1. Introduction
Inadequate hands washing and ineffective surfaces disinfection contribute to microorganisms spreading, giving rise to foodborne illness (Flórez, Rincón, Garzón,Vargas & Enríquez, 2008; Mkhungo, Bamikole & Ademola, 2018; Jansen et. al. 2019). Concomitantly, in recent decades, food origin outbreaks due to emerging multi-resistant strains are increasing (White, Zhao, Simjee, Wagner & Mcdermott, 2002; Azevedo, Albano, Silva & Teixeira, 2015; Friedman, 2015). Resistant strains are no longer exclusively acquired in hospital environments, but also in restaurants, education centers and homes (Langiano, Ferrara, Lanni, Viscardi & Abbatecola, 2012). Environmental safety studies report that inert surfaces, clothing, and cooking utensils are sources of transmission of potentially pathogenic microorganisms (Escobedo, Meneses & Castro, 2016; Caro-Hernández & Tobar, 2020). Most of the pathogenic bacterial communities can form biofilms (Srey, Jahid & Ha, 2013), that act as a shield against the action of antimicrobial substances, allowing microorganisms to persist for long periods in specific surfaces (Capita & Alonso-Calleja, 2013; Stewart, 2002). Added to this is the misuse of antimicrobial agents such as biocides, which exert selective pressure, increasing the problem (Friedman, 2015). There is a proven correlation between antibiotic resistance and biocidal resistance, indicating that bacteria that develop antibiotic resistance can also have cross resistance to some disinfectant types (Rutala, Stiegel, Sarubbi, & Weber,1997; Pal, Bengtsson-Palme, Kristiansson, & Larsson, 2015).
Having a better knowledge about the routes of dissemination of antimicrobial resistance, promoting the control of resistant bacteria in non-hospital environments and optimizing the use of disinfectants is very important to contribute to the mitigation of bacterial resistance. Therefore, the purpose of this study was to evaluate the resistance profile to antibiotics and commonly used disinfectants, in bacteria isolated from surfaces of formal and informal restaurants, and in this way, to be able to transfer more precise information to food handlers. In order to correct disinfection practices and acquire a deeper understanding of the problem.
2. Materials and methods
Origin of isolated bacteria
Isolates were previously obtained in a study conducted by the research group Microambiente Libre from the Faculty of Health Sciences, Universidad Libre, Cali (Caro-Hernández & Tobar 2020). In this study, samples from living surfaces (operator's hands) and inert surfaces (preparation surfaces, kitchen utensils and dispensers) were processed for microbiological isolation and identified by rapid biochemical tests, obtaining 52 isolates of which, 17 were selected for the present study (See Table 1).
Table 1 Evaluated bacterial isolates
Bacteria | Code* |
---|---|
Enterobacter agglomerans | M1C1 |
Enterobacter cloacae | M2C2 |
Enterobacter cloacae | M6C8 |
Enterobacter cloacae | M3C4 |
Serratia liquefaciens | M3C5 |
Escherichia coli | M12C13 |
Enterobacter agglomerans | M15C23 |
Enterobacter agglomerans | M15C24 |
Serratia liquefaciens | M17C29 |
Klebsiella oxytoca | M8C42 |
Aerococcus urinae | M2C3 |
Kocuria kristinae | M7C8 |
Kocuria kristinae | M8C13 |
Kytococcus sedentarius | M12C18 |
Staphylococcus warneri | M1C35 |
Staphylococcus heamolyticus | M1C36 |
Kocuria kristinae | M6C41 |
*Strain bank reference code Environmental Microbiology Laboratory, Universidad Libre Seccional Cali
Source: Produced by the authors
Maintenance and bacterial reconstitution
Bacterial isolates were kept at -81 ° C in a 10% glycerol solution, until further use. For reconstitution, isolates were inoculated in 5 mL Tryptic Soy Broth (TSB) (Merck Darmstadt Germany) and incubated at 32 ± 2 ° C with constant stirring for 12-24 h. Subsequently, colonies were isolated using the depletion method in Tryptic Soy AAgar medium (TSA) (Merck Darmstadt Germany), and incubated for 12-24 h at 32 ± 2 ° C. To verify colony purity, Gram staining was performed at the start of each test.
