INTRODUCTION
India leads banana production with 18% of the global harvest, 139 million t year-1 (Santosh et al., 2017). However, in terms of exporting countries, Ecuador ranks number one with 6,685,000 t, while Colombia occupies fourth place with 1,806,000 t, after the Philippines (3,950,000 t), Guatemala (2,494,000 t) and Costa Rica (2,200,000 t) (FAO, 2020). In Colombia, the average productivity in 2018 was 2,002 boxes/ha, equivalent to 36 t ha-1 and higher than that 2017 (AUGURA, 2018). The climatic behavior that characterizes the Uraba region, dry in the first half of the year and wet in the second half (Toro et al., 2016), directly affects production, generating shortage in the first half and surpluses in the second half, which directly affects international prices (AUGURA, 2017; 2018).
The photosynthetic process in plantains and bananas is controlled by internal factors, such as the genetic material, state of leaf development, integrity of the leaf blade, and by external factors, such as seasonality, daily climate, photosynthetically active radiation (PAR), shading and watering regime (Turner et al., 2009; Ramirez et al., 2011; Robinson and Galán, 2010). In Musaceae, external factors from the competition for sunlight within the plant community (Cayón et al., 2004) and for water severely affect production (Cayón, 2004; Santos et al., 2018). On the other hand, intrinsic factors, such as low tolerance to water stress, in banana cultivars with a dominance of acuminata genes (Ravi et al. 2013) and physiological factors, such as premature stomatal closure, significantly influence the efficiency of plants (Surendar, 2015). However, proper management of density and arrangement between plants can control or decrease these effects and increase yield per unit area as a result of the efficient use of sunlight and water during the early phases of crop growth.
Rodríguez et al. (2007) asserted that arrangements between plants facilitate better distribution in the field and a greater interception of solar radiation. In bananas, the double row arrangement increased the number of plants per hectare and production through an increase in the Leaf Area Index (LAI), which is a determining factor in PAR interception (Patil et al., 2018). Consequently, LAI plays an important role in the design of cultivation systems since, by varying the spacing between plants, the light interception, vigor of plants and yield are affected. Therefore, decreasing the distance between plants means LAI increases the efficiency for intercepting incident solar radiation but increases the light extinction coefficient (k) of the crop (Rodríguez et al., 2007; Turner et al., 2007).
Thippesha et al. (2008) stated that, by reducing the distance between banana plants, the intensity of available light decreased for the middle and lower strata of the plants, along with the rate of photosynthesis, but did not influence the production of dry matter per plant or flowering time. However, the timing of the harvest and ripening of fruits are delayed as the plant density increases. According to Benson (2013), a planting density over 2,000 plants/ha alters the growth and development of plants because of the superposition of roots and overlapping of leaves, which generate excessive shade, causing a low bunch mass.
Vargas and Sandoval (2005) and Smith et al. (2010) suggested the need to validate the behavior of physiological variables in response to increases in plantation densities in banana crops. Because of the lack of information on the phenotypic plasticity of cv. Williams, which is the most cultivated plant under the agro-ecological conditions of Uraba, Antioquia, this study aimed to evaluate the effect of planting densities and arrangements between plants on the photosynthetic potential of the plant community and productivity under the climatic conditions of the region.
MATERIALS AND METHODS
This study was carried out during the second semester of 2015 and the first semester of 2016 on the La Venturosa farm of the Bananeras del Uraba Company in Turbo, Antioquia, located at 07°53’ N and 76°41’ W with the characteristics of a very humid tropical forest. The predominant bioclimatic parameters at the site during the study were a cumulative precipitation of 3,194 mm, higher in the second half of 2016 with 1,718 mm, an average monthly maximum and minimum temperature of 32.1°C and 22.3°C, respectively, a relative humidity of 87.01%, and a monthly solar brightness of 112.36 light hours. The soil presented the geomorphological position of terraces, flat relief developed from alluviums of different granulometry, medium depth with moderate internal drainage, silty clay loam texture and medium fertility, which made it representative of the Inceptisol order (IGAC, 2007).
The physical and chemical characteristics of the soil were: clay loam texture, with a pH of 5.85; organic matter (OM) of 1.73%; Ca and Mg of 10.3 and 3.11 cmol+ kg-1, a slightly wide ratio (3.31) that could infer a Mg deficiency; K of 0.71 cmol+ kg-1; Effective Cation Exchange Capacity (ECEC) of 14.2 cmol+ kg-1; P and S of 21.4 and 32.32 mg kg-1; Zn and Cu of 2.4 and 3.1 mg kg-1; B of 0.54 mg kg-1; Mn of 0.54 mg kg-1 and Fe of 32.3 mg kg-1. Based on these results and the nutritional requirements indicated by Torres (2016), the corresponding amendments were applied.
