Introduction
The last millennium was a critical period for understanding environmental change because ofthe occurrence of major changes in climate, particularly during crucial periods such as the Medieval Climate Anomaly (MCA) (approximately AD 900-1300) (Soon et al., 2003; Gao et al., 2015; Peteet et al., 2020) and the Little Ice Age (LIA) (approximately AD 1300-1850) (Mann et al., 2009; Sun et al., 2020). Hydroclimatic variability demonstrates significant regional differences divided by the summer monsoon boundary in China compared to increasing trends in temperature (Chen et al., 2010; Chen et al., 2015). Most researches analyzing the variability of moisture and precipitation in eastern China over the last 1000 years have been based on historical documents (Zheng and Wang, 2005; Zheng et al., 2006).
Multi-proxy records from lake sediments are main sources of evidence for paleoenvironmental changes. Sedimentary lithology gives an overview about the compositional variability of the sediment records and the occurrence of unconformities. Palynological investigations of lacustrine sediments are useful tools for reconstructing past vegetation and hydrologic changes in lake ecosystems that were responses to variations in climate (Djamali et al., 2009). However, reconstructing climate based solely on pollen analysis could be biased due to human influence (Li et al., 2014). The sizes of lacustrine sediments grains are controlled primarily by water dynamics and sediment sources, which in turn are influenced by various factors such as local climate changes, regional hydrological transitions, and human activity (Lerman, 1978). Therefore, the environmental significance of variations in grain size is complex. The combined study of lithology, pollen assemblages and grain size has the potential to provide different yet complementary information about the paleoenvironment (Li et al., 2009).
During the historical period, several lakes have developed in the area where the Huai River, the Yellow River, and the Jing-Hang Grand Canal converge. This vitally contributed to the water conservation, flood control, and social economy development. Multi-proxy limnologic studies of these lakes using different time scales revealed that the evolution of the regional environment was frequently affected by the interaction between the large rivers, which formed the remaining lacustrine deposits, creating important archives for use in the study of the regional hydrological and environmental evolution (He et al., 2005; Zhang et al., 1999; Xia et al., 1995; Chen et al., 2013). Located in the lower reaches of the Huai River and west of the Jing-Hang Grand Canal, Gaoyou Lake is the sixth largest fresh-water lake of China (Fig. 1). Early studies largely based on historical records show that Gaoyou Lake was comprised of lagoons during the Early Holocene (Ling, 2001). The area of Gaoyou Lake was diminished to a shallow bay approximately 8,000 BP, when sea transgression reached its shorelines. Interfluve land was present between the Gaoyou and Baoying counties until 6,000 BP. In the historical period, Gaoyou Lake was part of the waterways of the Yellow River and Huai River and served as the water resource to the Grand Canal. As a result, the evolution of Gaoyou Lake was closely linked with the large river system and regional climate changes in eastern China, which made it an ideal site for the study of the regional hydrological and environmental evolution. Researches during the modern period have studied the changes in the water area, biological characteristics, and water quality of Gaoyou Lake (Wei et al., 2017; Guan et al., 2019; Zhang et al., 2020). However, the paleoenvironmental studies of Gaoyou Lake sediments have not received adequate attention. Our research team combined grain size, magnetic susceptibility, and geochemical proxies in Gaoyou Lake sediments to infer regional climate fluctuations (Li et al., 2007; Li et al., 2015). Yet, biological proxies such as pollen, one of the most sensitive paleoenvironmental indicators, have not been studied. This study presents the first pollen records collected from Gaoyou Lake, as well as grain size and lithology analyses, to provide a better understanding of the lake evolution and to deduce regional climate changes during the last millennium. The exploration of the interaction between the lake development, climate changes, and large river effects will provide scientific basis for regional ecosystem protection and construction. The aims of this paper can be summarized as:
Reconstructing the climatic and hydrological changes of Gaoyou Lake during the last millennium (past 1,100 years),
Detecting the evidence of human activities and historical events such as the course shifts of the Yellow River from the core sediments of Gaoyou Lake.
