INTRODUCTION
Tomato crops are associated with a wide range of pathogens: bacteria, fungi, viruses and nematodes present throughout the plant. Currently, at least nine fungal diseases, five bacterial diseases, and six viral diseases have been defined for tomato crops in addition to nematode attacks and eight different types of insect pests (Silva-Neto et al., 2013). Chemical control through the use of pesticides (insecticides, fungicides, nematicides, acaricides) is the main management method in tomato production systems in Brazil and in the world (Vale et al., 2013).
The annual consumption of agrochemicals in Brazil surpasses 300,000 t of formulated products, representing over 130,000 t of active ingredients. The consumption of agrochemicals has increased 700% in the last 40 years, while the agricultural area grew by only 78%, showing an increase in the consumption of agrochemicals per production area; 899,000,000 L of pesticides were sprayed in crops in only three Brazilian states (Mato Grosso, Paraná and Rio Grande Sul) (Fletcher and Barnett, 2003; Spadotto et al., 2004; Pignati et al., 2017). However, we emphasize that the inappropriate use of products can be extremely negative for the biological systems found in cultivated areas, which include biotic (pollinators, natural enemies and vegetation) and abiotic components (water and soil) (Freitas et al., 2009).
The main cause of the reduced number of pollinators in crops is the abusive or inappropriate application of agrochemicals. The indiscriminate and irrational use of agrochemicals in agroecosystems can unbalance the population of bees that visit these sites (Rocha, 2012). Insecticides cause acute toxicity, killing bees, and may also cause behavioral changes in individuals, which over time, causes serious damage to colony maintenance (social) or to populations of para-social or solitary bees (Rocha, 2012).
Among pesticides, neonicotinoids present a large problem for the pollination process. This class of insecticides is absorbed by plants, contaminating the pollen and nectar grains that are consumed by pollinators. In addition, even plants that were not treated can absorb chemical residues through soil previously treated with neonicotinoids (Hopwood et al., 2012) because of the long residual action. Gill and Raine (2014) reported that exposure to neonicotinoid and pyrethroid pesticides cause acute and chronic damage to the foraging activity of Bombus terrestris L. (Hymenoptera, Apidae) bees in the United Kingdom, leading to changes in behavior that are detrimental to colony survival and pollination. There is a high consumption of pesticides in Brazil, and their effects on pollinators are still unknown. Tomato crops, although autogamic, are pollinated by several bees. These bees carry out buzz pollination, clinging to the anther cone and vibrating it (leaving a mark on the pollinated flower). The vibration expels pollen from the anthers and increases the deposition of pollen grains on the stigma of the flower (pollen load). Thus, in addition to feeding on pollen from flowers, bees help increase production and the quality of fruits (Silva-Neto et al., 2013; Silva-Neto et al., 2016).
There is general concern about how insecticidal pesticides may affect pollinating bees and agricultural production (Hopwood et al., 2012). However, other classes of agrochemicals have not been studied because they do not affect pollinators directly. Nevertheless, fungicides can be lethal and/or sublethal to bees (Riedl et al., 2006). Johnson et al. (2013) emphasized that fungicides alone are not considered highly toxic to Apis mellifera (Hymenoptera, Apidae), but also stressed that when this group of pesticides is combined with other groups, the toxicity of the components increases, causing more damage to bees. Degrandi-Hoffman et al. (2015) showed that bees that fed on pollen contaminated with fungicide (Pristine group -pyraclostrobin + boscalid) presented sub-lethal effects of malnutrition, increased amount of viruses and higher pathogen susceptibility. However, Artz and Pitts-Singer (2015) observed that Rovral 4F (iprodione) and Pristine, fungicides commonly used in agricultural crops in California (USA), disturbed the orientation of two solitary bee species (Osmia lignaria and Megachile rotundata, Hymenoptera, Megachilidae), hindering return trips to the hive. In view of the above, the objective of this study was to estimate the effects of fungicides on the mortality of Melipona quadrifasciata bees, on the in-field pollination of flowers and on the formation of tomato fruits.