The cv. Williams plantlets obtained by in vitro culture were established in 40×40×40 cm holes, based on the design of the treatments presented in table 1. The phytosanitary management of pests, diseases (not including black sigatoka (Mycosphaerella fijiensis Morelet), weeds and cultural tasks such as: thinning, stripping, and phytosanitary defoliation were carried out cyclically in accordance with the technical recommendations of the technical assistance unit of the Bananeras de Uraba group, made in response to the suggestions of the marketing company UNIBAN.
Table 1 Description of the treatments, based on the combination of four plantation densities and three population arrangements in the banana crop (cv. Williams Musa AAA Simmonds).
Treatment | Population arrangement (m) | Population density (plants/ha) |
---|---|---|
1 | Control (2.7 x 2.7) | 1,700 |
2 | Triangle (2.3 x 2.3) | 2,000 |
3 | Triangle (2.15x2.15) | 2,500 |
4 | Triangle (1.95x1.95) | 3,000 |
5 | Triangle (1.8x1.8) | 3,500 |
6 | Rectangle (3.2 X 1.57) | 2,000 |
7 | Rectangle (2.8 x 1.30) | 2,500 |
8 | Rectangle (2.6 x 1.20) | 3,000 |
9 | Rectangle (2.4 x 1.15) | 3,500 |
10 | Double rows (dp* 1.0 m x dr** 3.3) | 2,000 |
11 | Double rows (dp 1.2 m x dr** 3.3) | 2,500 |
12 | Double rows (dp 1.0 x dr 3.0) | 3,000 |
13 | Double rows (dp 1.0 m x dr 2.8) | 3,500 |
*dp: distance between plants; **dr: distance between rows.
This study was established with a randomized complete block design and a 3×4 + 1 factorial arrangement, where the first factor corresponded to the population arrangement (rectangle, triangle and double row), the second factor was planting densities (2,000, 2,500, 3,000 and 3,500 plants/ha) and a relative control (1,700 plants/ha in a triangle), with three repetitions, for a total of 13 treatments. Each experiment unit consisted of a population of 26 plants established in an area of 120 m2, so the useful plot was in the center with 10 plants.
For each treatment, the harvest and post-harvest tasks were carried out in accordance with the quality protocols established by the marketing companies in the region: period of 12 weeks to harvest and a 35 mm thickness of the fruit in the second basal hand. In each treatment, four bunches from the plants located in the useful plot were selected, in which the planned determinations were made.
Were determinate physiological parameters in the different phenological phases of the crop [floral development, flowering and harvest] (Cayón, 2004) in the useful plot of each treatment, with an IRGA infrared gas exchange meter (LI-COR LI-6400XT®). The equipment was calibrated to a PAR of 1,000 μmol m-2 s-1 and a CO2 concentration of ± 350 ppm. The evaluated variables were: net photosynthesis (Pn, μmol mol-1 s-1), internal CO2 concentration (C i, μmol mol-1), stomatal conductance (g s , μmol m-2 s-1), transpiration (E, mmol H2O m-2 s-1), vapor pressure deficit (DPV, kPa) and leaf temperature (T i , °C). All readings were taken on the third leaf of the plants in relation to the flag leaf starting at 9:00 hours, when the best radiation conditions were present. Two readings were taken per day on the same plants and in each phase of the crop, for a total of four readings per week. With the recordings, the respective averages were determined. Additionally, the efficient use of water (WUE, µmol CO2 / mol H2O), the quantum efficiency (ϕ, mol O2 / mol photons) and the radiation use efficient (ɛ, g MJ-1) were determined (Solarte et al., 2010).
The bunches were harvested according to the commercial maturity point (between 10-12 weeks) based on the quality protocols established by the trader Uniban. The production was established with the bunch mass (BM; kg), number of hands (HN; No) and number of fruits (FN; No). The quality of the fruits was based on the length of the central fruit of the last hand (LnLH; cm), degree of the central fruit of the second hand (DSH; cm) and the degree of the central fruit of the last hand (DLH; cm). The BM was measured on a scale, starting from the gross mass of 10 bunches per treatment; the DSH and DLH were obtained with the diameter of the fruits in the middle part in the three central fruits.