Antibiotic susceptibility assessment
The 17 identified isolates were exposed to different antibiotics following the Kirby-Bauer method, described in the Clinical and Laboratory Standards Institute (Clinical and Laboratory Standards Institute (CLSI), 2013). Briefly, from a fresh culture isolate, a colony was taken and inoculated in 5 mL of 0.9% saline solution, until turbidity reached 0.5 in the McFarland scale. From the bacterial suspension a sample, by triplicate, was plated on Petri dishes containing 20 mL of Mueller Hinton Agar (Merck Darmstadt Germany). Four BD BBL antimicrobial susceptibility Test Discs (Becton Dickinson USA) with different antibiotics were placed on the surface of the Petri dishes. For Gram negative bacteria, Sulfamethoxazole / Trimethoprim (SXT) (1.25 Mg), Ciprofloxacin (CIP) (5 Mg), Kanamycin (K) (30 Mg), Gentamicin (GN) (10 Mg), Ceftriaxone (CRO) (30 Mg), Annamycin (AN) (30 Mg), Tetracycline (TE) (30 Mg), Ceftazidime (CAZ) (30 Mg) were tested. For Gram positive bacteria, Kanamycin (K) (30 Mg), Ciprofloxacin (CIP) (5 Mg), Ceftriaxone (CRO) (30 Mg), Rifampicin (5 Mg), Tetracycline (TE) (30 Mg) and Vancomycin (30 Mg) were used. Cultures exposed to antibiotics were incubated for 24 h at 32 ± 2°C. The tested bacteria were considered resistant, susceptible or of intermediate resistance, according to the cut-off values recommended by the CLSI and the antibiotic normalization values by the Kirby-Bauer technique (Annexed 1) (Bernal & Guzmán, 1984).
Evaluating resistance to various disinfectants Inoculum preparation
To begin with a known inoculum, an optical density (OD200nm) growth curve was constructed by measuring at different time intervals until reaching the late logarithmic phase. Taking these data into account, inoculum preparation was standardized as described in Radcliffe et al. (2004). For this, each of the isolates was plated in TSA by depletion. A single colony selected from each culture was inoculated in 5mL TBS and incubated overnight. An aliquot of 200 ML was taken from each overnight culture and inoculated onto 19.8 mL of TSB, followed by incubation at 32 ± 2 ° C under constant stirring, during 1.5 to 4.5 h, depending on the isolated (Radcliffe et al.. 2004).
Minimum inhibitory concentration test
Bacterial isolates were evaluated for the minimum inhibitory concentration (MIC) for each disinfectant. For this, five tubes with a final volume of 10 mL at different concentrations of the commercial disinfectant (% v / v), were inoculated with 200 ML of the late logarithmic phase culture. The concentrations used for Sodium Hypochlorite (NaOCl) were 0.2%, 0.4%, 0.6%, 0.8% and 1% and those used for Acetic Acid (CH3COOH) were 0.10%, 0.12%, 0.13%, 0.14%, 0.15 %. Bacteria that exhibited resistance to previous concentrations of CH3COOH were also tasted using higher concentrations (0.5% and 1%). All suspensions containing disinfectants and bacteria were incubated for 18 h at 32 ± 2 °C with constant stirring.