Study area
Gaoyou Lake (32°42'N-33°04'N, 119°06'E-119°25'E) spans approximately 39 km from north to south and approximately 17.3 km from west to east. It covers a 674.7 km2 area, with the lowest elevation at 3.3 m above sea level (a.s.l) and an average elevation of 4.4 m a.s.l of the lake bottom. It has an average water depth of 1.4 m and maximum depth of approximately 2.4 m (Wang and Dou, 1998). The average annual runoff into the lake is 2.2 x 1010 m3. Water from the Huai River contributes 95% of the total input for the south of the lake via the water way to the Yangtze River (Chen et al. , 2017). Several small creeks and streams-such as the Tonglong River, Xincun River, Baita River, Qinnan River, and Wangqiao River-also feed the lake (Fig. 1).
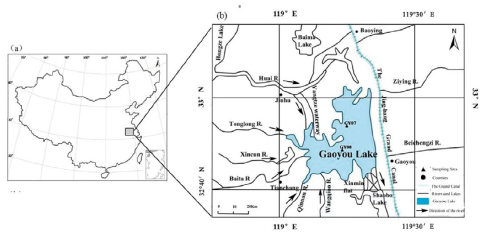
Figure 1 (a) Map showing the location of Gaoyou Lake in China. (b) The core sampling sites of Gaoyou Lake
The current catchment area has a northern subtropical monsoon climate, with an average annual temperature of 16.7°C and average annual precipitation of 1,029 mm according to the meteorological records at Gaoyou from 1981 to 2010 (Fig. 2). Precipitation during the monsoon season (June-September) accounts for 56.4% of the annual total. July (mean temperature of 27.2 °C) and January (2.8°C) are the warmest and the coldest month, respectively. The zonal vegetation in the catchment area is a mixed forest consisting of deciduous and evergreen broad-leaved trees. The current vegetation around Gaoyou Lake is mostly composed of farm and economic crops. Trees in the genera Salix, Ulmus, Populus, and Paulownia are commonly used in artificial cultivation (Wang and Yang, 1990). There are 38 genera of Planktonic algae in Gaoyou Lake, and these are dominated by Chlorophyta (40.0%), Cyanophyta (24.2%), and Bacillariophyta (13.64%) (Wei et al., 2017).
Materials and methods
Coring, dating and grain size
In November 2010, four cores (GY05, GY06, GY07, and GY08) were taken from Gaoyou Lake using a piston corer within a 2-m long and 80-mm diameter fitted steel coring tube. The cores were transported to the laboratory and stored at 4 °C. Each core was split, and the sediments were photographed and described in detail. One half of each core was archived and the other half was used for analysis. In this study, we focused on the pollen, particle size and lithology analysis of samples from Core GY08 (158 cm long) collected from the central part of the lake (32°53'36"N, 119°21'6"E) in 1.78 m of water. The lithology of Core GY07 (32°55'6"N, 119°19'39"E), collected at a water depth of 1.8 m and located approximately 12 km from GY08 was described and the core was sampled for grain size and chronological control analysis.
Grain size analyses were completed for 186 samples from GY08 at 0.5-cm interval along the top 30 cm, and 1-cm intervals between 30 and 158 cm. H2O2 and HCL were added to each sub-sample prior to analysis to remove organic and inorganic carbon. Then, the sediment was dissolved in a (NaPO3)6 solution for 24 h. Grain size distributions were determined by a laser particle size analyzer (Mastersizer 2000, Malvern Panalytical, United Kingdom). The grain size parameters were calculated using the Excel macro-program GRADISTAT (Blott and Pye, 2001), based on the following Equations S1 (Folk and Ward, 1957).
The age-depth model previously developed by Li et al. (2015) for the cores sampled at Gaoyou Lake was used for the study. In total, six calibrated accelerator mass spectrometry (AMS) 14C dates were presented in this study in two batches. Radiocarbon ages of four samples from Core GY 07 were determined at the Beta Analytic Radiocarbon Dating Laboratory in Miami, USA using AMS in 2013. Two samples (one from Core GY07 and one from Core GY08) were dated using the AMS system at the Xi'an Accelerator Mass Spectrometry Center, China, in 2020 (Table 1). The radiocarbon dates were calibrated using the software CALIB 6.0 and the INTCAL20 curve.
