Introduction
Lamprophyres comprise a set of uncommon hypoabissal mafic-ultramafic rocks usually of restricted occurrence as sills or dykes, occasionally as dyke swarms or stocks. They are usually linked to lamproite, kimberlite and carbonatite petrogenesis and to deep sublithospheric mantle melts. This group of rocks is characteristic of volatile-rich magmas, with fast ascent and emplacement at crustal levels during regional stages of lithospheric relaxation (Rock, 1987, 1991; Mitchell, 1994; Tappe et al., 2008). Alkaline magmatism is frequent in within-plate settings (Whalen et al., 1987; Bonin, 1996), associated with extensional processes. According to Rock (1991), chemical affinities define calk-alkaline, alkaline and ultramafic lamprophyres; the first usually being related to convergent settings, whereas the others two can be potentially related to large igneous provinces. Mostly, mafic dykes are prominent extensional structures and are helpful tools for paleogeography reconstructions as markers of former conjugate margins that were separated probably in relation to mantle plumes (e.g., Bleeker and Ernst, 2006; Ernst and Buchan, 2001a, b; Halls and Fahrig, 1987).
Mafic dykes have been formerly reported for the Rio de la Plata Craton (RPC), both for the Uruguayan shield and for the Tandilia belt in Argentina by Bossi et al., 1993; Mazzucchelli el al., 1995; Teixeira et al., 1999; Iacumin et al., 2001; and Teixeira et al., 2002; among other references. According to geochemical and isotopic studies, they correspond to a basaltic-andesite composition, with tholeiitic and calk-alkaline chemical affinity, and Paleo-Mesoproterozoic ages (Teixeira et al., 2013; Girardi et al., 2013).
Alkaline to sub-alkaline mafic magmatism has been found in southeast Uruguay, associated with the extensional events of the opening of the South Atlantic Ocean (Lustrino et al., 2005; Cernuschi et al., 2015).
The first references of lamprophyric dykes in Uruguay, that described the structural and petrographic features of mafic dykes located in the Montevideo coastal area, belong to Walther (1935; 1948). Later, regional studies on the crystalline basement of the RPC characterized these dykes as "mafic alkaline dykes", tentatively assigning them a Neoproterozoic age according to their field relationships, since they crosscut Paleoproterozoic units (Coronel and Oyhantçabal, 1988; Masquelin et al., 2003; Oyhantçabal et al., 2003; Pascale and Oyhantçabal, 2010).
Early reports of lamprophyres in the Tandilia basement (Argentina) correspond to the work of Quartino and Villar Fabre (1967), who described these rocks as possible deformed amphibolites. More recently, Dristas et al. (2013) carried out a petrological characterization on an ultramafic lamprophyre, also complemented by isotopic studies and K-Ar (on biotite/phlogopite) dating, yielding a minimum age of 1928 ± 54 Ma and a calk-alkaline nature.
Therefore, this paper is focused on the petrological characterization of a set of lamprophyric dykes in Uruguay and the first 40Ar-39Ar (on biotite/ phlogopite) dating, in order to classify them and to constrain this magmatism in the context of the RPC.
Regional framework
Almeida et al. (1973) originally defined the Río de la Plata Craton (RPC), including under this term the ''ancient cratonic areas'' of the southernmost South American platform already amalgamated by the upper Proterozoic.
The RPC represents one of the major nuclei of Western Gondwana, surrounded by late Neoproterozoic-Cambrian orogenic belts (e.g., Almeida et al., 2000; Cingolani 2010; Rapela et al., 2011 and references therein). The main tectonic units exposed in the Uruguayan shield are represented by Piedra Alta (Bossi et al., 1993) and Nico Pérez (Bossi and Campal, 1992) terranes, which are separated by the Sarandí del Yí shear zone (Preciozzi et al., 1979). Both Piedra Alta and Nico Pérez terranes in Uruguay, together with Buenos Aires Complex (BAC) in Argentina (Marchese and Di Paola, 1975) and Taquarembó block in southern Brazil, constitute the RPC (Figure 1a), according to the original definition of Almeida et al. (1973). Recently, Oyhantçabal et al. (2011) redefined the RPC limits in Uruguay and excluded the Nico Pérez terrane and Taquarembó block from the craton, after new geochronological, isotopic and gravity data interpretations.
