INTRODUCTION
The tomato (Solatium lycopersicum L.) is one of the most consumed vegetables globally, mainly because of its high nutritional value and antioxidant poten tial (Du et al., 2017). Overall tomato production increased from 27.6 million tons in 1960 to 177 mil lion tons in 2016 (FAOSTAT, 2016). In Brazil, table tomato cultivation stands out because of its socio economic importance, as it generates jobs, improv ing the income of rural workers and growers (Silva et al., 2013). Many factors can lead to a reduction in crop productivity, affecting the quality of the final product, which include problems caused by pests and diseases (Zaidi et al., 2018).
Disease management has become a challenge in agri culture as it requires pesticides, often with high toxicity (Carrascosa et al., 2015). Chemical pesticides, particularly soil fumigants, have been severely re stricted in recent decades because of the environmen tal consequences (Van Bruggen and Finckh, 2016), the residual effects on soil and the long period nec essary for degradation. In addition, the use of pesti cides in the environment impacts human health, and resistance is acquired by pathogens for the chemical groups. The use of pesticides should be minimized and rationalized to promote more sustainable agri culture (Lamichhane et al., 2015).
Phytosanitary management needs to be improved to minimize or mitigate its impact on the environment (Dayan et al., 2009). Plants have mechanisms to re spond to different types of stress, whether abiotic (Shah et al., 2014) or biotic (Shah and Zeier, 2013). These natural defense mechanisms remain inactive or latent until activated after exposure and/or con tact with inducing agents (Mandal, 2010).
The defense mechanisms of a plant are genetically controlled, depending on the expression after contact with the host. So, plants can activate the defense mechanism in several ways, including through oxidative activities (Teixeira et al., 2017; Xue and Yi, 2017), enzymes involved in the phenylpropanoid route (Sangeetha and Sarada, 2015), and enzymes involved in lipid peroxidation, among other forms. The use of resistance-inducing products for different crops is increasing, for example on vine (Xue and Yi, 2017), wheat (Moya-Elizondo and Jacobsen, 2016), pepper (Siddiqui and Meon, 2009), and peach (Jiao et al., 2018), mainly.
When plants recognize the resistance inducers ap plied, the intracellular signal transduction pathways are activated (Shah et al., 2014). Perception occurs when the molecules of the inducing agent bind to receptor molecules that are probably located in the plasma membrane of the plant cell; this reaction trig gers the activation of various defense mechanisms, culminating in protection against pathogens (Gra ham and Myers, 2011). These inductions alter the physiology of the plant and can interfere directly with productivity.
Application of resistance inductors that have Silicon (Si) is a potentially sustainable option to improve biotic and abiotic stresses in several plants (Zhu and Gong, 2014; Liang et al., 2015; Cooke and Leishman, 2016; Etesami, 2018).
Despite the importance of the use of these products, there are few studies on resistance inductors based on silicon oxide and aluminum in the tomato to ta ble culture. One source of these nutrients available to growers is through soil-mineral compost. Thus, this study evaluated the effect of foliar applications of a soil-mineral compound on the physiological and growth attributes of tomato plants.
MATERIAL AND METHODS
This experiment was conducted in the 2016 grow ing season in the commercial area of Lagoa Formosa, Minas Gerais, Brazil (18°30'01.6" S and 46°30'48.2" W) in the first crop of the year. The cultivar "Domi nador" was used, which has an indeterminate growth habit, high vigor, average cycle duration of 120 d and fruits with an aptitude for salads. The seedlings were produced in trays and transplanted to the field when they had two leaves, 28 d after sowing.
Cultural management including fungicides, insec ticides and herbicides was carried out in all treat ments. Each plot was composed of five rows, with a 6 m length, 2 m row spacing, and 0.6 m between plants. The useful area of each plot consisted of the three central lines, discarding 0.5 m at each border. The plants were irrigated with a central pivot and received fertirrigation according to the nutritional needs of the crop.
The experiment design used a randomized block with four treatments and five replications. The treat ments consisted of four management types of the soil-mineral compound during the crop cycle: man agement 1 was the control; management 2 consisted of foliar applications at the dose of 0.75 kg ha-1 per application at 15, 25, 40 and 60 days after transplant ing (DAT); management 3 consisted of foliar appli cations of 0.5 kg ha-1 at 15 DAT, 0.750 kg ha-1 at 25 DAT, 1.0 kg ha-1 at 40 DAT, and again at 60 DAT; and management 4 consisted of foliar applications of 1.0 kg ha-1 per application at 15, 25, and 40 DAT.