The analysis of variance (ANOVA) was used, one of which excluded the control and another that included the control. When the control was excluded, the differences between the means of the treatments represented by the population arrangements were determined with the Tukey test (P≤0.05). To estimate the relationships between the planting densities, regression models were developed, and orthogonal contrasts were made to compare the interaction between the treatments. In the ANOVA that included the control, a randomized complete block design was used, where the treatments corresponded to the combinations of the factors and the control, which were analyzed with a Tukey test (P≤0.05). In addition, orthogonal contrasts were developed to compare the control with the rest of the treatments. Correlation analyses were carried out between the gas exchange variables and the production variables.
RESULTS AND DISCUSSION
Gas exchange
The ANOVA of the gas exchange variables that excluded the control reported that the planting densities had an effect on Pn in the flowering and harvest phases, as did the interaction of the factors involved in the flowering phase (P≤0.01-0.05). The C i was sensitive to the effects of the plantation density in the harvest (P≤0.05); the same was observed for E in flowering (P≤0.01-0.05). The ANOVA that included the control showed that the spatial distribution of plants (arrangements) and densities influenced Pn in the flowering and harvest phases (P≤0.01-0.05) but no differences were detected between the control and the rest of the treatments.
In the flowering phase, the double row arrangement with a population of 2,000 plants/ha reached a Pn rate of 23.94 μmol CO2 mol-1 s-1, while in the other arrangements, a decreasing trend was evident (Tab. 2). With the densities of 2,500 and 3,000 plants/ha, there were no differences between the population arrangements. This resulted from the fact that theleaf area facilitated a greater interception of radiation (Tab. 2). In addition, the triangle arrangement contributed to the increase in Pn when combined with a high density because of the increase in the leaf area (LA) and LAI, variables that favored the interception of PAR. On the contrary, the same condition in the rectangle arrangement saw a decrease in the Pn, possibly associated with the greater overlap of the leaves because of the short distance between the plants located in the furrows that promoted greater intraspecific competition, as expressed by Rodriguez et al. (2007). However, the distribution of plants in the triangle arrangement contributed to a greater PAR interception by associating it with photosynthesis when density increased (Tab. 2). The double row arrangement with the increase in density showed, in general, that Pn was higher than in the rectangle arrangement and stable with the lower densities, probably because of a greater penetration of light laterally to the plants (Tab. 2). The Pn rates in the flowering and harvest phases were higher than those mentioned for banana cultivation by Eckstein and Robinson (1995), Galán and Robinson (2013) and Rodríguez et al. (2007), who observed significant effects with increasing planting densities.
Table 2 Pn response depending on the population arrangement and planting density in the flowering phase in banana cv. Williams (Musa AAA Simmonds).
Population arrangement | Population density (plants/ha) | |||
---|---|---|---|---|
2,000 | 2,500 | 3,000 | 3,500 | |
Triangle | 19.27 B ab | 14.31 A c | 17.52 A bc | 22.91 A a |
Rectangle | 20.05 AB a | 17.14 A ab | 14.15 A bc | 12.07 C c |
Double rows | 23.94 A a | 15.84 A b | 17.01 A b | 17.75 B b |
Means with the same lowercase letter in the rows do not differ statistically according to the orthogonal contrasts (P≤0.05).
In the flowering phase, the Pn was explained by a quadratic model (R 2 = 0.78), where the maximum value (18.94 μmol CO2 mol-1) was recorded with the density of 2,500 plants/ha, noting a reduction for the higher densities (Fig. 1A). It is possible that the behavior of Pn as a function of plant density was also influenced by the spatial distribution of plants and other environmental variables, such as temperature and cloudiness, which may explain the R 2 in the model. However, starting at 2,500 plants/ha, the photosynthetic activity was disadvantaged, an aspect that was directly related to the variables of E and C i . This led to the inference that, as a result of overlapping leaves, which was more accentuated with the increasing densities, there was probably a decrease in the transpiration rates, a process that influenced the gas exchange rates, affecting the C i , an essential input for Rubisco enzymatic activity, a fundamental enzyme for photosynthesis (Parry et al., 2013). A decrease in Pn with increasing planting densities, according to Siles et al. (2013), is due to the increase in the shading level, which causes lower solar interception in the lower leaf strata. Cayón (2001) maintained that there are other factors that influence Pn, such as the age of the leaf, which is related to the development phase, generating changes in the internal concentration of CO2, the activity of Rubisco and the electron transport chain.