MATERIALS AND METHODS
Study areas
The experiment was conducted under field conditions in the garden of the School of Agronomy of the Federal University of Goiás (16°35'48" S and 49°16'53" W, altitude of 709 m), municipality of Goiânia-GO, from November, 2014 to January, 2015. The climate is classified as Aw according to the Kõppen classification and is characterized by a dry winter and rainy summer (Peel et al., 2007). Annual rainfall is approximately 1,487.2 mm. The soil has a medium texture and is classified as Dystrophic Oxisol (Embrapa, 2006).
Experiment design and implementation
The experiment was conducted in a randomized blocks design, with 10 treatments (Tab. 1) and four replicates (flowerbed A, B, C and D). The "Italian tomato" tomato hybrid (Feltrin®) was used. Each plot consisted of eight plants with 30 cm spacing, two planting lines per plot, and 1m wide flowerbeds. The flowerbeds were covered with black mulch. The seedlings were transplanted 30 d after sowing on November 13, 2014. A bamboo and wire tutoring system was installed in the beds to support the tomato plants, and a drip irrigation system was used. Liming and fertilization procedures were carried out before planting the seedlings in addition to the cover fertilizer used after planting, following the soil analysis results. The first leaf spray was performed 8 days after transplanting (dat) (November 21, 2014), and the subsequent sprays occurred at weekly or fortnightly intervals (Tab. 1).
Pollen load of the flowers
The flowers were collected and fixed in FAA 80% (90% formaldehyde, 5% alcohol 80 °GL and 5% acetic acid) in December, 2014 at 70 dat to compare the amount of pollen grains in the stigma of the flowers of the plants exposed to the different treatments (n = 20 per treatment). Then, the stigmas were separated and placed in 9N NaOH for 1 h, and were stained with acetic acid and observed under an optical microscope.
The number of pollen grains in each stigma was counted in three fields of vision under an optical microscope (40x magnification). The fields of vision were the two opposing ends of the stigma and its central part (Dafni et al., 2005).
The presence of marks left by the jaws of bees on the anthers of the flowers during their visits was also observed. This mark is an important indication that bees that perform buzz pollination in poricidal anthers have visited. For this, the anther was observed under a stereoscopic microscope, where the mark was observed for its presence and the distance from the mark until the opening of the anthers' cone was measured using a millimeter paper as reference (Morandin et al., 2001a; 2001b; Silva-Neto et al., 2013).
Fruit characterization
Three tomatoes were collected per plant, amounting to 15 units per treatment (January, 2015, 90 dat), to evaluate the effect of pollination on fruit formation and production. The mass of the total fresh weight was measured on a precision scale (0.001 g) using gravimetry. The seeds were separated in Petri dishes and counted with a manual counter. The height and diameter of the fruits were evaluated using a digital caliper.
Bee mortality experiment
The Mandaean bees [(Melipona quadrifasciata (Lepeletier)] came from the hives of the School of Agronomy of the Federal University of Goiás, Goiânia-GO (16°35'16.39" S and 49°17'32.56" W). The bees were collected using a pooter and each colony was isolated in separate containers. Therefore, each colony was a replicate of the experiment. Samples of 10 to 15 bees per container with four replicates were used for each pesticide, plus the control. Thus, there were four samples with about 40 to 50 bees per treatment, amounting to over 200 bees. The collected bees rested for an hour, and the feeding consisted only of sugar syrup before they started feeding on food with added pesticide. The experiment procedure was performed in the laboratory under controlled conditions, with a temperature of 24°C.
Each container consisted of a clear, 1,000-mL plastic pot with part of its lid cut and replaced with a screen for ventilation (Fig 1). The containers were kept in the laboratory in a dark environment with a controlled temperature (20 to 25°C). Four agrochemical fungicides in addition to the control were evaluated, namely: Nativo® (trifloxystrobin+tebuconazole); Mancozeb® (manganese and zinc); Kocide® (copper hydroxide), and Serenade® (Bacillus subtilis). For each treatment, 50 mg of product were used in solution with sugar syrup (50%) at 3 mL in a 15 mL tube cap for the first feeding, replacing only the syrup every 24 h (adapted from Johnson et al., 2013).