Disinfectant susceptibility test
From the isolates that presented some level of antibiotic resistance, susceptibility to commercial disinfectants was evaluated, based on the method of Liao, Shollenberger, Phillips (2003). Briefly, 1 mL of late logarithmic phase culture was transferred to Eppendorf® tubes, then centrifuged at 12000 rpm. After the supernatant was removed from all tubes, each pellet was diluted in a disinfectant solution (0.9% peptone water - disinfectant) and measured at pre-established exposure times (l, 5, 10, 15, 20 and 25 min) (Liao et al 2003). The concentration used for each disinfectant solution (NaOCl and CH3COOH) was based on the previous MIC tests. All tests were done by triplicate. One milliliter of each suspension (bacterial inoculum-peptone water-disinfectant) was washed and centrifuged (l2000 rpm / l min) twice in order to inactivate disinfection. The final pellet obtained from the test was suspended in 1 mL PBS buffer (75 MM, pH 7.2) followed by serial dilutions, 10-2, 10-4 and 10-2. Each dilution was plated by duplicate using 100 ML, followed by 24-48 hours incubation at 32 ± 2°C.
Calculation of survival and death rate
The survival and death rate of the evaluated bacteria were determined following the protocol described by Ray (1979), where the survival rate was calculated using the following equation:
Were:
%S: survival rate.
ncp:plate count number (CFU / mL) after treatment with disinfectant. NCP:plate count number (CFU / mL) before treatment without disinfectant
(Ray, 1979).
Tetracycline gene detection in resistant isolates
Tetracycline gene detection was performed by means of PCR amplification, using the reference primers described in Ng, Martin, Alfa & Mulvey (2001).The selected primers were grouped into three classes: associated with efflux pumps tet (A), tet (B), tet (C), tet (D) and tet (E), associated with ribosomal protection or tet efflux mechanisms (L), tet (M), tet (O) and tet (S) and associated with enzymatic modification tet (X) (Annexed 2). PCR amplification was carried out according to the protocol described by Ng et al. (2001), and following the GoTaq® Green master Mix (Promega Madison Wisconsin USA) manufacturer instructions with 100 ng of DNA as a template. Sequences and annealing temperature of the used primers are shown in Annexed 2. The PCR products were visualized by electrophoresis in 1.5% agarose gels (w/v), and the amplicon sizes were verified by comparison with the 100-bp plus Thermo Fisher Scientific® ladder.
Data collection and statistical analysis
Survival and death percentages, obtained from the CFU/mL counts in susceptibility tests against NaOCl and CH3COOH disinfectants, were calculated for each concentration and contact time. A regression model was performed for each isolate, contrasting the dependent variable, the percentage of survival, against the independent variables. Statistical analysis was performed using the Rstudio® software packages (version 3.5.1).
3. Results
Susceptibility to antibiotics
Out of the 17 evaluated bacterial isolates against antibiotics, 10 belonged to this family, with the genus Enterobacter the most frequently found. All 17 isolates were sensitive to Gentamicin and Trimetropin-Sulfamethoxazole. In contrast, E agglomerans MICI, E cloacae M2C2, E cloacae M2C8, E cloacae M3C4 and S liquefaciens M3C5 isolates showed intermediate resistance against Ciprofloxacin (5 Mg), Kanamycin (30 Mg), Ceftriaxone (30 Mg), Anamicin (30 Mg) and Ceftazidime (30 Mg). It should be noted that E. coli M12C13 showed resistance against Ceftriazone (30 Mg) while the isolated E. cloacae M2C2, E cloacae M2C8, E. cloacae M3C4, and S. liquefaciens M3C5 showed resistance against Tetracycline (30 Mg). Although the majority of Gram positive isolates were sensitive to the evaluated antibiotics, A. urinae M2C3 showed resistance to Vancomycin, while Kocuria kristinae M7C8 and M8C13 exhibited resistance to both Ceftriaxone and Tetracycline. Resistance to antibiotics was evident in 9 out of the 17 isolates, with Tetracycline as the one to which most tested bacteria exhibited resistance, followed by Ceftriazone (See Table 2).
