II) and are highlighted with an asterisk in Table 3.
Other parameters, such as the separation parameter (R L ) and the change in free energy (ΔG), were calculated from the best fit of the Langmuir model. The separation parameter (R L ) values are shown in Figure 5; all values were in the range 0-1, suggesting that Pb, Zn and Cd adsorption in both tropical peats are favorable. In general, for both peats, the R L values indicated more favorable sorption at high initial metal ion concentrations than at a lower initial concentration. According to Poots et al. (1978) and Ho et al. (2002), values closer to zero indicate more favorable adsorption. On the basis of the R L parameter, peat C was very favorable for Pb and Cd adsorption but less favorable for Zn adsorption. In contrast, peat LA was more favorable for Pb and Zn adsorption than for Cd adsorption, following the order of preference obtained from the Langmuir model (Pb2+> Zn2+ > Cd2+). Overall, peat C is more favorable than peat LA for the adsorption of metallic ions, except for Zn.
The negative values for the change in free energy (ΔG) (Table 3) ranged between -28.2588 and -19.8527 kJ mol-1) and indicate that metal ion adsorption is spontaneous and feasible at 25°C, while the absolute magnitudes indicate a physisorption process, in accordance with the results determined from the pH data that suggested an ion exchange process. Adsorption with AG between -20 and 0 kJ mol-1 implies a physical process, while values from -80 to -400 kJ mol-1 correspond to chemisorption (Fernandes et al., 2010). In comparison, Ho (2006) obtained a value of -11.1 kJ mol-1 for the adsorption of Pb, while Balan et al. (2008) obtained a value of -26.054 kJ mol-1 for Cd adsorption. Both experiments were carried out in Sphagnum moss peat with temperatures close to 30°C, and the parameters were obtained from a Langmuir I linearization. These two values are close to those obtained for the peats in this study and show that the process of metallic ion adsorption in peat is generally achievable and spontaneous and is characterized by physical processes.
The Freundlich isotherm of sorption is also one of the most commonly used mathematical descriptions due to its wide adjustment range for experimental concentrations (Mobasherpour et al. 2012). The isotherm theory assumes that the adsorption energy decreases logarithmically as the surface becomes covered by the adsorbate. The Freundlich isotherm is better adjusted to heterogeneous surfaces (Soares and Casagrande, 2000). As occurred for the Langmuir model, most metallic ions were well adjusted to the Freundlich model, with the exception of Cd on peat LA (R2 of 0.6574) and Zn on peat C (0.6378) (Table 3). For the adjustment of Cd on peat C and Pb on peat LA, the correlation coefficients of the Freundlich isotherm (0.9387 and 0.9357, respectively) were practically the same as those of Langmuir I (0.9366 for Cd and 0.9369 for Pb). Lattuada et al. (2014) and Koivula et al. (2009) also found Freundlich to be the most suitable isotherm for Pb adsorption to tropical peat (from Brazil) and temperate peat (from Finland); Lattuada et al. (2014) and Kalmykova et al. (2008) found the same for Zn, and Qin et al. (2006), Balan et al. (2008) and Kalmykova et al. (2008) found the same for Cd.
The Freundlich constant n for all ions was less than 1 and ranged from 0.1586 to 0.3993 (Table 3), indicating favorable adsorption, strong interaction and surface heterogeneity. The closer the value n is to zero, the more heterogeneous the surface is (Soares and Casagrande, 2000; Bulgariu et al., 2011). Zhang et al. (2010) described n values of humic acid from sediment ranging from 0.735-0.902 and suggested unevenly distributed sorption sites on this material. K f is considered a Freundlich parameter related to the adsorption capacity (Oliveira et al. 2015), and the determined values indicate the great ability of peat C and peat LA to retain Pb (K f of 45.9934 and 24.3276 L kg-1, respectively). For Zn, the parameters were 20.9218 L kg-1 for peat C and 10.1438 L kg-1 for peat LA, while for Cd, the values were 29.9778 and 3.3527 L kg-1, respectively. The Kf values from this study are in agreement with values obtained for adsorption in soils, such as Zn and Cd adsorption in 11 soils (Spodosols and other types); the Kf ranged from 2.1 to 776.3 L kg-1 and 5.4 to 755.09 L kg-1, respectively (Buchter et al., 1989).
In addition to the adsorption efficiency of the material, the cost is also an important parameter and is even considered essential in the selection of any treatment process. As this study classifies tropical peat as a low-cost adsorbent, price analysis was also conducted.