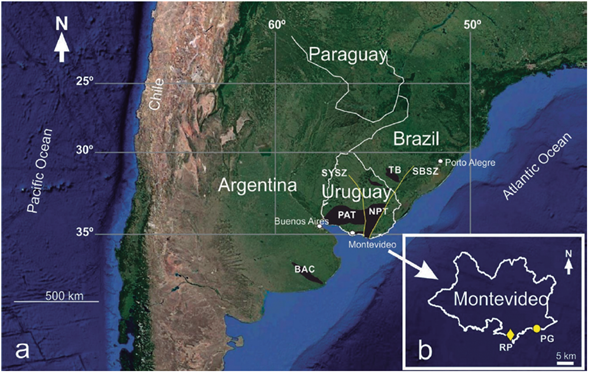
Figure 1 (a) Main Rio de la Plata cratonic exposures (b) Location of the outcrop areas in the coastal zone of Montevideo city. References: PG - Punta Gorda site, 34°54'00"S - 56°4'53"W; RP - Rodó Park site, 34°55'11"S - 56°10'20"W; PAT = Piedra Alta Terrane, NPT = Nico Pérez Terrane, BAC = Buenos Aires Complex, SYSZ = Sarandí del Yí shear zone, Sierra Ballena shear zone, TB = Taquarembó Block.
Most of the RPC consists of Paleoproterozoic crust over a large region of extension covered by Phanerozoic sediments; with characteristics of mature continental crust (Cordani et al., 2000; Pankhurst et al., 2003; Peel and Preciozzi, 2006; Rapela et al., 2007). Remnants of Archean crust have been recognized by Hartmann et al. (2001) in Nico Pérez terrane (NPT).
The Piedra Alta terrane (PAT; Figure 1a) is comprised of low- to medium-degree metamorphic orogenic belts and plutonic suites (ca. 2.1 Ga), a layered mafic complex, late- to post-orogenic magmatism (1.9-2.3 Ga) and extensional magmatism (1.7 Ga) represented by the Florida mafic dyke swarm (Preciozzi et al., 1999; Bossi and Cingolani, 2009; Rapela et al., 2007; Sánchez Bettucci et al. 2010; Oyhantçabal et al., 2011; Hartmann et al., 2000). Peel and Preciozzi (2006) proposed that the PAT represents a juvenile Paleoproterozoic unit that has been stable since 1.7 Ga, without a record of the influence of Neoproterozoic orogenies. Similar features were also defined for the BAC (e.g., Bossi and Cingolani, 2009; Rapela et al., 2007; 2011). Halls et al. (2001) and Teixeira et al. (2013), reviewing the ENE-WSW trending Florida dyke swarm from PAT and the N-NW Tandil dykes in the BAC, obtained high-quality U-Pb (ID-TIMS in baddeleyite) ages of 1790 ± 5 Ma and 1589 ± 3 Ma, respectively. According to these results, Teixeira et al. (2013) suggested that both units underwent extensional regimes at the Paleo-Mesoproterozoic boundary.
The study area is located in the southern portion of the PAT, along the coastal margin of Montevideo city (Figure 2). This area consists of medium grade San José metamorphic belt (Bossi et al. 1993; Oyhantçabal et al. 2003), which includes the Montevideo Formation and the Punta Carretas Orthogneissic Unit. Both units are crosscut by pegmatitic and aplitic dykes and, by the mafic dykes of the present study.
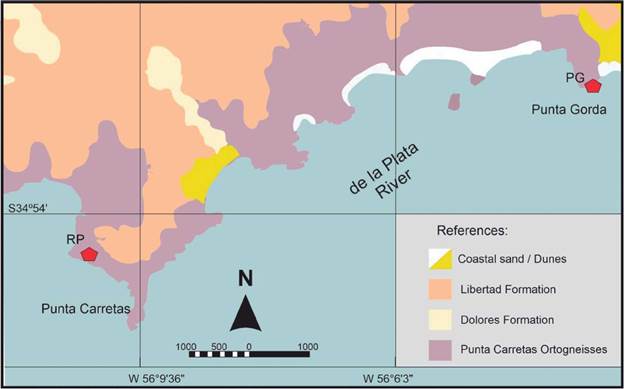
Figure 2 Geological sketch of the Montevideo coastal area (modified after Spoturno et al. 2004). References: Libertad Formation: Quaternary fine sediments; Dolores Formation: Quaternary wackes and mud sediments.