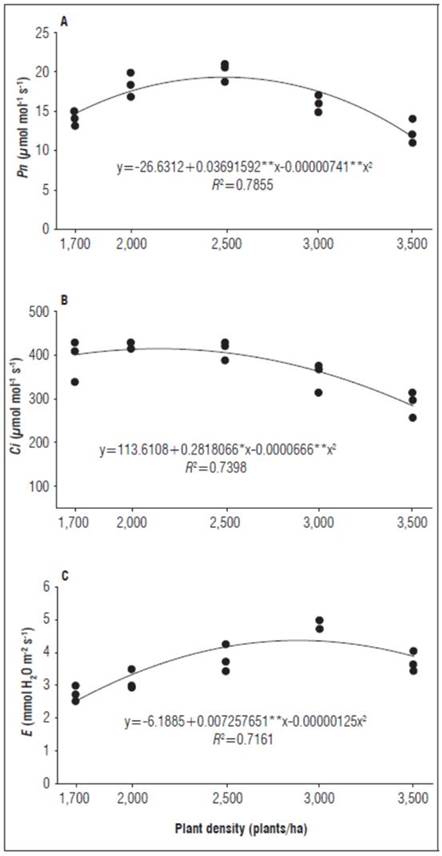
Figure 1. Response of Pn, C i and E as a function of population density: a) Pn in flowering, b) C i at harvest; c) E in flowering banana cv. Williams (Musa AAA Simmonds).
The Pn and E in the flowering phase and the As for the C i in the harvest phase were expressed by the quadratic models (R 2 = 0.73 and 0.71) (Fig. 1, B and C). The behavior of Pn and E at the low densities confirmed the relationship between these variables with increases or decreases densities occasioned by existing radiation and humidity conditions caused by changes in the number of plants and their different arrangements, that influence stomatal conductance and internal CO2 concentration (C i ) (Fig. 1, B and C). The results obtained for the lowest densities are in line with those of Liu et al. (2019), who stated that a decrease in C i is likely due to an increase in Pn, which causes a greater assimilation of CO2. Likewise, a positive correlation was observed between these variables since both processes depend on stomatal opening and closing, which regulate the flow of CO2 and water vapor (Enginneer et al. 2016; Cayón, 2001).
The analysis of variance that excluded the control showed that the WUE and ϕ responded to the planting density in the flowering and harvest phase, respectively. The analysis of variance that included the control showed significant differences between the treatments in the flowering phase for the variables indicated above; however, for the flowering ϕ, differences were detected between the control and the average of the rest of the treatments.
In the flowering phase, the WUE was represented by a quadratic model (R 2 = 0.91), observing that this variable decreased with increasing planting densities (Fig. 2A); the results allowed the inference that the density of 2,500 plants/ha offered a greater advantage in the WUE, while the low density of 1,700 and high density of 3,000 plants/ha require a greater use of water for biomass production. Related studies by Arantes et al. (2016) evidenced that, for different banana clones, the effects varied during the day. Cayón (2001) pointed out that Pn and E are positively correlated, and that their efficiency is concordant with the ontogeny of the foliar system. Lawson and Blatt (2014) clarified that Pn and E depend directly on WUE, a physiological process that in turn is linked to the dynamics of opening and closing of stomata because these structures regulate the flow of CO2 and water vapor and, therefore, directly affect Pn. It is important to highlight that the plantation densities generated environmental conditions that contributed to increasing photosynthesis in an attempt to improve the productivity of the banana crop.
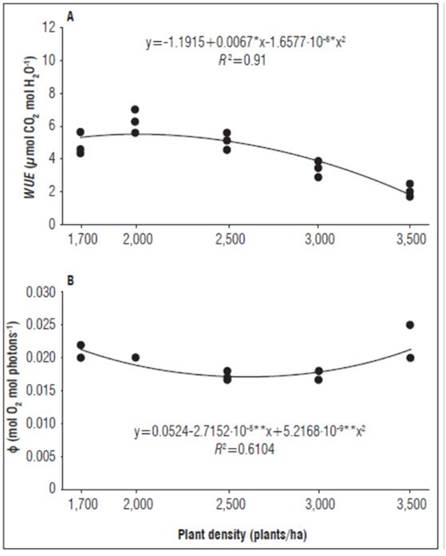
Figure 2. WUE and ϕ response as a function of population density in banana cv. Williams (Musa AAA Simmonds): a) WUE at flowering; b) ϕ at harvest.