Tetracycline resistance gene detection
Tetracycline resistance, tet (M) gene, was amplified in E. cloacae M2C2, E cloacae M2C8, S. liquefaciens M3C5 and K kristinae M7C8 isolates, becoming the most frequently detected in all samples. Complementarily, tet (D) gene was amplified in S. liquefaciens M3C5, tet (E) gene in E cloacae M2C2 and M3C4, and tet (O) gene in K kristinae M7C8 isolate. It should be noted that in the E. cloacae M2C2, S. liquefaciens M3C5 and K. kristinae M7C8 isolates, more than one Tetracycline resistance gene was amplified. The remaining evaluated genes [tet (A), tet (B), tet (C), tet (L), tet (S) and tet (X)] were undetected in all the I isolates (Figure 1).
Minimal inhibitory concentration
Considering that a correlation between antibiotic resistance and disinfectant resistance has been previously found (Rutala et al. 1997; Pal et al. 2015; Khan, Beattie, Knapp, 2012), the resistance to two commonly used disinfectants (Sodium Hypochlorite and Acetic Acid) was evaluated in eight isolates that exhibit resistance to antibiotics. In order to establish at which concentration, the bacterial growth was inhibited, a MIC test was performed. Results summarized in Table 3 show that both Gram negative and Gram positive isolates were inhibited at a 0.4% NaOCl concentration. The subsequent analysis dealt with evaluating the germicidal efficacy of disinfectant concentration recommended by manufacturers, (0.2% NaOCl). In contrast, the isolates exposed to CH3COOH exhibited different MICs values, ranging between 0.12% to 0.16% in Gram Negative isolates and from 0.13% to 0.5% in Gram positive isolates, with the exception of K. kristinae M8C13 with a MIC value of 1%.
Disinfectants susceptibility test
It was possible to demonstrate that NaOCl [0.2%] is the disinfectant that in the shortest time can inhibit the growth of most of the isolates evaluated. Gram negative bacteria show to be the most susceptible, with 0% survival after the first minute of exposure. Although the inhibitory action of NaOCl can be confirmed, we demonstrate that manufacturers recommendations around exposure (10min) and concentration (0.2% v/v) required for bacterial inactivation, is not effective I in all cases. In this study, different isolates were exposed to the recommended concentration. As a result, bacteria such as A. urinae M2C3 and K. kristinae M8C13 managed to recover and grow after 10 min of exposure to the recommended dose, however, survival rates are low (I% and 2%, respectively) (See Table 4).
Table 4 Survival percentage of Gram positive and Gram negative bacteria against NaOCl [0.2%]
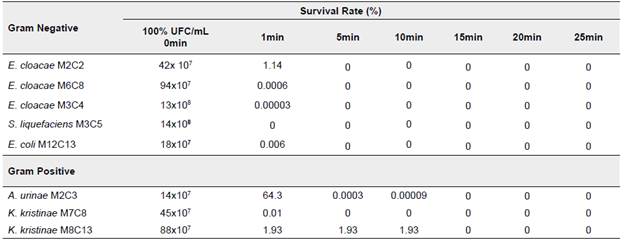
Source: Produced by the authors
Regarding CH3COOH, E. cloacae M2C2 showed the highest survival percentage (5.2%) in the MIC test, above all the other isolates, for up to 25 min of exposure, with a particularly high survival rate (84%) found after 15 min of exposure. E. cloacae M3C4 and E. cloacae M6C8 followed, showed a 4.8% and 4% survival rate after 25min of exposure. On the other hand, the least resistance isolate was E. coli M12C13 with a 0.2% survival rate after 25min of exposure (See Table 5).