Table 1 Radiocarbon chronology from Gaoyou Lake sediments
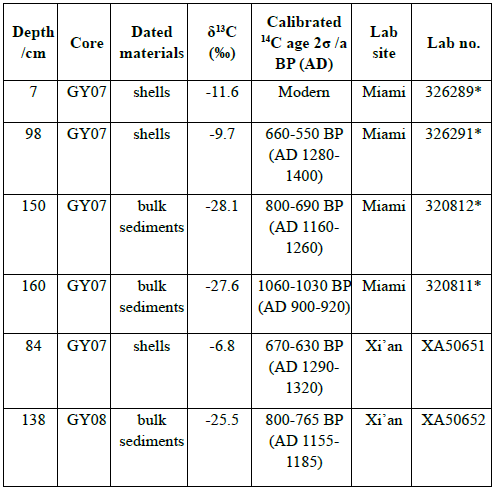
The data of the samples with * were quoted from Li et al., 2015.
Pollen analysis
Pollen samples were collected at 5-cm interval. Therefore, 30 samples were obtained with approximately 150 pollen grains in each sample. The samples were processed following the standard HF technique (Fœgri and Iversen, 1989). This involves treating 1-2 g of sediment with 10% HCL, 10% KOH, and 36% HF to remove carbonates, humid acids, and silicate, respectively. Lycopodium tablets were added to the samples to calculate the pollen concentrations. Pollen was counted and identified using an Olympus BX51 microscope at the Nanjing Institute of Geology and Paleontology, Chinese Academy of Sciences. Pollen percentage and concentration calculations were performed using the software TILIA 1.5.12. Only major pollen taxa with an occurrence frequency exceeding 2% in at least three samples were included in the numerical analyses and the pollen diagram. The CONISS program was used to divide the pollen diagram by constrained minimum-variance cluster analysis based on percentages of pollen (Grimm, 1987).
PCA and DCA analysis
Principal component analysis (PCA) is considered a powerful data reduction technique that produces a visual scatterplot for the qualitative assessment of the resemblances and differences among samples (Ter Brakk, 1988; Jiang et al., 2013; Geng et al., 2019). To ordinate the pollen data from Core GY08 and detect patterns of vegetation development, detrended correspondence analysis (DCA) and PCA were conducted based on the square root transformed pollen percentage data using CANOCO 4.5. DCA was initially performed to estimate the underlying linearity of the data. The preliminary DCA yielded a gradient length of 1.176 (< 2.0) standard units, which indicates a possible linear response of pollen assemblages to environmental variables. Therefore, PCA was chosen to ordinate the pollen data for the Gaoyou Lake sediment cores. The pollen and spore data were reduced to a two-dimensional plot and the resulting data set was displayed as a plot for samples and taxa based on PCA.
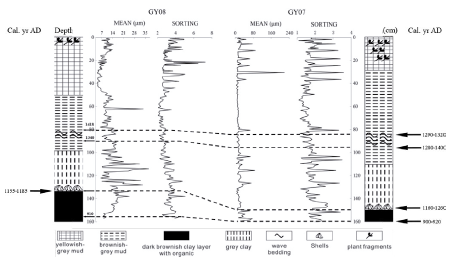
Figure 3 Lithology, grain size, and AMS 14C dates at GY07 and GY08. Data of GY07 were extracted from Li et al., 2015
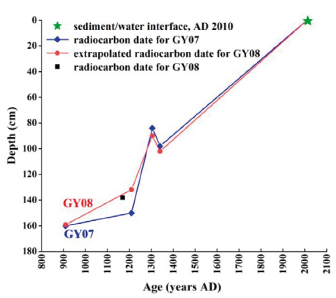
Figure 4 Age-depth model for the core GY08 and core GY07 from Gaoyou Lake sediments. Data of core GY07 were quoted from Li et al., 2015
Results
Core lithology and chronology
The lithology of GY08 has obvious layers, which may resulted from the changing paleosedimentary environment, paleoclimate and different material components. The upper 50 cm of GY08 was composed of brown and grey mud, with plant remains at the top 0-15 cm. The 50-100 cm tier had a grey and brown mud layer, and had horizontal-wavy bedding at the 80-90 cm tier. The 100-137 cm tier was dark grey to grey clay with a lot of shells and other organic fragments at the bottom of the segment. The 137-158 cm tier was grayish black and dark brownish clay, containing rich brownish organic matter and humus. Figure 3 showed the obvious stratigraphic similarities between cores GY08 and GY07. Core GY07 consisted of brownish-grey mud, grey clay, and dark brownish clay. The core showed wave and horizontal bedding at 85 cm, contained plant fragments on the top segment, and contained abundant shells at 144-161 cm. Both cores had the dark organic layer at the bottom (137158 cm at GY08, 144-161 cm at GY07), marked as a characteristic layer for corresponding relation between the two cores.