The Montevideo Formation (Bossi 1965) is a supracrustal volcanic-sedimentary sequence metamorphosed under amphibolite facies conditions (Oyhantçabal et al. 2002; 2003). It is composed of concordant para-gneiss, para-amphibolites and mica schists, with an EW regional structural trend (Pascale 2013).
The Punta Carretas Orthogneissic Unit was defined by Oyhantçabal et al. (2003), who identified the orthogneiss as resulting from metamorphic recrystallization of calk-alkaline granitoids. It represents the most widespread unit of the crystalline basement in Montevideo, intercalated with rocks of the Montevideo Formation and associated granitoids (Pascale 2013). They present structural trends of N280° to N70° of light-gray to pinkish granolepidoblastic rocks, composed of oligoclase, quartz, biotite, epidote, muscovite, apatite and microcline, and strongly crosscut by pegmatitic and aplitic intrusions. The only radiogenic age available for this unit, by Rb-Sr whole rock method, yielded and age of 1990 ± 32 Ma (Cingolani et al. 1997).
Previous work, involving some petrographic and structural features of the lamprophyric dykes, inferred that they represent at least the last magmatic event affecting the Montevideo Formation (Pascale and Oyhantçabal 2010; Pascale 2013).
Analytical procedures
Seven rocks with minimal visible secondary alteration and corresponding to two different set of dykes from the coastal area were sampled, examined in thin section and prepared for lithogeochemical analyses. Three polished petrographic sections were also prepared at the Instituto de Ciencias Geológicas for SEM-EDS and BSE analyses. These analyses were developed with the JEOL 5900 - Low vacuum equipment at the Faculty of Sciences, Uruguay. Analytical techniques that are routine for this type of equipment as well as standard mineral spectra from Severin (2004) and Reed (2005) were used.
All the chemical data interpreted here as well as the location of the dykes are shown in Table 1. All samples were carefully cleaned, crushed and further pulverized using an agate mill at the Instituto de Ciencias Geológicas (Montevideo). Major and trace elements were analyzed by ACME-Bureau Veritas Laboratories (Vancouver, Canada) following the LF- 202 Litho-Research package methodology. Ba, Sr, Y, Sc, Zr, V, and Be were analyzed using inductively coupled plasma-atomic emission spectrometry (ICP-AES). Trace-elements (including REE) were determined by inductively coupled plasma-mass spectrometry (ICP-MS). The analytical protocol at the ACME Laboratory includes the analysis of reference materials (STD-DS10; STD-GS311-1; STD-GS910-4; STD- OREAS45EA; STD- SO-18 and STD SO-19).
The 40Ar/39Ar geochronology was carried out at the University of Nevada (Las Vegas), using the data reduction LabSPEC software written by B. Idleman (Lehigh University). Biotite crystals of one sample of a lamprohyre dyke (PG-01) were separated using conventional techniques and then irradiated at the U.S. Geological Survey TRIGA Reactor (Denver, Colorado) for 20 hours, along with the GA-1550 biotite standard together with CaF2 and K-glass fragments. After a period of cooling, the irradiated sample was loaded for step heating in a Cu sample tray in a high vacuum extraction line and were fused using a 20 W CO2 laser and measured using a Balzers electron multiplier mass spectrometer. The measured (40Ar/39Ar)K values were 4.1 (± 58.54%) x 103. The Ca correction factors were (36Ar/37Ar)Ca = 2.55 (± 3.50%) x 10-4 and (39Ar/37Ar)Ca = 6.97 (± 3.69%) x 10-4. The J factors were determined by fusion of 4-8 individual crystals of GA-1550 biotite neutron fluence monitors, giving a reproducibility of 0.3% to 0.8% at each standard position. The measured 40Ar/36Ar ratios were 282.36±1.41%; thus, a discrimination correction of 1.0467 (4 AMU) was applied to the measured isotope ratios. An age of98.50 Ma (Spell and McDougall 2003) was used for the GA-1550 biotite fluence monitor to calculate the ages of the samples. The criterion followed to define a plateau age is the identification of three or more successive steps overlapping with an error at 2o level that together comprise >50% of 39Ar released. All analytical data are reported at the confidence level of 1o (standard deviation) and shown in Table 2.