The triangle arrangement generally favored the ϕ more than the other treatments; however, the double row arrangement also supported the latter (Tab. 2). These variables are important from the agronomic point of view for cultivation systems with high densities and irrigation systems; these data can be used as a reference point and are consistent with those indicated by Barrera et al. (2020) where the triangle distribution contributed to a greater bunch mass gain without affecting the exportable quality. The proposed values are similar to those of Santos et al. (2015) for the cultivation of bananas. The increase in densities was expected to improve the WUE because of the greater overlap of leaves that directly decreases temperature (Santos et al., 2015). An increase in production is expected with increased planting densities because a greater foliar area influences a greater interception in PAR (Rodríguez et al., 2007; Athani et al., 2009; Smith et al., 2010).
The ϕ was represented with a quadratic model that had an R 2 = 0.6104 (Fig. 2B), probably associated with other factors that intervene in the conversion of energy into biomass. Similar research has indicated that the evaluations carried out to measure the gas exchange were punctual values and were influenced by climatic conditions (Santos et al., 2015), not considered in the study. The ϕ decreased as the planting density increased, a trend that changed starting at 2,500 plants/ha (Fig. 2B). The initial indications were very likely due to the increase in transpiration rates generated by the increase in the number of plants and the mutual shading between the plants. The observations for the high densities could have been based on the need of the plants to make morphological couplings as a result of the low light intensity, a limitation that is compensated for with a larger leaf area as a strategy to increase the photosynthetic surface and improve the energy collection process (Jaramillo et al., 2009). The results indicated that the double row distribution (Tab. 2), in which the plants better intercepted the solar radiation and made use of it, which confirmed the observations of Cayón (2004) and Rodríguez et al. (2007), stressing that, in crops planted in rows, the greater penetration of light into the canopy and the degree of absorption of the incident PAR depend on the distances between rows and plants and the planting arrangement. Furthermore, the results are consistent with the report by Rocha et al. (2018) for banana ‘Prata’ and by Santos et al. (2015) for banana ‘BRS Princesa’. The data also indicated that the Pn and E were directly correlated with the g s , WUE and T i , while the WUE and Pn were inversely associated with the E and T i (Tab. 3), which is in line with the statements by Urban et al. (2017) that g s directly modifies water relations and photosynthesis and by Gariglio et al. (2007) that the amount of water that plants use and transpire depends on the species and the changing environmental conditions.
Table 3 Pearson's correlation between the variables of gas exchange and production in banana cv. Williams (Musa AAA Simmonds) in the triangle, rectangle and double row population arrangements in the flowering phase.
Arrangement | Variables | BM | NH | NF | LnLH | DSH | DLH |
---|---|---|---|---|---|---|---|
Triangle | Pn | -0,03 | 0,14 | -0,05 | -0,17 | -0,12 | -0,41 |
g s | 0,29 | -0,08 | -0,03 | 0,27 | 0,04 | 0,04 | |
C i | 0,16 | -0,13 | -0,30 | -0,05 | -0,05 | 0,37 | |
E | 0,35 | -0,32 | -0,30 | 0,27 | 0,32 | 0,57* | |
DPV | -0,12 | 0,00 | -0,22 | -0,31 | 0,18 | 0,07 | |
T i | 0,00 | 0,11 | -0,16 | -0,18 | 0,22 | -0,03 | |
Pn/E | 0,28 | 0,21 | 0,12 | -0,53* | -0,38 | -0,58* | |
Pn/RFA | 0,04 | 0,00 | 0,08 | 0,05 | -0,23 | -0,48 | |
Rectangle | Pn | 0,58* | -0,02 | -0,06 | 0,44 | 0,66* | 0,61* |
g s | -0,34 | -0,03 | -0,10 | 0,10 | -0,13 | -0,06 | |
C i | -0,08 | 0,14 | 0,00 | 0,02 | -0,14 | -0,02 | |
E | -0,45 | -0,30 | -0,48 | 0,23 | 0,09 | 0,31 | |
DPV | -0,06 | -0,13 | -0,18 | 0,02 | -0,03 | 0,16 | |
Pn/E | 0,64* | 0,22 | 0,24 | 0,08 | 0,38 | 0,22 | |
Pn/RFA | -0,21 | -0,21 | -0,13 | 0,49 | 0,57 | 0,52 | |
Doble row | Pn | -0,25 | -0,26 | -0,01 | -0,06 | -0,60* | -0,50 |
g s | 0,60* | 0,30 | 0,49 | 0,26 | 0,29 | -0,14 | |
C i | 0,22 | 0,13 | 0,07 | 0,14 | 0,23 | -0,46 | |
E | 0,49 | 0,29 | 0,31 | 0,25 | 0,12 | -0,29 | |
DPV | -0,50 | -0,35 | -0,46 | -0,03 | -0,34 | 0,06 | |
Pn/E | -0,50 | -0,32 | -0,15 | -0,22 | -0,50 | -0,01 | |
PnRFA | -0,25 | -0,25 | -0,02 | -0,16 | -0,49 | -0,61* |
BM: Bunch mass; NH: Number of hands; NF: Number of fruits; LnLH: Length of the last hand; DSH: Second hand grade; DLH: Last hand grade; *: Significant differences at 5% (P≤0.05).