The soil-mineral compound was a fine, balanced powder, prepared by milling, micronization and stan dardization of special clays that are commercially dis tributed in Brazil. The soil-mineral compound was a fine powder composed of Al2O3 (20.6%), SiO2 (17.4%), S (9.8%), CaO (1.3%), TiO2 (0.34%), MgO (0.18%), Fe2O3 (0.16%), and P2O5 (0.10). The doses were as in dicated in the commercial product (Rocksil®).
The foliar applications were done with costal spray ing with a CO2 injection. The bar contained a fan-type nozzle, with a constant pressure of 2.0 bar.
Biochemical evaluations
Leaf samples for the nitrate reductase determinations were performed at 8, 26, 40, 47 and 73 DAT, and, for the other analyses, they were taken only at 73 DAT. Completely expanded leaves were collected from the middle third of the plants.
The activity of the enzymes nitrate reductase, urease, lipid peroxidation, peroxidase enzymes, superoxide dismutase, phenylalanine ammonia-lyase, hydrogen peroxide, and total proteins were determined.
The NR analysis was performed according to the method proposed by Mulder et al. (1959). The urease was evaluated throughout extraction, and the analy sis of the plant material was done according to the methodology adapted from Hogan et al. (1983).
Samples of 200 mg of fresh biomass of leaves were macerated with 4.0 mL of 0.1 mol L-1 potassium phos phate buffer pH 6.8. Then, the samples were trans ferred to Eppendorf flasks and centrifuged at 10,000 rpm for 30 min at 4°C (Kar and Mishra, 1976). Then, the samples were stored at -20°C until determina tion of the total protein content of the leaf (Brad ford, 1976), superoxide dismutase activity (SOD) (Beuchamp and Fridovich, 1971), peroxidase activity (POD) (Teisseire and Guy, 2000), and phenylalanine ammonia-lyase activity (PAL) (Umesha, 2006). With the fresh leaf biomass the hydrogen peroxide content (H2O2) (Alexieva et al., 2001) and lipid peroxidation (LP) (Heath and Packer, 1968) were also evaluated.
Biomass attributes
One plant per replicate was harvested at 47 and 83 DAT for determination of the root, stem and leaf biomass. The samples were dried in a forced-air oven at 65°C for 48 h before the determination of the dry biomass. Productivity harvests were also performed at 73, 81, 92, 102, 110, 119, 124, 130 DAT.
Statistical analysis
The data were evaluated for normality and homoge neity using Levene and Shapiro-Wilk tests, both at the 5% significance level. A variance analysis was performed, and, when significant, the Tukey test was applied at the 5% level of significance. For some analyses, regression analysis was also performed. The analyses were performed using statistical software Genes (Cruz, 2013).
RESULTS AND DISCUSSION
For the nitrate reductase variable, the variance analy sis presented significance only for the management; there was no effect from the harvesting times or in teraction. The other attributes of nitrogen metabo lism and the activity of antioxidant enzymes had no effect from the management. There was a difference in the productivity attributes.
The nitrate reductase enzyme did not present a sig nificant difference during the evaluation periods al though some management showed a tendency for increasing (Fig. 1). The nitrate reductase enzyme acts on nitrogen assimilation in plants and reduces nitrate to nitrite through NADPH (nicotinamide adenine dinucleotide phosphate hydrogen) energy. Thus, the increase in this enzyme activity contributes to the increase of the assimilation of nitrogen (N) and the growth and development of the plant (Taiz et al., 2016).
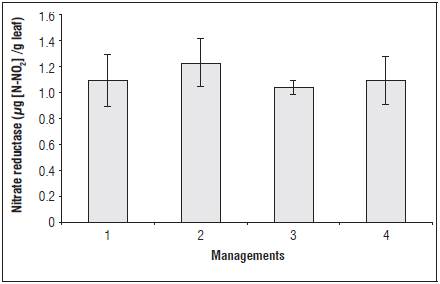
Figure 1 Activity of the enzyme nitrate reductase when submitted to different managements for foliar ap plications of the soil-mineral compound. Nitrate reductase (µg N-NO2/g leaf). The vertical bars indi cate ± standard error.
Resistance induction represents an extra energy ex penditure for plant defense and reduces nitrate reductase metabolism and consequently plant growth.