Li et al. (2015) presented an age-depth model of the sediment sequence that was based on four AMS radiocarbon dates obtained for GY07 in 2013 (Table 1). To establish a more accurate chronology, two more samples (one from each GY07 and GY08) were used to supplement that AMS dating in 2020. Here, the chronology of GY08 was developed by extrapolating the calibrated radiocarbon dates from GY07 to GY08, which was corroborated by the date at 138 cm in GY08 (Fig. 4). Core GY08 was formed in the 1,100 year period from AD 900 to 2010, at an average sedimentation rate of 0.15 cm/yr. The sedimentation rate was especially high during AD 1200-1400. The increase of depositional rate was assumed to be related with the result of the course change of the Yellow River in AD 1194 which brought heavy sediment into Gaoyou Lake. The interpretation was also justified by the abrupt increasing of particle size and pollen concentration, as discussed in detail below.
Grain size
The sediment sequence of GY08 was dominated by silt, which had a mean content of 84.5%. The remainder consisted of clay, fine sand, and medium and coarse sand-the latter two found in very small amounts. The grain size data showed a relatively stable median value and variable coarsest value. The sand fraction fluctuated greatly, varying between 0 and 40.7% at an average of 6.2%. The mean grain size of the sediment samples ranged from 4.4 to 37.2 um, with an average of 12.5 um. The value of sorting varied between 2.1 and 7.3, with a mean value of 3.6. The value of skewness changed from -0.5 to 0.6, averaging -0.1 while kurtosis oscillated between 2.3 and 0.8, with an average of 1.1(Table 2). The results indicate that the particle sorting was poor and finer relatively. The sediment distribution was asymmetric which shows that the samples may be combined from multiple sources. According to the results of the grain size analysis, suspended loads are the main component of sediments in Gaoyou Lake, whereas strong inflow occurred constantly. Figure 5 showed several obvious variations of most parameters at depths around 100-135 cm, 65 cm, and 20 cm indicating fluctuant sedimentary environment.
Table 2 The maximum (Max), minimum (Min) and average values of clay (%), silt (%), sand (%), mean (um), sorting, skewness and kurtosis of GY08

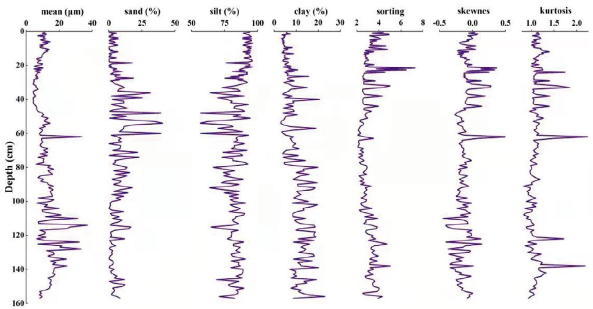
Figure 5 Mean (um), clay (%), silt (%), sand (%), sorting, skewness and kurtosis of GY08, based on the grain-size data
Pollen record
A total of 96 pollen and spore taxa were identified. To interpret the pollen spectra, all pollen types were classified into five eco-groups according to the life form of their parent plants. These eco-groups included tree and shrub pollen (including Pinus, Quercus, Cyclobalanopsis, Ulmus, Trema, Celtis, Liquidambar, Salix, Carpinus, and Pterocarya); terrestrial herb pollen (including Poaceae, Asteraceae, Chenopodioideae, Cruciferae, Artemisia, Polygonum, Fagopyrum, Plantago, Sesamum indicum, and Zea mays); aquatic pollen (including Typha and Cyperaceae); fern spores (including Selaginella, Hicriopteris, Pteris, Dicranopteris, Ceratopteris); and algae (including Pediastrum and Concentricystes). The photographs of the main pollen, spores, and algae in core GY08 are presented in Figure S1. Overall, terrestrial herb and tree & shrub pollen were the dominant pollen types in the entire profile with an average percentage of 46.5% and 34.8%, respectively.