Table 2 Ar-Ar biotite analysis for the sample PG01. References: Biotite, 3.74 mg, J = 0.00497 ± 0.13%. 4 amu discrimination = 1.0467 ± 1.41%, 40/39K = 0.0041 ± 58.54%, 36/37Ca = 0.000255 ± 3.50%, 39/37Ca = 0.000697 ± 3.69%. Isotope beams in mV, r.l.s.d. = released, error in age includes J error; all errors 1 sigma. (36Ar through 40Ar are measured beam intensities, corrected for decay for the age calculations).
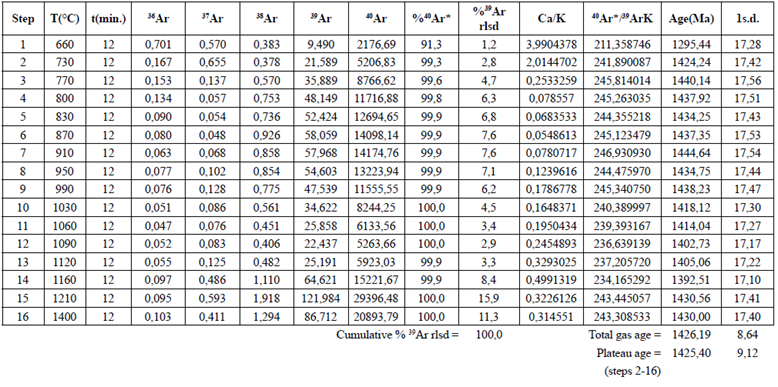
Petrographic features
Mafic dykes are gray to black, fine to medium grained, 0.3 to 2.0 meters width and tabular in shape. They often show irregular contacts with PAT units and are affected by two sets of NW and N-NW vertical to subvertical fractures. Sometimes they show an anastomosed pattern (Figure 3a), with sharp contacts and thin chilled margins (Figure 3d). No evidence of contact metamorphism has been found.
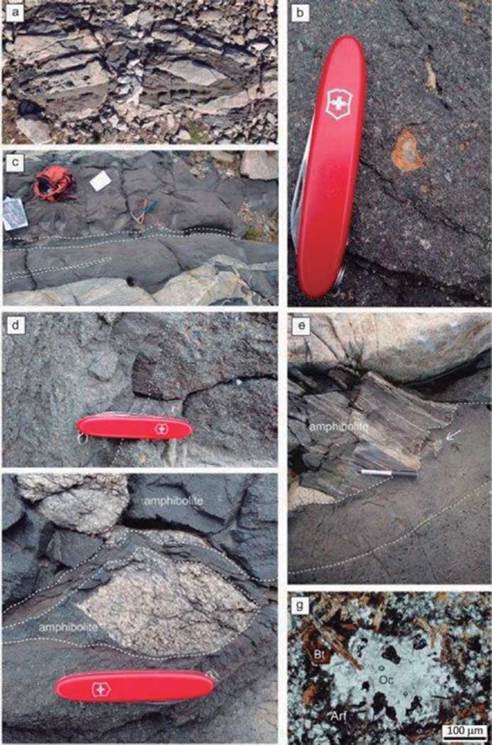
Figure 3 (a) Dyke showing anastomosed pattern at Punta Gorda (PG) site; (b) Ocelli with carbonate alteration rim; (c) dyke in dyke intrusion (PG site); (d) Detail of contacts with host rocks; (e) and (f) xenoliths from the surrounding crystalline basement represented mainly by ortho-gneiss and amphibolite; (g) ocelli filled mainly by carbonates, zeolites with halos of opaque minerals and arfvedsonite.