Thus, we verified that the foliar applications of the soil-mineral compound may not have activated re sistance mechanisms related to the nitrogen metabo lism enzymes.
According to Lawlor (2002), the role of nitrogen in the production of dry phytomass and, consequently, remobilization to reserve organs is directly related to photosynthesis. Photon energy is converted into chemical energy and stored in ATP (Adenosine triphosphate) and secondary metabolites, primarily NADH, which is used in the synthesis of carbon and nitrogen assimilates, particularly amino acids (Lawlor, 2002).
It was found that the activity of nitrate reductase re duces during harvests, more accentuated after flower ing. We hypothesize that the fruiting drain competes for the N present in the plant, which explains this reduction in enzyme activity.
The protein content and the activity of the urease en zyme did not differ between the management types; however, it was noted that treatments 2, 3 and 4 in creased the total protein content (Fig. 2A).
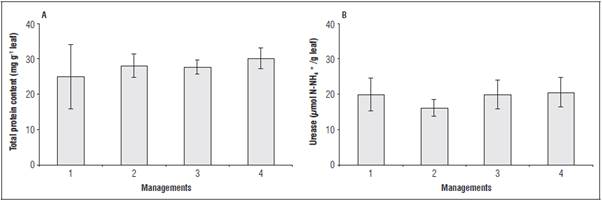
Figure 2 Protein content (A) and urease enzyme activity (B) in tomato leaves at 73 DAT when submitted to different management types for foliar applications of the soil-mineral compound. The vertical bars indicate ± standard error.
The quantification of the protein content in the leaf during the reproductive growth evidences the redis tribution of amino acids from leaves to reproductive growth. A higher protein content in the leaves may be an indicator of greater assimilation of atmospheric CO2 because the main enzyme of photosynthesis is composed of N, the ribulose-1.5-bisphosphate car boxylase/oxygenase (Rubisco) (Taiz et al., 2016).
Oxidative metabolism
The managements 2, 3 and 4 presented higher levels of hydrogen peroxide than management 1 (control), without significant differences (Fig. 3A). Manage ment 4 presented, on average, values of hydrogen peroxide production that were approximately 56% higher than treatment 1 (control), which demon strated that the resistance inducer activated the oxidative metabolism in some signal transduction pathways in the plant tissue.
The increase of this free radical indicates that the de fense signaling of the plants probably activated genes related to pathogenesis. Thus, the increase in the syn thesis of hydrogen peroxide does not always result in negative effects in plants, such as destruction of membranes, because this molecule can function as a signal agent in plants, which includes responses to pathogen elicitors, stomatal closure, acquired sys temic resistance, and programmed cell death (Chen and Gallie, 2005).
For the enzyme POD, there was a positive trend in managements 2, 3 and 4, without statistical signif icance (Fig. 3B). The increase in the activity of the POD enzyme as well as the increase in H2O2 content was a protective measure to degrade the reactive oxy gen species (ROS) in the plants (Barbosa et al., 2014). PODs are related to the synthesis of lignin and suberin, which increase the hardness of tissues and the production of quinones and active oxygen, which have antibiotic activities (Stout et al., 1994).
The activity of the SOD enzyme was reduced in managements 3, 4 and 2, in relation to treatment 1 (control) (Fig. 3C). This enzyme is responsible for the degradation of superoxide ERO in hydrogen perox ide, so it is later degraded by CAT and POD enzymes (Mittler, 2002). An increased SOD and POD activity is directly related to plant tolerance to environmental stresses, such as saline stress (Koca et al., 2007). How ever, when the activity of the enzyme is saturated be fore reaching the maximum concentration of reactive oxygen species, lipid peroxidation may occur.
The activity of the PAL had a different behavior (Fig. 3D). This enzyme is responsible for catalyzing the conversion of phenylalanine to trans cinnamic acid, the first step for the biosynthesis of phenylpropanoids. This compound is the basis for the synthe sis pathway of secondary metabolites that exhibit antioxidant activity, such as flavonoids and tannins (Dias et al., 2015). A different behavior can be ex plained by a different route of action for defending plants against stress. Alternative products, such as chitosan and plant extracts, may increase the activity of phenylalanine-ammonia-lyase (PAL) (Lorencetti et al., 2015).