Table 5 Survival percentage of Gram positive and Gram negative bacteria against CH3COOH
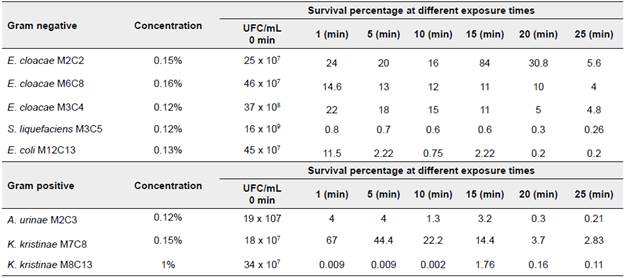
Source: Produced by the authors
Above Gram positive bacteria, K. kristinae M7C8 was the least susceptible, with a 44.4% survival rate after 5 min of exposure, meaning a 2.83% reduction in the survival rate after 25 min. Other Gram positive isolates like A. urinaeM2C3 and K. kristinae M8C13 showed 0.21% and 1.72% after 15 min of exposure. Based on previous results, the inhibitory capacity of disinfectant CH3COOH is low for most isolates, showing statistical significance in relation to exposure time (p<0.001).
4. Discussion
The pressure exerted by the inappropriate use of antimicrobial substances has led to the spread of bacterial resistance, even outside hospital facilities (Azevedo et al. 2015), increasing this public health problem (Friedman, 2015). A clear example of this are bacteria isolated from food or food contact surfaces, several of which belong to the Enterobacteriaceae family (Valdiviezo-Lugo, BettinaVillalobos, Martinez & Nazaret, 2006). It is well known that several species from this family have increased their resistance to antibiotics (Azevedo et al. 2015; Iredell, Brown & Tagg, 2015). Previously, we found that most of the bacteria isolated from food contact surfaces included in this study, belong to the Enterobacteriaceae family (Caro-Hernández & Tobar 2020). The current study shows that most of the obtained isolates (47%) exhibit some degree of resistance to antimicrobial agents, and majority of these isolates are resistant to more than one antibiotic. Our results are consistent with those obtained by Azevedo et al. (2015), where 49.2% of bacteria from the Enterobacteriaceae family, collected from surfaces such as cutting boards, cutlery, dishwashers, oven knobs, etc., showed resistance to at least one antibiotic (Ampicillin, Nitrofurantoin, Tetracycline, Nalidixic Acid, Chloramphenicol and Trimetropin) (Azevedo et al. 2015). The presence of this type of bacteria on the sampled surfaces is possibly due to cross contamination and poor hygiene practices by food handlers, which have become the most important spreading vector for contamination. Although members of the antibiotic-resistant Enterobacteriaceae family are generally found in hospital settings (Marques Di Primo et al. 2017), there is evidence that the same family, containing antibiotic resistance genes, can be found in surrounded areas outside hospitals (Azevedo et al. 2015). The most important reservoir of these bacteria is the gastrointestinal tract of human and farm animals, which can be treated with sup-therapeutic concentrations of antibiotics that consequently generate resistance in these bacteria. The water, food and environmental contamination with resistant bacteria is an important way for their diffusion, therefore, becoming a crucial control area (Pitout, Nordmann, Lauplad & Poirel, 2005; Li, Chang, Zhang, Hu & Wang, 2019).
Most of the evaluated isolates (35.3%) showed Tetracycline resistance. This antibiotic has been extensively used at a therapeutic level (Roberts,1992; Villedieu et al. 2003). In addition, its sub-therapeutic use in different countries, as a food additive to promote the growth of animals, has substantially increased the resistance to this antibiotic (Jara, 2007; Barton, 2014; Seifi, & Khoshbakht, 2012), leading to health emergencies due to the decreased antibiotic efficacy (Chopra & Roberts, 2001). Likewise, Ceftriaxone, for which 17.2% of the evaluated isolates showed resistance to, is one of the most widely prescribed antibiotics for different types of infections, and there is evidence that it is one of the antibiotics to which more genera of the Enterobacteriaceae family show resistance (Goldstein et al.1993; Velickovic-Radovanovic, et al. 2015). In the case of Vancomycin, only Aerococcus urinaeM2C3 showed a clear resistance. Resistance to Vancomycin has been reported, especially for Enterococcus spp. (Arias, & Murray, 2012; Gousia, Economou, Bozidis & Papadopoulou, 2015) and recently in specific strains of Staphylococcus aureus, which is alarming since it has been a frequently prescribed antibiotic in the treatment of serious infections caused by S. aureus strains that are resistant to Methicillin (Velásquez-Meza, 2005).