Correlation between the variables of gas exchange and production
In the floral differentiation phase, there were no positive correlations between the production variables and field arrangement. It is possible that, at this phase of development, the pressure exerted by the densities and decreased leaf overlap did not influence the physiological response of the plants. Furthermore, the metabolic activity of leaves during the floral differentiation phase is high (Vargas-Calvo et al., 2015).
In the flowering phase with the triangle arrangement, the E was positively correlated with the variable DLH (Tab. 3). Higher transpiration rates mean more possibilities to increase g s because of greater stomatal opening, which is associated with higher Pn rates, as long as the environmental conditions oscillate in the ranges required for C3 plants, such as bananas (Ainsworth and Rogers, 2007). The aforementioned conditions favor the availability of assimilates to fill bunches; however, in C3 plants, the use of water is not very efficient because CO2 capture is done at the cost of large deliveries of water to the atmosphere (Qaderi et al., 2019). On the other hand, Gariglio et al. (2007) argued that E is associated with increased production, while Runkle (2015) stated that internal CO2 concentrations are favored by high levels of radiation and moderate or warmer temperatures.
The correlation of E with LnLH indicates that photosynthesis rates are associated with transpiration rates, which contribute to fruit filling, while photosynthetic efficiency can be affected by the degree of leaf exposure and the ability to intercept solar radiation (Tab. 3), which is consistent with the findings of Coelho et al. (2006), who indicated that water demand and crop productivity tend to increase linearly with E, which negatively affects the correlation of WUE with LnLH and DLH by increasing densities or changing distribution. These results are consistent with those indicated by Rodríguez et al. (2007), who reported that high banana plantation densities intercept a higher proportion of PAR, which can exceed 90% of the incident radiation because of the increase in LAI, a product of the planting density, obtaining lighter bunches. Thippesha et al. (2008) stated that, by reducing the distance between banana plants, the intensity of light available for the middle and lower strata of the plant is decreased, even with a low Pn, without affecting production per plant and time to flowering because the assimilated demand is supplied by the individual photosynthetic efficiency of the most exposed leaves, a process that benefits from the reduction in the temperature inside the canopy by making enzymatic processes more efficient.
In the rectangular arrangement, the Pn was positively correlated with the variables BM, DSH, and DLH (Tab. 3). The data marked the effect of the arrangements on the gas exchange variables since, the higher the Pn is, the higher the production of assimilates is, the higher the profit in the BM is, and the greater the filling of the hands is. The dependence observed in the variables was largely due to the changes generated by the spatial arrangements and population densities that influenced the magnitude of the incident PAR in the leaf strata and the intensity of the temperature on the photosynthetic surfaces despite the lack of significant effects on the thermal level of the leaves, which was pointed out by Santos et al. (2015), noting that increases in leaf temperature increase transpiration and reduce efficiency in the use of water and could also be associated with changes in photosynthesis because of variations in temperature, as highlighted by Hermida-Carrera et al. (2016) when stating that the thermal dependence of Rubisco's catalytic activity is a factor that limits the CO2 assimilation potential of plants.
In the double row arrangement, the Pn presented negative correlations with all the variables, while the g s and the E had a negative correlation with the DLH variable (Tab. 3). The relationships corresponded with the conditions generated in the different treatments as a result of the variations in the environment caused by the distribution of the plants in the different densities, with the magnitudes of each of the variables affected to different degrees. For example, by improving the interception of radiation with the arrangement of the leaves, the bunch filling increased, particularly with the lower densities, which favored the use of the photoassimilates by the fruits. Likewise, by generating conditions that favored higher photosynthesis rates, the VPD increased, with a consequent effect on the CO2 exchange rate and a lower contribution of assimilates for the fruits. This situation was evident during the development of the study, particularly in treatments with high plant densities, in which high transpiration rates and variations in other environmental variables occurred. Studies on this subject have discussed the relationship between photosynthesis and gas exchange with the production of assimilates, without neglecting the availability of water, a fundamental element in the soil-plant-atmosphere relationship (Landsberg and Waring, 2017).