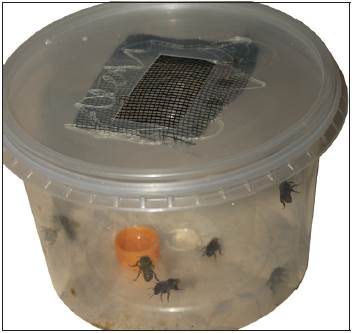
Figure 1 Plastic container with fungicide associated with the diet of bees from the species Melipona quadrifasciata.
The mortality and behavior (alimentation, motility and orientation) of the bees were checked every 3 h, except between 10 pm and 7 am. The number of dead bees was recorded for 54 h, and the values were used to calculate the mortality rate (percentage of dead bees divided by the total number of bees).
Statistical analysis
The significance of the effect of the treatments was determined with an F-Test. A Tukey multiple comparison test with a statistical significance of 95% was used. A principal component analysis (PCA - correlation matrix) was used to characterize the different treatments simultaneously. To perform the analyzes, we used the software Past 2.17.
A polynomial regression analysis was carried out to assess the mean mortality rate over time, observing the best fit in the curve and considering a statistical significance of 95% for each treatment. The mortality rates of the different treatments, at 24 and 48 h of evaluation, were compared using an analysis of variance with Tukey's multiple comparison test (95%).
RESULTS
The mortality rate for Melipona quadrifasciata, during 24 h of evaluation, was higher for copper hydroxide (75%) and trifloxystrobin+tebuconazole (50%). However, the mortality rate for manganese (2%) and zinc, B. subtilis (19%) and the control treatment (2%) did not differ from each other (F(2;22) = 66,461; F=0,0000). The higher mortality rates for the 48-hour evaluation period were observed for copper hydroxide (88%) and trifloxystrobin+tebuconazole (55%), while the mortality rates for the other treatments were the same as that recorded for the 24-h period (F = 6.72; GL=8; F=0.0001) (Fig. 2).
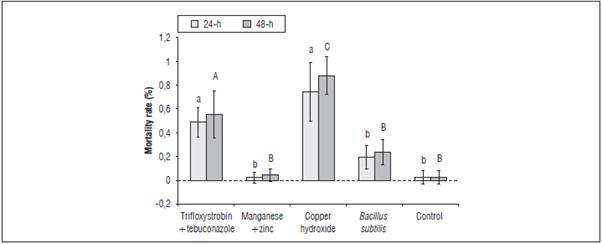
Figure 2 Mortality rate of Melipona quadrifasciata in different treatments with fungicides and evaluation periods. Lowercase letters differentiate statistical significance for the treatment evaluated for 24 h, and capital letters for 48 h (P≤0.05).
The treatments with fungicides, copper hydroxide and trifloxystrobin+tebuconazole exerted an acute effect, with increasing mortality rate up to 24 h, killing most of the bees. From 30 h on, the mortality rate stabilized until reaching 54 h of evaluation. The fungicides that caused the highest mortality, before killing all the bees, caused reactions, such as repetitive behaviors, imbalance and diarrhea (Fig. 3).
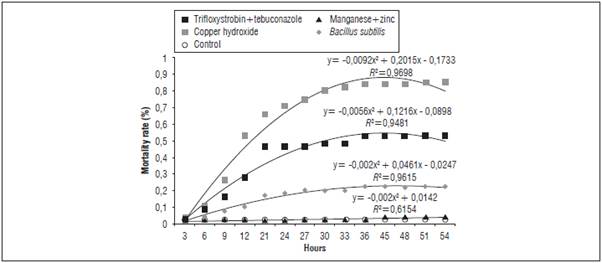
Figure 3 Average mortality rate of Melipona quadrifasciata in different fungicide treatments and 54 h of evaluation.