The levels of lipid peroxidation were similar among all treatments (Fig. 3E). Lipid peroxidation is used as a basis to measure the damage caused by the action of reactive oxygen species on the unsaturated lipids of cell membranes. This leads to membrane destruc tion, failure of cellular mechanisms and, in extreme cases, cell death (Lima and Abdalla, 2001). Therefore, because there was no difference between the treat ments and the control, there was no cellular dam age from the foliar application of the soil-mineral compound.
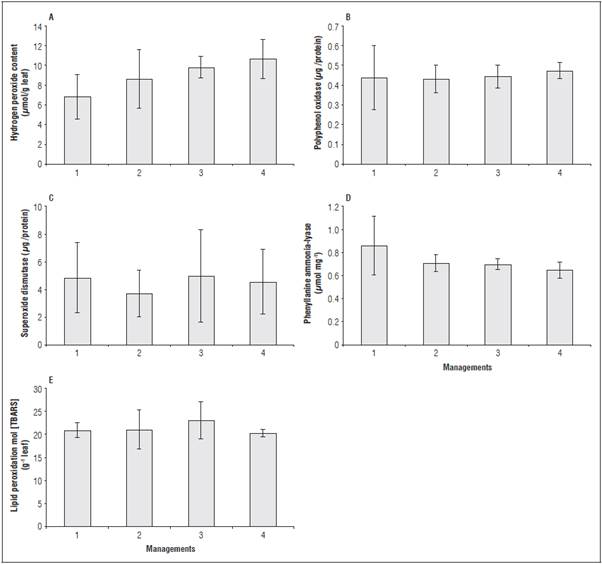
Figure 3 Hydrogen peroxide content (A), polyphenol oxidase activity (B), superoxide dismutase μ -1 (C), phenylalanine ammonia-lyase (D), and lipid peroxidation (E) of tomato leaves at 73 DAT, when submitted to different management types for foliar applications of the soil-mineral compound. The vertical bars indicate ± standard error.
Silicon increases the synthesis of phenolic compounds in plants and increases the activity of the following enzymes: polyphenol oxidase (PPO), peroxidases (POD) and phenylalanine ammonia-lyase (PAL) (Gomes et al., 2005). As a consequence, it tends to reduce the rate of pathogen growth and the incidence of pest insects. The application of Si increased the ac cumulation of phenolic compounds in walls of epi dermal cells of Triticum aestivum, and consequently, increased the resistance of plants infected by Blumeria graminis f. sp. tritici (Bélanger et al., 2003).
A PPO plays an important role in plants since it pro vides resistance to attack from pathogens and dis eases. It has also been reported that PPO may exert a direct relationship with photosynthesis since it assists in the maintenance of system homeostasis (Boeckx et al., 2015). This enzyme performs the oxi dation of diphenol in quinone, a beneficial compound for the photosystem. In addition, during this process, PPO removes excess O2 from the system, avoiding the possible formation of superoxide radicals (Boeckx et al., 2015).
Although the foliar applied soil-mineral compound had Si, no change in the activity of SOD and PAL en zymes was observed. The interaction of Si with these enzymes is probably more complex and depends on other factors, such as dose, culture, form of appli cation, and interaction with other nutrients in the application, among other factors, which needs more studies to be better understood.
Biomass attributes
About 95% of the dry mass accumulated by plants during their cycle is derived from the photosynthetic activity, and the rest comes from the soil (Benincasa, 2004). The root dry and stem dry biomass did not dif fer between the management types (Fig. 4AB). There was a trend for a higher dry leaf biomass in manage ment 4, without significant differences (Fig. 4C).
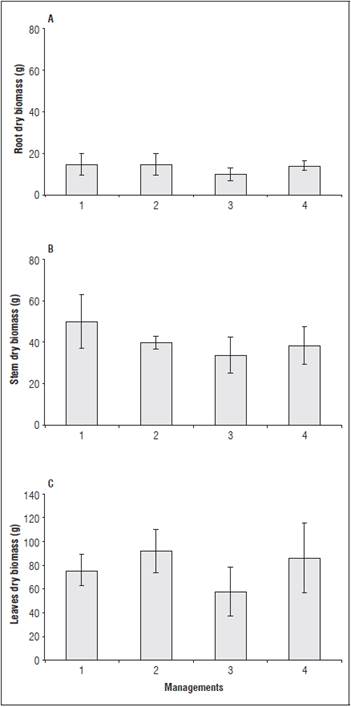
Figure 4 Root (A), stem (B), and leaf (C) biomass of tomato plants when submitted to different management types for foliar applications of the soil-mineral compound. The vertical bars indicate ± standard error.