When evaluating Tetracycline resistance genes, we could confirm that those bacteria that are resistant to more than one type of antibiotic, have different Tetracycline resistance g enes. In this study, the tet (M) gene, associated with both, efflux pumps and ribosomal protection (Ng et al. 2001 ; Roberts, 1992; Jara, 2007), was more frequently found among E. cloacae M2C2 and M2C8, S. liquefaciens M3C5 and K kristinae M8C13 isolates, which is confirmed by several authors who affirm that the tet(M) gene has a wide distribution, and can be detected in both, Gram positive and Gram negative bacteria. This is due to tet (M) gene association with frequently conjugated transposons (Doherty, Trzcinski, Pickerill, Dowson, & Zawadzki, 2000; Meygret, Le Roy, Renaudin, Bébéar, & Pereyre, 2018). On the other hand, the tet (D) and tet (E) genes, which are known to be associated with efflux pumps, were detected exclusively in Gram negative bacteria (S. liquefaciens M3C5, E. cloacae M2C2 and M3C4), which confirms what has already been stated by other authors: tet (D) and tet (E) genes are only found in this group of bacteria (Jara, 2007; Hedayatianfard, Akhlaghi, & Sharifiyazdi, 2014). Finally, the tet (O) gene, which has a wide distribution in different Gram-positive bacteria, located in conjugative plasmids (Roberts,1992), was detected in K kristinae M7C8.
Regarding the susceptibility to disinfectants, the results showed that despite the disinfectant efficiency of NaOCl, there are bacteria capable of resisting, so it is important to reconsider the concentration used in each case. Inappropriate use and poor control of this type of disinfectant can result in an increase of bacterial resistance to the compound (Sommer, Munck, Toft-Kehler & Andersson, 2017), and even leads to the activation of resistance factors or the generation of mutations that can be later horizontally transferred. NaOCl is one of the most widely used disinfectants worldwide, which makes it a good candidate for disinfection studies. Rutala, Berbee, Aguilar, Sobsey & Weber (2000) demonstrated its high level of action as a household disinfectant against different isolates (Rutala et al. 2000). Similarly, other studies showed that NaOCL has good efficacy, although some of the exposed isolates have a high level of resistance (Radcliffe et al. 2004; Rutala et al. 2000). However, it should be considered that this compound is a bacteriostatic element, since it acts by inhibiting the nucleic acids synthesis (Davin-Regli, & Pagés, 2012), explaining why sometimes the effectiveness of the compound is diminished.
On the contrary, the survival rates for different evaluated isolates confirm the poor disinfectant efficacy of CH3COOH. During this study it was observed that some of the isolates sensitive to NaOCl were resistant to CH3COOH. It is important to highlight that most of the tested bacteria were resistant to the concentration recommended by the manufacturer (0.12%), up until the maximum exposure time. Based on these results, we can assure that when using the manufacturer recommendations towards exposure and concentration in food preparation areas, this disinfectant must be intercalated or mixed with complementary disinfectants to improve its action. It is important to pinpoint that resistance to the compound is not well understood yet (Russell,1991; Liao et al. 2003) and multiple factors, such as: a) prevention of the CH3COOH entry to the bacteria due to the optimization of the lipid ratio. b) assimilation of the compound due to the ability to convert acetic acid into an alternative energy source during the Krebs cycle. c) transport by efflux or efflux pumps through two intracellular discharge systems and d) protection by cytoplasmic proteins, an environmental adaptation induced by chaperones that stabilize internal proteins from damage (Russell, 1991; Nakano & Ebisuya, 2016), must be considered. The low efficacy of CH3COOH has been observed in different studies. Liao et al. (2003), showed that their action on Salmonella spp., cannot be exclusively affected by the concentration or the exposure, but also by the age of the isolate, being the stationary phase wherein bacteria show greater resistance. In addition, the same author confirms that its action improves if it is used in combination with Hydrogen Peroxide (Liao et al. 2003). Rutala et al. (2000) evaluated CH3COOH, along with other commonly used disinfectants, demonstrating that the inhibitory action was low against bacteria of the Enterobacteriaceae family, compared to other disinfectants (TBQ, Vesphene IIse, ethanol, Sodium Bicarbonate...) (Rutala et al. 2000).