The fungicide (active principle), the dosage and the frequency of application all affected bee pollination and, consequently, fruit production {F(10.315)=2.823; F=0.002}. Lower pollen loads in the flower stigma were recorded for treatments 7 (trifloxystrobin+bacillus), 9 (propineb+trifloxystrobin+tebuconazole) and 10 (trifloxystrobin+tebuconazole+ B. subtilis + copper hydroxide), consisting of 100 grains of pollen, less than the combination of copper and Mancozeb. All of the treatments had the chemical compound trifloxystrobin although the the combination of compounds varied (Tab. 2).
Table 2 Treatments carried out on the tomato plants (Solanum lycopersicum) and results obtained for the pollen load (Pollen), pollination marks (Marks), mark distance, number of seeds, mass, height and width (Height x Width). Means with different letters indicate significant difference according to the Tukey test (P≤0.05) (F(10,315)=2.823; P=0.002).
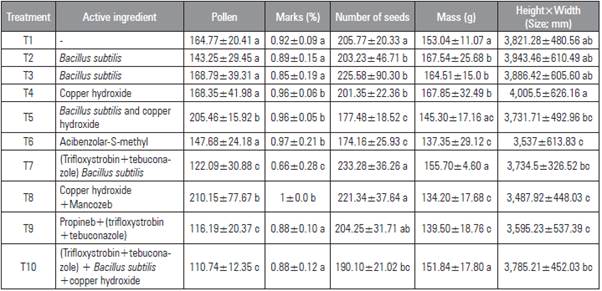
The same treatments that provided the lowest pollen load also presented the lowest percentage of pollination marks in the flowers. The treatments containing trifloxystrobin reduced the number of pollination marks by 44%. The number of seeds varied among the treatments, different from the pollen load, but still a reflection of pollination. The treatments with the lower amount of seeds were treatment 5 (B. subtilis+copper hydroxide), 6 (acibenzolar), and 10 (trifloxystrobin+tebuconazole+ B. subtilis+copper hydroxide). Despite exhibiting the lowest pollen load, treatment 9 (trifloxystrobin+ B. subtilis) provided one of the highest number of seeds, with gains over 24%, as compared to that obtained in the worst treatment (T6 or acibenzolar-S-methyl).
The treatments that provided greater production in mass and larger fruits were treatment 1 (control), 2 (B. subtilis 12), 3 (B. subtilis 6), 4 (copper hydroxide), 7 (trifloxystrobin+B. subtilis) and 10 (trifloxystrobin+-tebuconazole+ B. subtilis+copper hydroxide). The better treatments, in regards to all of the variables assessed, were the control treatment, the biological interventions (B. subtilis 6 and 12) and the treatments using copper hydroxide. Treatment 10, with tri-floxystrobin, tebuconazole, B. subtilis and copper, also presented gains compared to the treatments that used the same chemical without Bacillus. Treatment 6 (acibenzolar), 8 (copper hydroxide+manganese and zinc) and 9 (propineb+trifloxystrobin+tebuconazole) provided the worst results for pollen load, seed and fruit mass, except when combined with B. subtilis.
The agrochemical application frequency was important for bee mortality and tomato production. A higher number of applications of a given treatment can cause a lower occurrence of pollination marks and pollen grains on the stigma and lead to fruit with a lower number of seeds, mass, and size (Fig. 3 and 4).
DISCUSSION
The results on the mortality rate of Melipona quadrifasciata is congruent with the lethal dose effects (LD50) of some fungicides described for Apis mellifera as described in the package leaflet of the studied pesticides. The fungicide Kocide® (copper hydroxide-based) had a LD50 48 h after the intake of only 8.15 μg kg-1. However, the same lethal dose for contact was 100 μg kg-1. The fungicide Nativo® (trifloxystrobin+ tebuconazole-based) exhibited a LD50 for ingestion of 273.86 /μg/bee and 298.4 μg/bee for contact. Mancozeb presented a LD50 of 100 μg/bee, without reference to contact or ingestion, as described in the leaflet of each pesticide. The units may vary since they are established by the manufacturer.