In the harvest phase with the triangle arrangement, the Pn had a positive correlation with the variables BM, HN and DSH. A similar association was observed when the E and C i were compared with the variables BM, DSH and DLH; while the WUE was negatively correlated with the LnLH and DLH (Tab. 4). When the treatments considered the low densities, the CO2 exchange rates increased, improving the plants' biochemical processes, such as photosynthesis, as reflected in greater filling of the bunches and hands, as evidenced for the density 2,000 plants/ha (Tab. 2). On the other hand, the increase in planting densities influenced higher transpiration rates and a decrease in incident radiation inside the cultivation system, making the WUE and photosynthetic processes less effective, conditions that negatively affected bunch filling and manifested the lowest BM observed in the higher densities. Senevirathna et al. (2008) stated that net CO2 assimilation rates generally decrease with low radiation, but that the specific area of the leaf and the ratio of the leaf area increase proportionally with decreasing energy levels from the sun, while the chlorophyll a/b ratio decreases, reflecting greater efficiency in the use of light under shady conditions. On the contrary, Siles et al. (2013) and Rocha et al. (2017) argued that exposure to full radiation in banana crops increases yield but clarified that net photosynthesis curves vary and other biochemical parameters of photosynthesis are affected by shading and temperature conditions of the leaf.
Table 4 Pearson's correlation between the variables of gas exchange and production in banana cv. Williams (Musa AAA Simmonds) in the triangle, rectangle and double row population arrangement in the harvest phase.
Arrangement | Variables | BM | NH | NF | LnLH | DSH | DLH |
---|---|---|---|---|---|---|---|
Triangle | Pn | 0.39 | 0.24 | -0.08 | -0.16 | 0.09 | -0.09 |
g s | -0.12 | 0.08 | -0.20 | -0.11 | -0.08 | 0.44 | |
C i | 0.23 | -0.07 | -0.29 | -0.02 | 0.28 | 0.57** | |
E | 0.10 | 0.00 | -0.22 | -0.04 | 0.07 | 0.48 | |
VPD | 0.09 | -0.09 | 0.06 | 0.32 | -0.03 | -0.20 | |
T i | 0.30 | 0.15 | 0.22 | 0.35 | -0.10 | -0.23 | |
WUE | -0.28 | 0.21 | 0.12 | -0.53* | -0.38 | -0.58* | |
ϕ | 0.04 | 0.00 | 0.08 | 0.05 | -0.23 | -0.48 | |
ɛ | 0.78** | -0.42 | -0.34 | 0.19 | 0.20 | 0.55* | |
Rectangle | Pn | -0.14 | -0.49 | -0.42 | 0.39 | 0.19 | 0.40 |
g s | 0.42 | 0.19 | -0.04 | -0.08 | 0.68* | 0.47 | |
C i | 0.36 | -0.13 | 0.08 | 0.11 | 0.43 | 0.18 | |
E | 0.29 | 0.16 | -0.10 | -0.07 | 0.62* | 0.49 | |
VPD | -0.46 | -0.45 | -0.16 | 0.38 | -0.55 | -0.27 | |
T i | -0.55 | -0.53 | -0.26 | 0.44 | -0.54 | -0.23 | |
WUE | -0.55 | -0.53 | -0.26 | 0.44 | -0.54 | -0.23 | |
ϕ | -0.41 | -0.43 | -0.20 | 0.32 | -0.47 | -0.20 | |
ɛ | 0.45 | -0.54 | -0.21 | 0.33 | 0.36 | -0.42 | |
Double row | Pn | -0.30 | -0.18 | -0.29 | 0.08 | -0.12 | 0.27 |
g s | -0.55 | -0.39 | -0.42 | -0.35 | -0.47 | -0.46 | |
C i | -0.34 | -0.09 | -0.38 | -0.26 | 0.05 | -0.25 | |
E | -0.08 | -0.16 | -0.34 | 0.07 | 0.15 | -0.52 | |
VPD | 0.02 | 0.39 | 0.36 | -0.20 | -0.07 | 0.64* | |
T i | -0.24 | 0.26 | 0.21 | -0.22 | -0.11 | 0.66* | |
WUE | -0.24 | 0.26 | 0.21 | -0.22 | -0.11 | 0.66* | |
ϕ | -0.03 | 0.08 | 0.12 | 0.17 | -0.03 | 0.73** | |
ɛ | 0.67* | 0.70* | 0.86** | 0.00 | -0.18 | 0.26 |
BM: Bunch mass; NH: Number of hands; NF: Number of fruits; LnLH: Length of the last hand; DSH: Second Hand Grade; DLH: Last hand grade; *: Significant differences at 5% (P ≤ 0.05). **: Significant differences at 1% (P ≤0.01).
