INTRODUCTION
The current challenge in tropical agriculture is increasing crop production with water scarcity, in terms of quality and quantity (Fuentes-Castañeda et al., 2016). Low quality water sources have been sought forirrigation, including the use of treated domestic effluents (Cifuentes‐Torres et al., 2020; Egbuikwem et al., 2020).
Appropriate systems for irrigation with treated domestic wastewater are needed to reduce risks of human and environmental contamination. An environmentally and sanitarily viable solution for the disposal of these waters is hydroponic cultivation. In hydroponics, according to Cuba et al. (2015), there is no direct contamination of the aerial part of the plants because the effluent only has contact with the roots.
The NFT (Nutrient Film Technique) system is the most commonly used; however, non-availability of electricity in some areas or secure source of power supply circulation of nutrient solution in the hydroponic channels (Silva et al., 2016), even for short time intervals, may lead to total loss of production. For this reason, the DFT (Deep Nutrient Flow Technique) system has emerged, in which a film of nutrient solution is kept in the tube during the cultivation period, making it possible to meet the water needs of plants in case there is a power interruption (Silva et al., 2016). Yet another advantage of this system is energy savings because the nutrient solution recirculation interval does not need to be as short as in the NFT system.
Studies on the DFT system adapted in PVC tubes have been conducted mainly with vegetables, such as coriander (Santos Júnior et al., 2015; Silva et al., 2018; Silva et al., 2020a), lettuce (Cova et al., 2017) and chicory (Silva et al., 2020b). According to Bione et al. (2014), in hydroponic cultivation, it is easier to diversify crops, and medicinal/condiment plants such as basil have more space.
Basil is used in cooking as a condiment, especially its fresh leaves. In addition, basil is used to extract essential oil, which is rich in phenolic compounds, with multiple uses in food, cosmetic, perfume and pharmaceutical industries (Heidari, 2012), and linalool is one of the most appreciated components of the essential oil (Veloso et al., 2014).
Basil has shown better yield in cultivation systems without soil (hydroponics) than in conventional systems (Saha et al., 2016). Therefore, it has been grown under different hydroponic conditions. Walters and Currey (2015) and Santos et al. (2019) found no significant differences for growth variables and production of basil under cultivation in the hydroponic systems NFT and DFT. The use of marginal quality water, such as brackish water, increased the yield of essential oil in the basil genotypes ‘Grecco a Palla’ (Gondim Filho et al., 2018) and ‘Gennaro de Menta’ (Azevedo Neto et al., 2019; Silva et al., 2019). In addition, the use of treated domestic effluents proved to be viable for the cultivation of this crop (Alves et al., 2019; Gonçalves et al., 2019) in DFT hydroponic system in tubes.
The objective of this study was to evaluate the biomass production and essential oil content and composition of two basil genotypes:‘Alfavaca Basilicão’ (Ocimum basilicum L.) and ‘Grecco a Palla’ (Ocimum minimum L.), in hydroponic systems with different plant spacing using treated domestic effluents.
MATERIALS AND METHODS
Cultivation conditions and study location
Two experiments with basil were carried out in a greenhouse (East-West orientation), from March to May (Experiment I) and from July to September (Experiment II) in 2015in the experimental area of the Post Graduate Program in Agricultural Engineering at the Soil and Water Engineering Nucleus at the Federal University of Recôncavo of Bahia (UFRB), located in the municipality of Cruz das Almas, Bahia State, Brazil (12º40’19” S, 39º06’23” W, and at an elevation of 220 m a.s.l.).
Experiment design and treatments
In both experiments, the basil genotypes ‘Alfavaca Basilicão’ (Ocimum basilicum L.) and ‘Grecco a Palla’ (Ocimum minimum L.) were used and analyzed individually. The experiments were carried out in a completely randomized design with four replicates in a 2×3 factorial arrangement. Only treated domestic effluents were used in Experiment I; two hydroponic systems (NFT and DFT adapted in PVC tubes) and three plant spacings in the hydroponic channels (0.20, 0.30, and 0.40 m) were evaluated. In Experiment II, the DFT hydroponic system, two types of water (tap water and treated domestic effluents) and three nutrient solution recirculation intervals (2, 4, and 6 h) were evaluated. Hydroponic cultivation is a closed system in which the nutrient solution is recirculated. The NFT hydroponic system is characterized by frequent circulation of the nutrient solution, generally with short intervals of 15 min. The DFT system has a layer of nutrient solution that remains in constant contact with the roots, as such, the plants do not undergo any water restriction, which means the interval time between the circulation of the nutrient solution can be extended, as has been shown by various authors (Silva et al., 2016; Silva et al., 2018; Santos et al., 2019; Silva et al., 2020a).