Finally, our results confirm what was stated by other authors, showing that when isolates show resistance against some type of antibiotic, they can also exhibit resistance against some type of disinfectant or vice versa (Townsend, Ashdown, Greed & Grubb,1984; Pal et al. 2015; Khan et al. 2012). This assumption can be clearly observed in K kristinae M8C13, which showed resistance to Ceftriaxone and Tetracycline, the latter confirmed by the detection of the Tetracycline tet (M) gene and, to the two evaluated disinfectants. Resistance to both antibiotics and disinfectants may probably be due to resistance factors that are related or are somehow activated by the generated stress after chemical exposure to the compound. Khan et al. (2012) revealed a correlation between tolerance to chlorinated compounds and resistance to antibiotics such as Tetracycline, Sulfamethoxazole and Amoxicillin (Khan et al. 2012). More recently, Liu et al. (2018) observed that both extracellular and intracellular resistance genes (ermB, tetA, tetB, tetC, sul/, sul2, sul3, ampC aph(2')- Id, katG, vanA, tetA, tetB, tetM, tetQ: tetX, suU, sul2, sul3, ermB, blaTEM, and qnrA) may increase due to the exposure to chlorination (Liu et al. 2018). In addition, it is known that resistance mechanisms such as efflux pumps, permeability changes and biofilm formation are common elements against disinfectants and antibiotics (Abdallah, Benoliel, Drider, Dhulster & Eddine, 2014), which could explain the resistance of K kristinae M8C13, which It has been described as a biofilm-forming bacterium (Purty et al. 2013).
The evidence here gathered leads us to conclude that the selective pressure exerted by biocides can favor the expression and dissemination of resistance mechanisms, thereby, extending antimicrobial resistance, which represents a major problem in the fight against bacterial resistance to antibiotics.
5. Conclusions
This study shows that the highest percentage of antibiotic resistant isolates belongs to the Enterobacteriaceae family, highlighting the importance of food handlers as a source of dissemination for these microorganisms. Antibiotic resistance against Tetracycline found among most isolates, confirms the presence of antibiotic resistance genes, with tetM gene holding the highest frequency among all isolates. Likewise, it was noted that both E. cloacae M2C2 and S. liquefaciens M3C5 possess more than one antibiotic resistance gene. Disinfectant NaOCl was found as highly efficient for bacterial inactivation. However, the resistance showed by isolates A. urinae M2C3 and K. kristinae M8C13, after 10 min of exposure, leads to the thought that concentration recommended by commercial companies might not be sufficient in all cases. In contrast, CH3COOH did not show to be very effective as a disinfectant against most isolates, confirming the results supported by different authors, which show various resistance factors against this disinfectant. It was confirmed that K kristinae M8C13 has resistance to more than one antibiotic (ceftriaxone and tetracycline), and that it contains the tetracycline resistance gene tetM also showing resistance against the two disinfectants evaluated (NaOCl and CH3COOH), evidence that shows this isolate as the one with the highest resistance. The above-mentioned results could demonstrate the relationship that exists between antibiotic resistance and disinfectant resistance, a topic that needs to be explored in order to have a better understanding of the factors involved in the activation and transfer of bacterial resistance.