Copper is a widely used component in agriculture in the form of sulfates and hydroxides and is allowed in the production of organic foods because it is considered non-toxic and not harmful to the environment. However, this statement has been challenged in regards to bees. In this study, the toxicity and mortality of the bees as the result of the ingestion of copper (copper hydroxide) were considered high, even when compared with trifloxystrobin and tebuconazole based products. Barbosa et al. (2015) studied the effects of copper-based fungicides on bees in bioassays with the stingless bee Friesella schrottkyi (Friese) and reported high mortality rates for worker bees, but low rates when compared to other pesticides, such as spinosad (insecticide).
The fungicides evaluated in this study with the highest M. quadrifasciata mortality rates are considered as lethal as some insecticides that are more harmful to bees. Jacob et al. (2013) tested fipronil in Scapto-trigona postica and Costa et al. (2015) tested imidacloprid in M. scutellaris; both found mortality results similar to that reported in this study for fungicides. This shows that fungicides can be as harmful to bees as insecticides even though that is not their purpose. Here, we point out that, even though they are different species or different methodologies, these results are important, showing the impacts of agrochemicals on bees.
The results obtained in this study only show the acute effects on bees, occurring approximately 50 h after contact of the bees with the fungicide. Chronic effects may also occur, but should be investigated more thoroughly. The fungicides tested on M. quadrifasciata were only evaluated by ingestion with sugar syrup since sole contact of the product with the exoskeleton of the insect may cause other effects, such as symptoms of malnutrition, susceptibility to pathogens, and orientation disturbance in flight and foraging. Agrochemicals, even when used in low frequency or concentration levels, may cause lethal or sublethal effects, with behavioral changes that affect pollinator choices (Thompson, 2003).
Other products, such as Serenede® (based on B. subtilis) and Bion® (acibenzolar-S-methyl), did not show predetermined LD50 for any bee species (including Apis mellifera).Ngugi et al. (2005) used Apis mellifera as vectors of Bacillus subtilis and pollinators of blueberries in a greenhouse. In this case, B. subtilis was used to fight a fungus common in the culture, Monilinia vaccinii-corymbosi (Reade) Honey.
Copper hydroxide (Kocide®) is a pesticide with a high mortality rate among M. quadrifasciata bees, but did not considerably reduce the number of bite marks in the anthers of the tomato flowers. This lack of bee visitation marks on the tomato flowers may have been due to the residue of the pesticide remaining on the flowers as a powder and not as a solution (as the applied in this study), which may reduce the product's potential harm to insects. Kocide® did not affect bee visitation in this research, but this does not mean that residue in flowers cannot harm bees after pollen collection, with lethal or sub-lethal effects on bee development.
Unlike the toxicity presented by copper hydroxide, Mancozeb® (Carbamate with manganese and zinc salts) was not toxic for M. quadrifasciata, providing results similar to the control treatment. If Mancozeb® is considered efficient in controlling fungal infections in tomato, it is an agrochemical option with less impact on pollinating agents.
For pollen load, the alternating trifloxystrobin+tebuconazole and B. subtilis+copper hydroxide applications reduced the visitation effect and increased pollination, as compared to the application of only trifloxystrobin+rebuconazole. The concern about the effects of pesticides on pollinating bees is almost always associated with insecticides because of their direct effect on insects (Carvalho et al., 2009). Still, fungicides comprise a large class of chemicals widely used in agriculture, especially in tomato crops (Vale et al., 2013).
Sanchez-Bayo and Goka (2014) studied pollen grains contaminated with agrochemicals and found that fungicides are more frequent in contaminated pollen. This finding reinforces the need for specific studies on the effect of fungicides on pollinating bees in agricultural crops. Thus, this biological control was efficient in fighting fungal diseases and did not affect the bees that pollinated the crop. Ngugi et al. (2005) reported that B. subtilis did not affect the deposition of pollen grains on stigma, nor the growth of the pollen tube, meaning pollination occurred as expected under natural conditions. Bees are insects that naturally interact with fungi and microorganisms. When these microorganisms are not directly pathogenic to bees, the groups may interact in equilibrium (Mcfrederick et al., 2014). Therefore, the interaction between B. subtilis and bees may be harnessed for agricultural purposes (Park et al., 2013).