The pollen data are shown in Figure 6. Based on the changes of pollen types, percentages and concentrations of the major taxa, and CONISS results, the pollen spectra can be divided into four pollen assemblage zones. The lowest pollen zone (Phase A, 158-100 cm) is characterized by the lowest percentage of tree and shrub pollen with the average value of 31.2%. Out of the 27 trees and shrubs pollen taxa identified, only 14 taxa were present in Phase A including Pinus, Quercus, Cyclobalanopsis, and Ulmus. Terrestrial herb pollen dominated with an average percentage of 50.3% and consisted mainly of Chenopodioideae (4.12%) and Artemisia (3.45%). Among fern spores, Ceratopteris reached its highest percentage in this zone. Pollen concentrations ranged from 26 to 195 grains/drop, with an average concentration of 58.3 grains/drop.
Phase B (100-45 cm) was notable for a clear increase in pollen contents for most taxa. The contents of aquatic pollen (12.3%) and fern spores (11.0%) significantly increased compared to the entire core. Several pollen types first appeared in this zone, such as Zea mays, Sesamum indicum, Liquidambar, Salix, and Pediastrum. Herb decreases to 39.0%, but they were still the dominant pollen. Pollen concentrations varied from 27 to 176 grains/drop, with an average concentration of 57.9 grains/drop.
The remarkable characteristic of Phase C (45-20 cm) was the terrestrial herbs pollen percentages increase to their highest average value of 51.3% at the expense of the fern spore percentages (6.6%). Pediastrum disappeared in this zone. The percentage of Cyperaceae decreased to its minimum of 2%, while the percentages of Artemisia (10.4%) and Chenopodioideae (6.7%) increased to their peak values over the whole core. The average pollen concentration was at the lowest value.
Herbs were still the dominant pollen in Phase D (20-0 cm) with percentages slightly decreasing to 49.7% compared to Phase C. Tree and shrub pollen percentages increased to 37.3% with relatively high content of Quercus (2.7%), while the percentage of Ulmus dropped to 2%. The percentages of fern spores and algae both increased in comparison to Phase C, with the average values being 8.1% and 0.8%, respectively. The average pollen concentration increased to the highest value.
PCA analysis
The result showed that Cyperaceae, Polygonum, Typha, Ceratopteris and other algae adapting to wet environments tend to spread in the positive values of the first axis, while Artemisia, Chenopodioideae and Asteraceae-which prefer an arid environment-were distributed towards the opposite direction. This indicated that the first principal component mainly reflected the changes in humidity, with the samples on axis 1 showing positive values related to wet conditions and negative values related to dry conditions (Fig. 7).
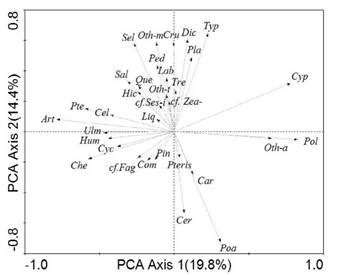
Figure 7 Results of PCA analysis in the pollen record of core GY08. Liq(Liquidambar); Hic(Hicriopteris); Sal(Salix); Que(Quercus); Ses-i(Sesamum indicum); Oth-t(Other trilete spore); Oth-m(Other monolete spore); Sel(Selaginella); Ped(Pediastrum); Lab(Labiatae); Cru(Cruciferae); Dic(Dicranopteris); Typ(Typha); Pla(Plantago); Zea-(Zea mays); Tre(Trema); Cel(Celtis); Pte(Pterocarya); Art(Artemisia); Ulm(Ulmus); Hum(Humulus); Cyc(Cyclobalanopsis); Che(Chenopodioideae); Fag(Fagopyrum); Com(Compositae); Pin(Pinus); Pteris(Pteris); Cer(Ceratopteris); Car(Carpinus); Poa(Poaceae); Oth-a(Other Algae); Pol(Polygonum); Cyp(Cyperaceae)
Taxa such as Dicranopteris, Cruciferae, Selaginella, Labiatae, Sesamum, and Pediastrum were influenced strongly by human agricultural activity (Li et al., 2008; Xie et al., 2008). They were mainly distributed at positive values along the second axis, implying that the second axis may represent the intensity of human activity. The PCA of the pollen assemblages of the Luonan Basin also showed that the first axis reflected the variation in humidity and the second axis may relate to human activity (Zhang et al., 2014).