They are concordant with the regional foliation trend (N70°-N80°). Even the smaller dykes with widths of a few centimeters often intrude through lateral fractures with a NW direction. Double intrusion processes or "dyke in dyke" features are observed (Figure 3c), which has been well documented as a common process for lamprophyres worldwide (Rock 1987). They have porphyritic texture with increasing grain size toward the dyke center. Occasionally, the central zone is porphyritic and vesiculated, with biotite/pyroxene macrocrysts and globular structures.
These globular structures (ocelli;Phillpotts 1990) are almost spherical but frequently show coalescence, developing irregular shapes (Figure 3b). They vary in size between 0.1 cm to 1.5 cm, and they are filled mainly by carbonates, zeolites with halos of opaque minerals and arfvedsonite (Figure 3g).
Mafic and felsic xenoliths from the surrounding crystalline basement are frequent, represented by ortho-gneiss and amphibolite (Figures 3e and 3f).
Microscopically, rocks are porphyritic to glomeroporphyritic dykes, with biotite-phlogopite, augite-aegirine as main macrocrysts and with olivine and leucite subordinately; in a groundmass composed of alkaline feldspar (orthoclase), clinopyroxene, carbonate (dolomite), leucite, and opaque minerals (Figure 4a). The groundmass is very fine grained, showing an intergranular texture (between 50-100 um). Feldspathoid glomeruli and carbonate patches are present within the groundmass (Figure 4b).
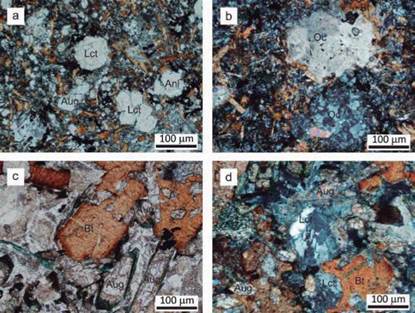
Figure 4 Photomicrographies under polarized microscope (crossed nicols), (a) Detail of the ocelli with leucite (Lct) and analcite (Anl) and phenocryst of augite (Aug); (b) Coalescent ocelli (Oc) filled with feldspathoids; (c) Augite (Aug) and biotite (Bt) phenocrysts; (d) Ocelli with carbonates (border) and leucite (Lct); groundmass with leucite, biotite, augite and carbonates.
Globular structures (ocelli) are a common petrographic feature with radial halos of biotite-phlogopite and pale blue acicular amphibole, probably riebeckite/arfvedsonite (Figure 4c). Other ocelli, between 50-300 um sized, are filled by carbonates, alkaline feldspar (orthoclase) and/or feldspathoid (leucite), (Figure 4d). According to these petrographic features they would correspond to Type 1 aggregates, as defined by Azbej et al. (2006). Opaque minerals vary approximately 5-10 modal %, they have subautomorph shapes and they are sometimes interstitially distributed in the groundmass.
SEM-EDS studies were developed to better characterize the mineral composition of the groundmass. The spectra obtained were compared to standard mineral spectra from Severin (2004) and Reed (2005) in order to identify part of the minerals of the dykes. Some of these representative minerals and their spectra are presented in Figure 5.
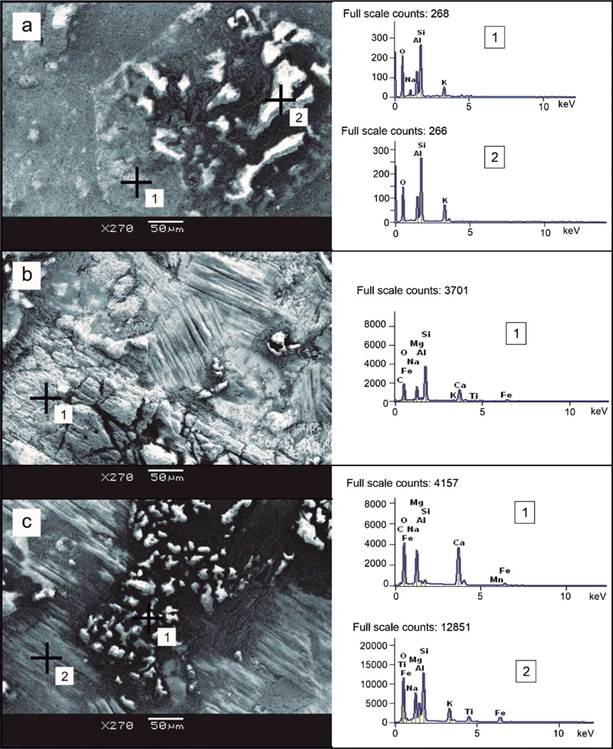
Figure 5 (a) BSE image with EDS spectra of leucite/nepheline crystal (sample PG-01); (b) BSE image of augite and phlogopite crystals (sample PG-04), EDS spectrum of augite. (c) BSE image of dolomite with spongy texture, showing high relief relative to the biotite and their respective EDS spectra (sample PG-01).