The treated domestic effluents came from the sewage treatment unitof the Bahia State Water and Sanitation Company (EMBASA), located in the municipalityof Muritiba, Bahia State, Brazil. To avoid large variations resulting from seasonality, a sufficient volume was collected and stored to conduct the experiments. The domestic sewage was treated with a grid and box of sand, removing all solid material, and then placed in a reactor and pond,where about 90% of the organic matter was digested, and pathogens such as viruses and bacteria were eliminated. The characteristics of treated domestic effluents and tap water are shown in table 1.
Table 1. Physio-chemical characterization of treated domestic effluents (TDE) and tap water (TW).
Water | pH | EC | P | K | N | Na | Ca | Mg | Cl | SO4 | CO3 | HCO3 | SAR |
---|---|---|---|---|---|---|---|---|---|---|---|---|---|
(dS m-1) | (mg L-1) | (mmol L-1)0.5 | |||||||||||
TDE | 7.16 | 1.00 | 0.05 | 29.32 | 21.85 | 138.00 | 15.00 | 18.96 | 175.83 | A | P | 238.51 | 5.55 |
TW | 6.41 | 0.25 | - | 6.63 | - | 39.79 | 20.40 | 21.12 | 66.29 | P | A | 25.01 | 2.93 |
Analyzed in Laboratory of Sanitary Engineering of Federal University of Campina Grande, Paraíba State, Brazil.
A - Absent; P - Present.
The water was used to prepare the nutrient solution of Furlani et al. (1999) (Tab. 2) and to replenish the volume consumed by the basil plants.
Table 2. Composition of standard nutrient solution of 17 utilized in the study.
Fertilizers | Conc | NH4 | NO3 | P | K | Ca | Mg | S | B | Cu | Fe | Mn | Mo | Zn |
---|---|---|---|---|---|---|---|---|---|---|---|---|---|---|
mg L-1 | ||||||||||||||
Calcium nitrate dihydrate | 750 | 7.5 | 108.75 | 142.5 | ||||||||||
Potassium nitrate | 500 | 65 | 182.5 | |||||||||||
Mono ammonium phosphate | 150 | 16.5 | 39 | |||||||||||
Magnesium sulphate | 400 | 40 | 52 | |||||||||||
Copper sulphate | 0.15 | 0.02 | ||||||||||||
Zinc sulphate | 0.30 | 0.07 | ||||||||||||
Manganese sulphate | 1.50 | 0.39 | ||||||||||||
Boric acid | 1.80 | 0.31 | ||||||||||||
Sodium molybedate | 0.15 | 0.06 | ||||||||||||
Tenso-Fe (FeEDDHMA 6%) | 35 | 2.08 | ||||||||||||
Concentration (Conc) | 24 | 173.75 | 39 | 182.5 | 142.5 | 40 | 52 | 0.31 | 0.02 | 2.08 | 0.39 | 0.06 | 0.07 |
Experiment structure
In Experiment I, both hydroponic systems had channels made of PVC tubes (6-m length and 0.075 m in diameter). The plants were spaced apart in the channels according to the corresponding spacing (0.20, 0.30, or 0.40 m), grown in circular holes with a 0.05 m diameter. The hydroponic channels were installed with zero and 3% slopes in the DFT (Fig. 1A) and NFT (Fig. 1B) systems, respectively. In Experiment II, the same structure was used; however, only the DFT hydroponic system was tested with a single plant spacing (0.20 m) in the channels. More details of the experiment structure can be seen in Alves et al. (2019).