Discussion
Proxy interpretation and discussion
The assessment of lacustrine sediment lithology may provide a clue to reconstruct lake levels and infer environmental conditions. A highly variable local environment was suggested by rapidly changing lithology. In terms of sediment transport dynamics, the presence of coarse grains in the lithology may reflect climate change or results of mass movements (Kliem et al., 2013). Moreover and most important, the sedimentation lithology offers the detection of flood deposits, which are marked by a high content of soil organic matter and appear as dark-brown layers that can be easily differentiated from the background (Simonneau et al., 2013; Zhang et al., 2015).
Particle size analysis has been used as a well utilized tool for reconstructing paleo-sedimentary environments based on grain size parameters such as mean diameter, sorting, skewness and kurtosis (Folk and ward, 1957; Folk, 1974; Sun et al., 2021). Grain size of the lacustrine sediment is related to hydraulic processes associated with transporting, sorting and deposition (Guo et al., 2021). The particle size chiefly depends on the hydrodynamic energy of the lake water, which is strongly linked to water level, energy of the input, and the source domains (Li et al., 2009). On one hand, grain size can be regarded as a lake level index that is sensitive to regional hydrological conditions. Previous studies (Chen et al., 2003) show that during wet periods, water levels rise and lakes expand. Hence, the distance between the lake shore and coring site would increase. It is more difficult for coarse particles to reach the core site, which leads to a decrease in grain size. Conversely, during dry periods, coarse sediments can more easily reach the coring site due to the diminished lake area. As a result, the mean value of particle size increases. On the other hand, transport ability of runoff was important factor that controls the grain size of sediment materials. The change of sediment source, intensity of erosion, human activities could affect the particle size by modifying the runoff transport. Thus, grain size has been widely used in the studies to trace and separate material sources (Ghilardi et al, 2008; Jia et al, 2012; Sun et al., 2015).
Identification of the sources of the pollen types is essential for interpretation of the pollen signal and reconstruction of palaeovegetation. The evolution of Gaoyou Lake is affected by the interaction of the river-lake system, thus the pollen of the lacustrine sediments may have multiple sources. The waterborne pollen by the inflowing rivers may also make a substantial contribution to the pollen influx to the lake. Thus, pollen in alluvium originates from both the realm of the rivers and the lake itself. Former studies of alluvial pollen on the North China Plain demonstrated that most of the arboreal pollen which derived from mountain trees, such as Pinus, Picea, Quercus, Betula, Tilia and Castanea, were transported by rivers from the mountains to the plain. By contrast, the appearance of Salix, Ulmus, Populus and Ailanthus, which were treated as plain trees, may derived from the plain itself. Most of the nonarboreal pollen were derived from the plain around the lakes. High percentages of pinus pollen can be used as an indicator of flooding because the larger and heavier pollen grains were mostly deposited by flood. Artemisia and Chenopodioideae pollen percentages mainly have close relations with ecological regions (Xu et al. , 2007). Cyperaceae pollen has a strong correlation with plant cover.