Lithogeochemistry
Analyses of seven samples and their locations are presented in Table 1. The dykes are low in SiO2 (45.21%-51.43%), high in MgO (8.52%-11.08%), FeOt (9.92%-10.42%), and TiO2 (2.98-3.27%) and show exceptionally high values of K2O ranging between 7.09-8.13%. They are enriched in incompatible elements, such as the HFSE, Zr (556-663 ppm), Nb (72-112 ppm) and Th (1120 ppm) and the large ion lithophile elements, such as Ba (4486-5980 ppm), Sr (1017-2026 ppm) and Rb (129 -248 ppm).
According to the TAS diagram (Le Bas et al. 1986; Figure 6) the samples can be classified as phonotephrites/tephriphonolites. All the samples have total alkalis close to 10 wt% and K2O/Na2O ratios approximately 4, a relation that points to a possible ultrapotassic character/shoshonitic nature.
Using the Al2O3 versus CaO classification diagram from Foley et al. (1987) and the K2O-MgO-Al2O3 ternary diagram from Rock (1987), the samples can be classified as lamprophyres and, according to their alkali values the samples overlapped the fields lamprophyres/lamproites (Figure 7b).
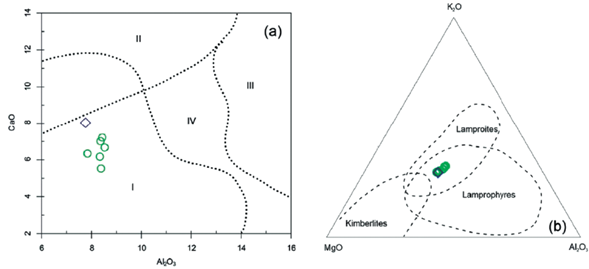
Figure 7 Classification diagrams for ultrapotassic rocks according to (a) Foley et al. (1987) and (b) Rock (1987). Symbols are the same as in Figure 6. References Fig. 7a: I - Lamproites; II - Kamafugites (low SiO2, high Cao); III - Orogenic areas (high CaO and Al2O3) and IV - Transitional group.
As shown in Table 1, the samples have high alkali values (mean K2O + Na2O > 8, mostly close to 10 wt.%), with K2O/Na2O > 3. In order to constrain the alkaline affinity of the dykes and to discard a possible shoshonitic nature the K2O - Na2O diagram was used, showing as a result an ultrapotassic character for all the samples (Figure 8).
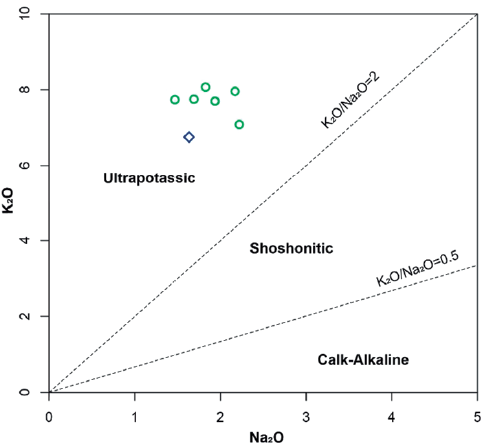
Figure 8 K2O - Na2O diagram, depicting the alkaline character (from Foley et al. 1987, after Turner et al. 1996). Symbols are the same as in Figure 6.