Pettis et al. (2013) detected about 35 agrochemicals in pollen grains from almond, apple, blueberry, cranberry, pumpkin, melon and cucumber crops, most of which were insecticides followed by fungicides. The authors concluded that a greater amount of pesticide on pollen leads to an increased chance of pollinators being infected by the fungus Nosema spp., which is considered very harmful to bees (Apis mellifera and natives). Thus, they highlighted the relevance of verifying sub-lethal effects of fungicides and other products used in agriculture on bees.
Some fungicides can have a major impact on crop pollination by reducing the number of visits to crop flowers, by repelling bees or affecting plant physiology and, consequently, reducing pollen viability and availability of nutritious food (Solomon and Hooker, 1989; Riedl et al., 2006). Captan® is a fungicide widely used in apple trees and may cause reduced visitation by reducing the attractiveness of flowers to bees through the presence of harmful chemical compounds. Thus, Captan® reduces foraging, causes morphogenic defects in adults exposed in the larval stage (on legs, wings and body) and increases larval mortality (Mussen et al., 2004; Freitas and Pinheiro, 2010).
In organic tomato crops in Piauí, 76% more bees were found than in conventional crops that use pesticides. This shows that the products may be affecting the pollinator community and visitation, with lethal or sublethal effects (Santos and Nascimento, 2011). The use of pesticides during flowering in the cultivation of mangoes (Mangifera indica) in the sub-middle San Francisco Valley reduced the frequency of bee visits by 50% and diptera by 20% (Siqueira, 2008).
In this study, the frequency of the application of fungicides was relevant in the tomato crop in terms of bees. The application frequency was related to seed production and fruit characteristics in the principal components analysis. The agrotoxic agent applied at a higher frequency, even when biological (Bacillus subtilis), reduced bee visitation more intensely than products considered more toxic (acibenzolar-S-metil) applied with a lower frequency. This pattern reinforces the relationship between the application frequency of agrochemicals and manufacturer's recommendation, making it vital to check the period established by the manufacturer for the product to be re-applied to a crop. The application frequency of agrochemicals, time of day and forms of application are relevant for the effects that products will have on targets (insect pests and fungi) and on non-target insects, such as bees (Franceschinelli et al., 2017). These non-target effects are relevant research topics for future studies. Thus, a greater number of applications of pesticides on tomato flowers leads to a lower number of visits from bees to flowers. Consequently, there will be a smaller amount of pollen grains deposited on the stigmas, affecting fruit quality and yield (Nunes-Silva et al., 2013; Silva-Neto et al., 2013).
The effects of different pesticides differ among products from different classes (e.g., insecticides, fungicides, herbicides and others) in terms of their mechanisms of action on bees. Insecticides affect bees as neurotoxic agents, affecting the transmission of the nerve impulse, interferes with growth, damaging the transformation of the stages of the insects, and affects metabolic processes, such as cellular respiration and death. For the fungicides, the direct and indirect effects verified for the bees and the mechanisms of action are not known (Tomé et al., 2015; Lima et al., 2016; Rodrigues et al., 2016). Thus, more research on pesticides (insecticides and other classes) and agricultural crop pollinators are needed.
In conclusion, Kocide (copper hydroxide) and Nativo (trifloxystrobin and tebuconazole) provided the highest mortality rates for Melipona quadrifasciata. The treatments with Mancozeb and Serenade and the control treatment did not differ from each other.
Trifloxystrobin and tebuconazole were the most repellent active ingredients for bee pollination in the tomato crop. Fruits from the plants treated with Bacillus subtilis and copper hydroxide were larger and had higher mass.