In the Brazilian market, local peats have been sold on a large scale in 2019 for an average of U$0.05 per kilo (approximately R$0.22 in national currency). On the other hand, in the same market, activated carbon is traded at between U$1.20 and U$2.10 per kilo (approximately R$5.00 - R$9.00), average prices that are 33 times higher than the price estimated for peats. Activated carbon is the most common and efficient adsorbent but has disadvantages due to its cost (especially in developing countries) (Silva et al., 2020). The financial advantage of peat as an alternative and low-cost adsorptive material confirms the importance of the study of local and efficient materials.
In general, both tested tropical peats are efficient adsorptive materials, especially for the removal of Pb. The peat deposits are located near industrial regions and are already close to areas with great potential for contamination. Both peats are therefore easily accessible materials for the remediation of these contaminated areas and can be used in adsorption technologies. These materials can maintain high efficiencies for contaminant removal but reduce the associated treatment costs. Thus, these findings provide direction for the use of local materials in a tropical area for soil and water treatment. According to Crini (2006) and Sharma et al. (2011), an adsorption process can be an attractive technology if the low-cost adsorbent is easy to acquire and ready for use.
Conclusion
The batch adsorption experiments showed a high percentage of Pb, Zn and Cd removal by both tropical peats, with the removal efficiency being a function of the initial concentration of the cation. The removal order was Pb2+ > Cd2+ ≥ Zn2+ for peat C and Pb2+ > Zn2+ > Cd2+ for peat LA, showing the higher affinity of both peats for Pb. Pb removal by both materials ranged from approximately 46.0 to 100.0%. Regarding Zn and Cd, the efficiency of removal by peat C remained between 90.8 and 27.8%, while that by peat LA was between 52.6 and 9.8%.
Peat C was slightly more efficient than peat LA, which may have been due to the characteristics of peat C that were more favorable to adsorption than those of peat LA, such as the higher surface area, higher organic matter content, and lower pH of peat C, which resulted in a lower pH in the initial test condition ("initial pH"). Peat C is already commercialized in Brazil, which would further facilitate its use as an adsorptive material.
During the batch assays, the increase in solution pH after adsorption as the initial concentration increased suggested an ion exchange mechanism. This finding is in accordance with the parameter ΔG, which implied a physisorption process. According to the parameters ΔG and R L , adsorption occurred in favorable, spontaneous and feasible conditions.
Among the isotherm models used to describe the metal adsorption, Langmuir had the best correlation, which included the best fit for both the Langmuir I and Langmuir II models. These best fits assume a monolayer sorption capacity (qm ), decreasing in the order of 37.3134, 29.0697 and 21.2890 mmol kg-1 for peat C and 21.7391, 14.2550 and 3.6460 mmol kg-1 for peat LA for Pb, Zn, and Cd, respectively. Such adsorption capacities are comparable to the values for other peats (including temperate and tropical peats), as well as for other biosorbents, proving the potential use of the tested peats.
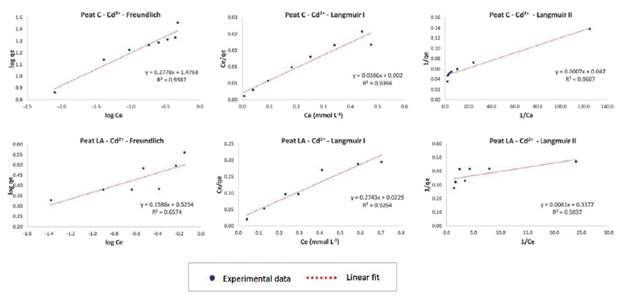
Figure 8 Adsorption isotherms of Cd ions onto peat C (Cravinhos) and peat LA (Luis Antonio) using Freundlich, Langmuir I and Langmuir II model fitting linearization
In some cases, the Freundlich model also achieved a high adjustment, comparable to that of the Langmuir model. The removal of Pb and Cd by peat C (R2 of0.9891 and 0.9387, respectively) and Pb and Zn on peat LA (R2 of0.9357 and 0.9674, respectively) indicated favorable adsorption, strong interaction and surface heterogeneity.
Regarding costs, the price of peat in the Brazilian market was found to be 33 times lower than that of activated carbon (an efficient adsorbent with regular use).
In general, both tropical peats are efficient, alternative and low-cost adsorption materials, especially with respect to the removal of Pb. Sources of both peats are located near large industrial areas in Brazil, and the presented findings contribute to the use of local materials to remediate contaminated sites, thus lowering costs and maintaining efficiency.