The REE patterns normalized to chondrite (Boynton 1984) are strongly fractionated, showing values from La to Lu between 110 - 600 ppm (Figure 9a), with different trends for the samples. In the primitive mantle-normalized multielement plot (Sun and McDonough 1989; Figure 9b), a significant enrichment in LILEs relative to HFSEs is observed, showing a steep pattern with through at U, Sr, and P and remarkable Ba and Nd positive spikes.
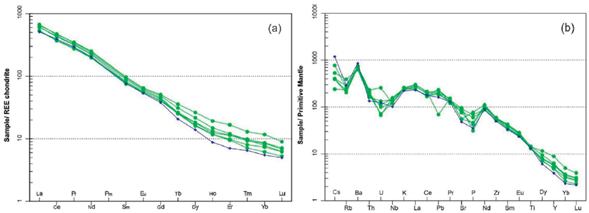
Figure 9 REE patterns and spider-diagrams, normalized according to (a) Chondrite (Boynton 1984); (b) Primitive mantle (Sun and Mc Donough 1989). Symbols are the same as in Figure 6.
The dykes have high Nb/Ta ratios, similar to the primitive mantle. Also, high Nb and Zr values with relatively low values of Hf and Y are commonly observed in alkaline rocks from within-plate settings (Green 1995).
40 Ar/ 39 Ar results
The biotite-phlogopite analysis from the center of one dyke (N° PG-01, site PG; Figure 2) produced relatively undisturbed spectra and a well-defined plateau age (Figure 10, Table 2) of 1425.40 ± 9.12 Ma (1a). The plateau is defined by the release of at least 99% of 39Ar gas in fifteen consecutive steps out of sixteen.
The anomalously young apparent age in the first low-temperature step yielded a general increasing trend for this spectrum. Minor disturbances also occur in high-temperature steps, suggesting degassing from high temperature resilient impurities. No statistically valid isochron was obtained.
Discussion
According to the petrographic features described previously, the analyzed dykes can be classified as lamprophyres. Based on the latter, the mineral assembly (biotite-phlogopite + augite ± aegirine augite ± altered olivine ± dolomite ± amphibole ± chlorite ± feldspars and feldspathoids, in the groundmass) and the high values of K2O, P2O5 and Ba pointed to their classification as minettes (Rock 1991; Le Maitre et al. 2002). Although the presence of feldspathoids in minette type lamprophyres is not provided by the IUGS classification, it has been reported by several authors (e.g., Esperanza and Holloway 1987; Wallace and Carmichael, 1989; Bhowmick, 2000; Gupta 2015). In addition to the general mineralogical features, other chemical criteria can be taken into account to constrain their classification (Mitchell and Bergman, 1991).
In this sense, the dykes from Montevideo are not only ultrapotassic (K2O/ Na2O > 3) but also peralkaline (K2O+Na2O)/Al2O3 > 1) and perpotassic rocks (K2O/Al2O3 > 1), with CaO and Fe2O3t ≤ 10 wt. % and a high Ba (>5.000 ppm), TiO2 (~3 wt. %), Zr (~650 ppm) and Sr (~1500 ppm). All these are typical characteristics of lamproites according to Foley et al. (1987) and Le Maitre et al. (2002). Nevertheless, the typical lamproite mg# values are higher than 70, and the La content is higher than 200 ppm whereas in the studied dykes they are between 62-67 and approximately 182, respectively. The dykes present a mean value of 3.7 for the Nb/Y ratio, which characterizes them as alkaline (Winchester and Floyd, 1977). Alkaline lamprophyres usually have Cr, Co and Ni contents of 97, 38 and 65 ppm, respectively. In contrast, the lamprophyric dykes of Montevideo have these values of approximately 480, 56 and 172 ppm, close to those representatives of ultrapotassic lamprophyres (Mitchell and Bergman, 1991).
All dykes present high K/Ti and (La/Sm)N ratios (>2.3 and > 2.6, respectively) that are commonly related to enriched mantle sources (Mahoney et al. 2002). The mg# (62-67) suggests a mantle source for these dykes from values of Sc (15-16 ppm), Cr (420-520 ppm), Co (47-75 ppm) and Ni (143-239 ppm) (Rhodes, 1981).