Si, after being absorbed, is translocated and deposited just below the cuticle, forming a double layer of sili con-cuticle. This contributes to protection from abi otic stresses, such as: elemental toxicity, salinity, and frost, among others; as well as protection from biotic stresses, such as pests and diseases (Ranganathan et al., 2006). The accumulation of Si makes leaves more upright and rigid and increases the interception of light and, consequently, photosynthetic efficiency (Gonçalves, 2009).
Productivity
All treatments with foliar applications of the soil-mineral compost increased yield per plant and yield. The highest production per plant and productivity were obtained in management 3, with 3.11 kg/plant and 31,152 kg ha-1, respectively (Fig. 5, A and B). The use of the soil mineral compost increased tomato yield by 20% in management 3, a good option for in creasing crop productivity.
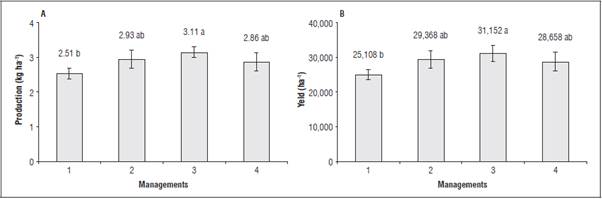
Figure 5 Production per plant (A) and yield (B) of tomato plants when submitted to different management types of foliar applications of the soil-mineral compost. Means with different letters indicate a significant statistical differences according to Tukey test (P≤0.05) (n=5) ± standard error.
Si, applied in the form of aluminum silicate on vines (Vitis vinifera), contributes to the control of mildew (Plasmopara viticola) (Gomes et al., 2011). The use of a soil-mineral compost in guava (Psidium guajava) con tributes to the control of anthracnose (Colletotrichum gloeosporioides) and reduced the diameter of colletotricum lesions (Colletotrichum gloesporioides) in fruits (Gomes et al., 2016). The aluminum oxide (Al2O3), silicon dioxide (SiO2) and sulfur (S) present in the soil-mineral compost inhibited in vitro tests on the mycelial growth of colletotrichum (Colletotrichum gloesporioides) (Gomes et al., 2016).
We hypothesize that foliar applications of aluminum oxides can trigger the production of reactive oxygen species in plants, which stimulates the production of antioxidant enzymes.
Si was identified as a resistance inducer in some monocotyledons, such as rice (Oriza sativa), corn (Zea mays L.) and wheat (Triticum aestivum L.), which actively absorb and accumulate large amounts of silicon (Liang et al., 2015). This was also observed in some dicotyledonous crops, such as cotton (Gossypium hirsutum), soybean (Glycine max (L.) Merr.), and tomato (Solanum lycopersicum L.), and also in some cucurbitaceous species (Liang et al., 2015).
Si can favor the upright position of leaves, indirectly favors photosynthesis, and makes the opening and closing of stomata more efficient (Pereira et al., 2003). The productivity of sugarcane increased with the fo liar applications of Si (Elawad et al., 1982). Foliar ap plications of potassium silicate (K2SiO3) increase the chlorophyll content and growth of strawberry plants (Fragaria X ananassa) (Wang and Galletta, 1998).
The effectiveness of the application of Si in the miti gation of stress depends on the species (Mitani and Ma, 2005) and the level of stress suffered by the plant (Hodson et al., 2005). In this experiment, the plants were not subjected to severe stress conditions, which may have contributed to the stress metabo lism not responding significantly to the soil-mineral compound treatments. Biochemical and molecular responses using Si occur when the plant is subject to stress conditions (Liang et al., 2015).
Although the treatments with foliar applications did not modify the activity of the evaluated en zymes, the increase in productivity showed that the management types with the compost soil mineral contributed in another way to an increased tomato productivity. We hypothesized that there may have been an increase in proteins, lignins, and efficiency of the photosynthetic activity, but more studies are required for a better understanding.
CONCLUSION
The foliar applications of the soil-mineral compost at doses of 0.5, 0.75, 1.0 and 1.0 kg ha-1 at 15, 25, 40 and 60 DAT, respectively, increased the yield of the table tomatoes.
No effect from the foliar applications of the soil-min eral compound was observed on the enzymes related to stress metabolism as a total protein, hydrogen peroxide, nitrate reductase enzyme activity, urease, superoxide dismutase, peroxidase, phenylalanine am monia lyase and lipid peroxidation under the condi tions of this study.