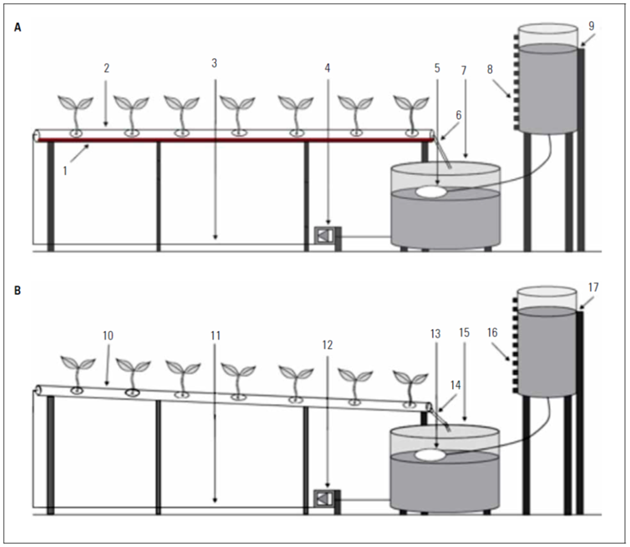
Figure 1. Illustration of the hydroponic systems DFT adapted in PVC tubes (A) and NFT (B) (adapted from Santos et al., 2018). 1. solution depth in hydroponic channel; 2 and 10. hydroponic channel installed with zero and 3% slopes, respectively; 3 and 11. PVC tube carrying the solution to the hydroponic channel; 4 and 12. electric pump to inject the solution into the hydroponic channel; 5 and 13. ballcock valve; 6 and 14. conduction of the excess solution to the solution tank; 7 and 15. plastic tank with nutrient solution; 8 and 16. transparent tube with a tape ruler; 9 and 17. water supply tank.
Seedling production
In Experiment I, basil seeds (ISLA Sementes®, Porto Alegre, Brazil) were sown on 6 March 2015 in 110-mL plastic cups containing coconut fiber substrate (home fabrication). Three seeds were sown per cup (individually per genotype) and covered with a thin layer of vermiculite. The bottoms of the cups were cut for root growth. The seedlings were manually irrigated until 15 days after sowing (DAS) using tap water, with an electrical conductivity (EC) of 0.25 dS m-1. Afterwards, thinning was carried out, leaving one seedling per cup, and the irrigation was performed with the nutrient solution of Furlani et al. (1999) at 50% concentration for 15 d.
For Experiment II, basil seeds were sown on 27 July 2015 on 2×2×2 cm phenolic foam (Oasis® Produtos Florais, Holambra, Brazil), planting one seed per cell, and covered with vermiculite. After germination, seedlings were irrigated daily with EC of 0.25 dS m-1 water until 15 DAS. After this period, the seedlings were transferred to a nursery (NFT system), where they received a nutrient solution (Furlani et al., 1999) at 50% concentration for 15 d. The irrigation in the nursery were controlled with an analog timer at intermittent intervals of 15 min, from 06:00 to 18:00 h. From 18:00 to 06:00 h, the nutrient solution was recirculated once every 2 h for 15 min.
Nutrient solution and experiment management
To prepare the nutrient solution, fertilizer salts were dissolved in the treated domestic effluents according to the recommendations of Furlani et al. (1999) (Tab. 2), with an electrical conductivity (ECsol) of 2.85 dS m-1 and pH of 6.4 in Experiment I. In Experiment II, the ECsol values were 2.23 and 2.98 dS m-1, and the pH was 5.8 and 6.2, respectively for the solutions prepared using tap water and treated domestic effluents. The ECsol and pH of the solutions were determined using an HI 15321 model conductivity meter and HI 3220 model pH meter (Hanna Instruments Brazil Exp. Imp. Ltda, Barueri, Brazil). During the experiments, the pH and ECsol values were monitored. When the pH values were outside the ideal range (between 5.5 and 6.5) for hydroponic cultivation, corrections were made by adding 30% HCl or 20 g L-1 of sodium hydroxide (Vetec Química Fina Ltda, Duque de Caxias, Brazil).
In Experiment I, the recirculation of solution in the cultivation channels in both systems (NFT and DFT) was similar to that used in the nursery (Experiment II). For Experiment II, the solution recirculation used the corresponding interval (2, 4, or 6 h) with one analog timer for each treatment; each circulation lasted 15 min.
The central part of each hydroponic channel received twelve 30-d old basil seedlings (individually for each genotype), using the nutrient solution at 100% strength (Furlani et al., 1999) (Tab. 2).