Vegetation and climate reconstruction using pollen records are often based on the presence and percentage abundance of selected indicator taxa (Herzschuh et al., 2009). Artemisia spp. and Chenopodioideae are widespread in temperate regions of the Northern Hemisphere. They are common in temperate steppes, meadows, and uplands especially in dry areas (Zhao et al., 2021). Thus, increases in their pollen percentages may indicate climate changes in more arid conditions. Pediastrum spp. is green algae common in freshwater and lives in shallow ponds, lakes, or small rivers. When Pediastrum increases, it suggests a warm and humid climate, or increased nutrients in the lake system (Tong et al. , 2009). Pollen assemblages are the proxies commonly used in paleoclimate reconstruction to identify human influences on the environment. Detecting human impacts via pollen analysis is based not only on abrupt changes in pollen concentration and richness, but also by the occurrence of cereal-type and cropland weed pollen (Li et al., 2008). Cereal-type pollen has been used as an ideal indicator of human activity. The occurrence and increase of Cereal-type pollen such as rice, corn, and wheat in sediments is direct evidence of an agricultural area (Djamali et al., 2009). Poaceae pollen is ubiquitous and widely used as a paleoclimatic indicator for increased regional aridity. The duration and intensity of human occupation are also variables affecting the percentages of Poaceae pollen (Bush, 2002).
Lake evolution and environmental reconstruction
A synopsis of the interpretation of grain size and pollen combined with core lithology results in the following most probable scenario of the paleoenvironmental history of Gaoyou Lake.
During stage A (158-100 cm; AD 900-1300), the percentages of tree and shrub pollen were the lowest of all phases. Terrestrial herbs such as Artemisia, Asteraceae, and Chenopodioideae showed relatively higher percentages than the average values of the whole core, indicating a drier climate. In addition, the low humidity was implied by negative PCA-1 scores. The particle size data from Gaoyou Lake were characterized by marked fluctuations. Based on the principle of sediment sorting, the high and greatly changed coarse component suggested a low and fluctuating lake level. The synthesis of the proxies indicated low water level and unstable climate during the deposition of this layer. This relatively dry interval can be attributed to an arid situation in the southern part of eastern China during the MCA period. The results were in agreement with previous studies (Zheng et al., 2006; Mann et al., 2009). Ge et al. (2014) also found the dry period to occur during the Northern Song, Southern Song, and Yuan Dynasties (AD 960-1368) in eastern China.
The lithology of the bottom part of this layer consisted of dark brownish clay with a large amount of organic matter and humus. Previous studies demonstrated that the color of the alluvial sediments appeared brown, which is a notable characteristic thereof, and a layer with abundant biogenic debris is a signal of flood events (Sirocko et al., 2013). According to historical documents, the Yellow River battered its banks and began to shift southward, encroaching the Huai River in AD 1128, due to human disturbance for military purposes (Xu, 1993). As a result, the Yellow River took over the lower channel of the Huai River heading for the Yellow Sea in AD 1194 (Fig. 8). Since then, a huge sediment load from the Yellow River has entered Gaoyou Lake. The change of material sources also led to the change of pollen concentration. Consistent with the result, Figure 5 and Figure 6 showed the increase in sand content and pollen concentration around AD 1200. Former researchers found that suspended sand increases the buoyancy of the river water. Due to the high sand content of the water from the Yellow River, it contains more pollen (Xu et al., 1996).
1. The channel after the major course change in AD 1048; 2. The channel after the major course change in AD 1194; 3. The channel after the major course change in AD 1494; 4. The channel after the major course change in AD 1855
Stage B (100-45 cm; AD 1300-1650) was separated from the previous zone by the clear increase in the proportion of aquatic pollen, accompanied by the decreasing value of mean particle size, both indicating that climate conditions were more humid. Positive PCA-1 denoted that the climate became wetter at this stage. It was consistent with previous studies that relatively warm and humid climate prevailed in North Jiangsu before the end of 16th century (Chen et al., 1998). Historical documents recorded 91 heavy floods during the Yuan and Ming Dynasties (AD 1279-1643) (Yang and Wang, 1995).
The presence of Cereal-type pollen such as Zea mays (65 cm, around AD 1530) and Sesamum indicum (90 cm, around AD 1340) is important as it may mark the expansion of agricultural activities during this period. Productive American crops such as maize were introduced and popularized in China at the beginning of the sixteenth century, which was confirmed by the peak in maize pollen percentage at 65 cm (around AD 1530). The earliest recorded planting of maize in China can be found during AD 1511 in "Ying State Records", the neighboring Anhui Province. The record of maize cultivation in the Gaoyou Lake Basin first appeared in the "Xinghua County Annals" in AD 1559 (Zhang, 1990).
