1. Introduction
In many applications, where voltage and current regulation are needed from unregulated sources such as battery charging and discharging, power factor correction, fuel cell regulation, maximum power tracking of solar panels, among others, step-up/ step-down converters are required [1] [2] [3] [4] [5] [6] [7] [8] [9].
Buck or boost converters have greater efficiencies in comparison with step-up/step-down DC-DC converters with a single active switch, such as buck-boost, fly back, Sepic and Cuk topologies, because their stresses are lower than these converters [8] [10]. In voltage regulation applications is widely used buck converters with a boost converter by means of two independently controllable switches [3] [4] [11] [12] [13]. These converters do not operate in buck-boost mode, because it is more efficient to work them in buck or boost mode, depending on their input and output voltages [2].
Most of the converters cited above have the following drawback: when they are working in boost mode, under continuous conduction mode (CCM), they have a right-half-plane (RHP) zero that complicates the design of their controller, limits their bandwidth of the loop an increases the output capacitor size [8] [11]. A solution to the problem of the RPH zeros in this research work is the use magnetic coupling between inductors with damping networks [14] [15] [16]. This solution has allowed the design of high-power converters with high efficiency and wide bandwidth [8] [9] [17] [18].
In distributed generation (DG) systems [19] and satellite power systems [20] [21] different DC-DC converters need to be designed in which the input or output current control depends on their position in the system. Proton exchange membrane fuel cell (PEMFC) systems are good candidates for supplying electrical power in DG systems [22] residential environments [23], electric vehicles [24] [25] and DC bus applications [26]. The PEM stack is considered slow because its dynamics are limited by the compressor that supplies the oxygen to the cathode. As a consequence of the dynamic response limitations, a load transient could cause a short duration large-voltage drop, which is characteristic of the oxygen starvation phenomenon that could be harmful for the PEMFC [27]. Prevention of this undesired phenomenon has been addressed using batteries, capacitors, or other Auxiliary Storage Devices (ASDs) to ensure a fast dynamic response to any load power transient.
These systems prevent the oxygen starvation phenomenon by limiting the slew-rate (SR) of the FC current by means of current-controlled DCDC converters.
This set of elements, known as a FC hybrid system, are needed to support the operation of the FC [28] [29] [30] [31]. The modular buck-boost converter used in these research works, whose schematic circuit is shown in Fig. 1, was proposed in [8] along with the current control proposed in [9] and improved in [32], where it has been input/output current regulated following classical rules [33] [34] [35] [36]. The FC topologies mentioned earlier have the zero crossing saturation problem in the low-level analog current controllers, which causes a potential instability of the dc bus voltage, with a duration of 1 or 2 ms. In the scope of these works, this issue was addressed at the expense of decreased total power conversion efficiency.
State-feedback control based on linear matrix inequalities provides a solution that ensures to comply with several number of dynamics constraints, unlike classical techniques [37]. LMI control technique uses modern numerical optimization methods to ensure the control requirements and thus to obtain the controller parameters [38]. Some authors have reported LMI controller for switching regulator [39]-[41]. This technique allows to ensure requirements on stability, a prescribed closed loop pole placement, and control effort. Thus, this work presents a LMI control for a non-inverting buck-boost converter which ensures stability and some dynamical constraints.
This works is organized as follows: Section II shows the small-signal converter model and LMI design expressions used in the control design. Matlab and PSIM simulations on an 800-W coupled-inductor buck-boost dc-dc switching converter are presented in Section III. Finally, the main conclusion of this work are summarized in Section IV.
2. Material and Methods
2.1 Analysis of the Proposed Converter
For studying the converter of Fig. 1 is assumed that the input and output voltages which correspond to vg and vo , respectively, are voltage sources such as FCs, batteries, supercapacitors and regulated DC buses. Assuming CCM, no parasitic effects and a switching frequency higher than the converter natural frequency and the AC variations are much smaller than the steady-state values, the system of differential equations can be linearized around the operating point to obtain the small-signal SSA model [42], which is shown as follows:
where and correspond to the small-signal state vector and AC variations of Buck and Boost stages duty cycles, respectively. Assuming that the converter is in steady-state with constant duty cycles d
1(t) = D
1 and d
2(t) = D
2, input voltage
vg
(t) =
Vg
, and output voltage
vo
(t) =
Vo
, the state matrix A and the input vectors B
1 and B
2 of the system are given by:
where Pg is the average input power given by Pg = Vg Ig .
The model can be augmented to ensure zero steady-state error in the input current ig (t) by introducing a new state variable x 6 corresponding to
where Igref is the input reference current. Thus, the augmented model can be written as:
where
In order to have a better understanding of the SSA model mentioned above, let us consider the following set of converter nominal parameters: Lm = 35 µH, C = 2.2 µF, Rd = 1.5 Ω, Cd = 22 µF, and L = 35 µH. The voltages and power levels are determined by the following ranges: 15 V < vg < 70 V, 0 V < vo < 70 V, 0 A < ig < 16 A, and 0 A < io < 16 A.
Once we have obtained the small-signal SSA model, different control schemes can be implemented to regulate the input or the output current of the proposed buckboost converter. We have selected a LMI-based state-feedback controller because it offers, among others, take into account pole placement constraints, control effort limitation, and decay rate and bandwidth improvement.
Additionally, the use of the SSA method allows to describe LMI constraints, which it can be computed automatically by a standard optimization algorithm [38].