Finally, the ɛ was positively correlated with the variables BM, LnLH, and DLH (Tab. 4). In the harvest phase, the leaf age is advanced, so photosynthetic and transpiration processes are affected by the drop in chlorophyll content. These results agree with the observations of Cayón et al. (2004), who argued that there is a positive relationship between Pn, E and chlorophyll content in any phase of leaf development, and of Cayón (2001), who pointed out that changes occur in the phyllotaxis of leaves as development progresses, affecting the exposure of the leaf blade to radiation; however, Rodríguez et al. (2007) did not report effects on the insertion angle of the leaf from an increase in densities and population arrangements. Likewise, shady conditions can influence the aqueous balance of the stomatal cavity, as reflected in the turgidity of the guard cells and the opening of the stomata, with directly affects Pn and other associated metabolic processes (Lawson and Blatt, 2014).
In the rectangular arrangement, the Pn had a positive correlation with the variables LnLH, DSH and DLH; the same dependency was registered between the g s and the variables BM, HN, DSH and DLH; likewise, the ɛ depended on the variables BM, LnLH, DSH and DLH (Tab. 4). In the BM variable, the correlation with the Pn was antagonistic with respect to the correlations of the variables g s and E. This was explained by the fact that these processes are inclusive of each other, and their magnitudes increased or decreased proportionally in such a way that the positive correlations contributed assimilates to bunch filling; however, when VPD is high, stomatal closure occurs, which is reflected in falls in Pn. This is similar to that reported by Huntingford et al. (2015) for correlations between variables associated with g s , such as DPV and Pn. These results indicated that the photosynthetic activity of the banana plants was variable and conditioned by the spatial arrangements of the treatments. Santosh et al. (2017) indicated that climatic conditions change the water content of plants, gas exchange, and leaf temperature, variations that influence growth, development, and production. Moreover, Magnabosco and Cardin (2018) indicated that the photosynthesis rate of a leaf depends on multiple individual factors, each of which presents its own response to each environmental variable. Lanuakum et al. (2015) suggested that a negative relationship between canopy temperature and other performance attributes, such as temperature and plant density, influence banana productivity. Further, other related studies have indicated that planting densities and plant distribution influence the productivity of the banana crop (Rodriguez et al. 2007; Barrera et al., 2020).
In the double row arrangement, the VPD was positively correlated with the variables BM, HN, FN and DHL; the same was repeated for the variable ɛ (Tab. 4). The relationships showed that the low planting densities favored higher transpiration rates, which contributed positively to the increase in photosynthesis rates and a greater mass gain in BM, which is why these densities were considered more efficient. On the contrary, when the densities increased although there was greater interception of radiation, this fact was not reflected in the bunch size because the conditions generated by the competition between the plants and overlapping leaves affected the photosynthetic efficiency of the plants, as reflected in the low WUE. Lanuakum et al. (2015) reported high correlation coefficients in most of the studied characteristics, which was associated with the influence of solar radiation on the best crop yield. Therefore, by increasing planting densities, the exposure of leaves to light and the degree of insertion of these are modified until they are partially shaded, which somehow reduces Pn and E, affecting the balance of gas exchange in the plant (Cayón, 2001), which affects ɛ. Finally, these results agree with those of Hatfield and Dold (2019), who pointed out that this process affects an increase in the CO2 inside leaves in the presence of adequate levels of radiation and temperature, which normally cause an increase in photosynthetic rates and improve ɛ.
It was determined that the arrangement of the plants played an important role in the Pn; in addition, the triangle arrangement favored the variables associated with this process more. These results coincide with those reported by Surendar et al. (2015), who argued that the correlation between the number of leaves, the total chlorophyll content and the relative water content with yield is positive and significant. Santos et al. (2015) noted that increases in E rates are associated with growth in Pn rates, which leads to increases in production.
CONCLUSIONS
Banana cultivation cv. Williams (Musa AAA Simmonds) with a density of 2,500 plants/ha and arranged in a triangle or double rows, makes more efficient use of climate elements associated with photosynthetic activity under the environmental conditions of Uraba, Antioquia, an aspect that is positively reflected in the productivity and quality of fruits.
An increase in the number of plants and incorporation of alternatives for spatial field distribution are viable options for balancing harvest with variations in the availability of rain throughout the year, improving the income of farmers.