Few pine species are found in the modern vegetation of the lake basin. Consequently, Pinus pollen mainly should be an alien component transported to Gaoyou Lake. In this study area, fluvial flow is more capable than wind to transport large quantities of exotic pollen over long distances, especially for Pinus pollen because of its larger size. As a result, increase of Pinus spp. content suggested that the enhanced fluvial flow or the regional climate turned wet (Jiang et al., 2013). The high Pinus percentage of this stage indicated a more exogenous input to the lake. Since AD 1194, the Yellow River has encroached the Huai River Basin. As a result, huge quantities of water with high sediment load from the Yellow River have poured into the lower reaches of the Huai River, leading to a rapid expansion of Gaoyou Lake (Xu, 1993). Finally, the lake enlarged gradually and amalgamated to form the present Gaoyou Lake around AD 1600 (Liao, 1992).
Stage C (45-20 cm; AD 1650-1850) was marked by an upsurge in terrestrial herb taxa, especially dry-tolerant plants such as Artemisia, Asteraceae and Chenopodioideae. The negative PCA-1 scores, extreme low percentages of aquatics, and absence of algae indicated further drying during this stage that was apparently connected with the LIA. Previous research demonstrated that the LIA was characterized by a cold and dry climate throughout China from the late 16th century until the end of the 19th century, especially in the mid-seventeenth century (Zhu, 1973; Chen et al., 1998).
The abrupt decrease of mean particle size and increase of sand content at 20 cm in the core (dated around AD 1860) was prominent in this stage. It coincided with the decrease in percentages of several types of tree pollen, such as Quercus, Ulmus, Celtis, and Pinus. This transition may be due to the northward shift of the Yellow River in AD 1855. Historical documents showed that the Yellow River broke its northern bank at Tongwaxiang and changed to a northeast course into the Bohai Sea in AD 1855 (Fig. 8), ending a 700-year long occupation of the Huai River (Xu, 1993). Thus, proportions of coarse particles dropped sharply. The reduced stream inflow may have led to the decreased percentage of exotic tree pollens.
Stage D (20-0 cm; AD 1850-2010) was separated from the preceding zone by the notably decreasing value of the mean particle size and the most positive PCA-1 scores, suggesting a more humid period. The decreased herb pollen percentages as well as the increased proportions of fern spores and algae also confirmed this inference. The anomalous warming over China in the 20th century is well known (Yang et al., 2002). Previous studies also reported that moister conditions in China occurred since 1890 (Peng et al., 2009).
The most conspicuous feature of this stage was the appreciable increase in anthropogenic herbs, such as Fagopyrum and Sesamum indicum, indicating intensified cereal cultivation. The high amounts of Poaceae and Cruciferae were also evidence of human disturbance (Li et al., 2008). The rise of Pediastrum is interpreted to be related to increased nutrients in the lake. It is documented that lake reclamation in the Gaoyou Lake area started in 1857 (Wang and Yang-1990). In 1988, the area of Gaoyou Lake shrank by 25% compared to the 1950s. The population had almost doubled during the four decades from the 1950s to the 1990s (Li et al., 2011). As a result, water quality has degraded since the 1990s with accelerated urbanization.
Conclusion
This study indicated that the main component of the sediments in Core GY08 was silt. Terrestrial herbs were the dominant pollen in the entire profile of core GY08.
Pollen assemblages, sediment grain size and lithology of Core GY08 recorded four main phases in the climatic and hydrological changes of Gaoyou Lake over the last millennium. During AD 900-1300, the climate was dry, and Gaoyou Lake experienced a stage of low water levels. The lake began to expand under more humid conditions after AD 1300. Subsequently, the water levels dropped because of the dry climate during AD 1650-1850 in conjunction with the LIA. After AD 1850, a wet climate prevailed in the study area.
Major regional events such as course changes of the Yellow River during AD 1194 and AD 1855 were reflected in abrupt variations of sediment characteristics and the changing percentage of river-transported pollen from exotic plants. The first appearance of maize pollen during AD 1560 as well as the increase in pollen of plants associated with human activities in the modern era reflected a widespread human interference with the natural vegetation and an intense cultivation of the lake area.