2.2 LMI Control Design
In this section, we review brief concepts of LMI control that are next applied to buck-boost converter. Linear Matrix Inequalities has being used in control for long time [37], a classical control theorem establishes that the system Matlab [38]
is stable if all trajectories converge to zero, quadratically stable in Lyapunov sense, if and only if there exists a positive definite matrix P, such that
Hence the quadratic stability requirement has become a problem of solving a linear matrix inequality P. By means of a numerically simple test, it’s possible to check the overall stability of a dynamics system. This concept can also be extended to feedback law. Given the system
where is the input signal, for which we assume a linear feedback control A closed system is stable if there exists a P > 0, such that
However, the inequality (9) is nonlinear. So, it can be rewritten as:
where W = P -1, Y is defined so that F = YW -1. Thus, we can obtain the feedback gains F that stabilize the system (8) that fulfill with (10).
Other restrictions can be imposed on the feedback gain vector F to ensure an appropriate dynamic behavior in closed loop [39]. Another important constraint to be imposed to the systems dynamics is the pole placement [43]. We desire that the closed-loop poles have a minimum damping ratio and a minimum decay rate. Therefore, the constraints of decay rate and damping ratio are imposed by means of the following LMIs.
A control fulfilling with all previous restrictions but presenting an excessive gain F would by the duty cycle saturation, which would worsen the expected performances. So, we bound the control effort along the trajectory for any initial condition inside the ellipsoid by means of the following additional LMI
The proposed LMI control technique consists of solving inequalities (10)-(13) to find the matrix W and Y that satisfy all the previous constraints. Hence, the design procedure becomes a feasible problem that can be written as:
The solution of this feasible problem with its corresponding constraints will provide a feedback gain vector, i.e. F=YW -1. The solution of these LMIs can be readily solved by standard interior point methods using Matlab (38).
3. Results and Discussion
In this section, we presents an LMI control approach applied to the problem of regulating the input current of the coupled inductor buck-boost dc-dc switching converter, which is shown in the Fig. 1. It can operate in three different modes, depending on its input and output voltages: buck mode ( vg > vo ), buckboost mode ( vg = vo ) and boost mode ( vg < vo ).
To test the validity range of the small-signal SSA model obtained in equations 1 and 2, some specific simulations has been carried out and compared with the proposed converter Simulink model of Fig. 2(a) and the PSIM dc-dc converter circuit diagram of Fig. 2(b).
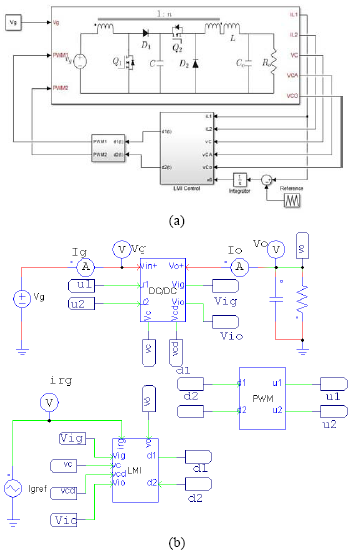
Figure 2 Non-inverting buck-boost converter and its respective current control block diagrams. (a) Buck-boost converter Simulink model. (b) Circuit diagram corresponding to the PSIM simulation.
The LMI control procedure consists of finding the feedback gain F such that satisfy the set of requirements of the feasible problem of the equation (14). The feasible problem is subject to constraints on stability (10), on pole placement (11)-(12) and on control effort (13). We chose as control parameters those of Table 1.
Once the parameters values of the converter and controller have been defined, the next step is to obtain the feedback gain vector. Thus, solving the feasible algorithm (14) for the system (5), by means of the LMI Toolbox of Matlab [38], the state-feedback controller obtained for the converter is:
After the LMI input current control has been designed, specific simulations must be required to verify the correct performance of the system.
These simulations are input current reference changes under the buck and boost modes, which will be shown in the following subsections.
3.1 Buck Mode
The first simulation tested the buck-boost converter LMI input current control in buck mode applying the current reference values changes from Igeref = 4 A to Igeref = 8 A, with a 2-kHz triangular waveform and a 50 % duty cycle shown in Fig. 3. Different current ripple values show the different operation points of the converter when the output voltage changes from 35 V to 45 V, with a constant input voltage and resistance load of 70 V and 4 Ω, respectively. PSIM and Matlab simulations matched correctly.
3.2 Boost Mode
Similarly to the above subsection, the simulation results from Fig. 4 shows good agreement between Matlab and PSIM simulation results. This test has been done using a constant input voltage and resistance load of 30 V and 4 Ω, respectively. The change of current reference values has been done by means of 2-kHz triangular waveform. The reference current Igeref varies from 8 A to 16 A, while the output voltage changes from 32 V to 41 V.
4. Conclusions
An input current control based on LMI for an 800-W coupled-inductor buck-boost dc-dc switching converter has been designed and simulated in this work. This control technique would make the converter also suitable for large voltage conversion ratio applications like unregulated sources such as battery charging and discharging, power factor correction, fuel cell regulation, maximum power tracking of solar panels, among others. Additionally, the zero crossing saturation problem in the low-level analog current controllers could be addressed by means of this state-feedback controller. The theoretical analyses have been simulated and validated by means of Matlab and PSIM. Actually, we are studying the transition between buck and boost modes and we pretend to extend this analysis designing an input/output LMI current controller in future studies. The aim of this work will be improve the modular properties of the buck-boost converter used in previous research works.