Alkaline magmatism is frequent in within-plate environments, related to extensional processes (Whalen et al. 1987) and is characterized by high concentrations of HFSE and low values of LILE. Alkaline rocks showing strong enrichment in LILE and LREE are commonly associated with anorogenic or post-orogenic settings (Plá et al., 2011).
Alkaline ultrapotassic magma shows high incompatible element content regardless of the tectonic environment supporting an origin by partial melting of lherzolites (low rates <1%) from an enriched mantle source (Lloyd et al. 1985; Foley 1992; Foley and Peccerillo 1992; Ionov et al. 1997). Furthermore, high concentrations of LREE have been observed for other ultrapotassic within plate rocks, such as lamprophyres and lamproites as well as post-collisional lamprophyres like minettes (Foley 1992; Gibson et al. 1992). The high concentrations of Y and Zr, and relatively lower concentrations of Hf, Nb, Ta and HFSE are consistent with a within plate environment (Foley and Peccerillo, 1992). The Nb/U ratios for lower and upper crust are 21 and 9, respectively; rocks affected by crustal contamination present Nb/U values between 9 and 40 ppm (Gregoire et al., 2000). The dyke mean values of 43 ppm suggest that crustal contamination during the magmatic ascent/ emplacement is not obvious.
Several authors (e.g., Masquelin et al. 2003, Oyhantçabal et al., 2003, Pascale and Oyhantçabal, 2010) have attributed a Cambrian age to these dykes because other mafic dykes located to the North of Montevideo city crosscut the La Paz granite (585 ± 4 Ma, Cingolani et al. 2012). Martino (2017) analyzed these dykes and found that they correspond to lamprophyres of calk-alkaline affinity, and according to their petrographic features they were classified as espessartites. Interesting features that support their contemporaneity of emplacement with the La Paz granite are evidence of magma mingling processes. Therefore, these calk-alkaline lamprophyres are clearly different and later than the dykes discussed in this paper. Mafic dykes of Mesoproterozoic age have been mentioned for the RPC, but in general, with different mineralogy and/or chemical affinity (Girardi et al. 2013; Teixeira et al. 2013; Dristas et al. 2013, among others). An interesting fact reported by these authors is that according to the isotopic data from the Tandil dykes, an enriched original source could be a characteristic feature of the mantle of the Rio de la Plata Craton in Paleoproterozoic time. Lamprophyric dykes were only reported by Dristas et al. (2013), with a minimum age of 1.92 ± 54 Ga obtained by the K-Ar method on phlogopites. Teixeira et al. (2013) proposed the idea that the Columbia supercontinent started a major break up at 1.59 Ga, based on geochronological and paleomagnetic data. Therefore, the emplacement of the lamprophyric dykes studied in this paper may be related to the initial stages of a later major extensional event.
Conclusions
The lamprophyric dykes from Montevideo, with variable structural trend between N75°-N85°, have porphyritic texture with mainly phlogopite phenocrysts and a groundmass composed of biotite-phlogopite + augite -aegirine augite ± altered olivine ± dolomite ± amphibole ± chlorite ± feldspars and feldspathoids. Ocellar structures with carbonate, zeolites, leucite and fibrous alkaline amphibole are present.
They are chemically ultrapotassic dykes (K2O/Na2O > 3), with peralkaline (K2O+Na2O)/Al2O3 > 1) and perpotassic nature (K2O/Al2O3 > 1), with CaO and Fe2O3t ≤ 10 wt. %, very high Ba (>5.000 ppm), TiO2 (~3 wt. %), Zr (~650 ppm), and Sr (~1500 ppm), mg# approximately 63-67 and they are highly enriched in LILE in relation to primitive mantle.
According to the mineralogical criteria established by the IUGS the dykes can be classified as minettes (lamprophyres) and from a chemical point of view as alkaline/ ultrapotassic lamprophyres.
The chemical features point to a deep/enriched mantle source for the origin of this magma related to a within-plate tectonic setting.
The crystallization age of 1.42 Ga determined by the Ar/Ar method on biotite (phlogopite), points to an extensional event for the Rio de la Plata craton at that time.