Evaluated variables
Biomass production. Harvests were carried out at 30 and 35 days after transplanting (DAT) in Experiments I and II, (in each plot) 6 and 12 plants, respectively. The plants were placed in paper bags and dried in a Q314M model forced-air oven (Quimis®, Diadema, Brazil) at 45ºC for 10 d (until constant weight) to quantify the shoot dry biomass (SDB).
Extraction and quantification of essential oil. The essential oil extraction was performed at the Phytochemistry Laboratory of UFRB. The oil was extracted from the leaves, which were separated from the stems after drying. In a 30 g sample of ground leafdry biomass (LDB) as previously established by Alves et al. (2015), the oil was extracted withhydrodistillation using a Clevenger apparatus (Clevenger, 1928). The distillation time (2 h) was counted from the moment the first drop of essential oil was deposited in the collector. The final volume of the extracted essential oil was verified in theClevenger collector. The collected volumes were used to calculate the oil content (OC %, v/m) (Eq. 1) and oil yield (OY, mL/plant) (Eq. 2).
where, U is the moisture content in the LDB sample, obtained after drying at 45°C.
Chemical composition of essential oil. The chemical composition analysis of the basil essential oil was done at the Chromatography Laboratory of the State University of Southwestern Bahia, Jequié, Brazil. The oil composition was analyzed with gas chromatography, using a Shimadzu GC-2010 gas chromatograph coupled to a Shimadzu MS-QP 2010 mass spectrometer.A DB-5 ms capillary column (5%phenyl and 95% dimethylpolysiloxane, 20 m × 0.18 mm i.d., 0.4 μm film thickness) was used, with helium as the carrier gas at a flow rate of 1.8 mL min-1. The injector temperature was 220ºC, and the detector temperature was 230ºC. The initial column heatingschedule was from 40ºC (for 2 min) to 240ºC at a rate of 3ºC min-1.
The constituents were identified by comparing their mass spectra with those of the device database and the NIST 62 database and from the literature (McLafferty and Stauffer, 1989). The analysis of total ion chromatogram was used in the calculation of Kovats indices, obtained by injecting a homologous series of linear hydrocarbons under the same analysis conditions (C4-C24), which was compared to those of Nist and Adams (2007).The relative percentage ofthe constituents was calculated using the peak area of each substance in the chromatogram.
RESULTS AND DISCUSSION
Biomass production
The shoot dry biomass (SDB) of the basil ‘Alfavaca Basilicão’ and ‘Grecco a Palla’ were not significantly affected (P>0.05) by the factors studied in Experiment I, with means ranging from 8.6 to 9.1 g/plant and 7.7 to 9.6 g/plant, respectively (Tab. 3). For the genotype ‘Grecco a Palla’ in Experiment II (Tab. 3), the SDB was significantly influenced (P<0.05) by the intervals of the nutrient solution recirculation, with the highest mean (20.6 g/plant) obtained under the interval of 2 h, in comparison to the intervals of 4 and 6 h.
Table 3. Summary of F test of the analysis of variance and mean values of shoot dry biomass (SDB) of the basil genotypes ‘Alfavaca Basilicão’ (AB) and ‘Grecco a Palla’ (GP) in Experiment I (hydroponic systems and plant spacings - PS) and Experiment II (types of water and nutrient solution recirculation intervals - IRNS).
Experiment I | SDB | Experiment II | SDB | ||
---|---|---|---|---|---|
Source of variation | AB | GP | Source of variation | AB | GP |
Systems | NS | NS | Type of water | NS | NS |
PS | NS | NS | IRNS | NS | * |
Systems x PS | NS | NS | Type of water x IRNS | NS | NS |
CV (%) | 31.62 | 21.04 | CV (%) | 31.62 | 22.09 |
Means | |||||
Systems | Types of water | ||||
DFT | 9.0 a | 8.4 a | Tap water | 13.5 a | 17.7 a |
NFT | 8.6 a | 8.6 a | Treated domestic effluents | 14.4 a | 15.3 a |
PS | IRNS | ||||
0.20 m | 9.1 a | 7.7 a | 2 h | 16.0 a | 20.6 a |
0.30 m | 8.6 a | 8.3 a | 4 h | 13.4 a | 14.0 b |
0.40 m | 8.6 a | 9.6 a | 6 h | 12.6 a | 14.9 b |
Means followed by different letters in the columns for each factor indicate significant differences according to the Tukey´s test (P≤0.05); * significant at P≤0.05 and NS - not significant according to the F-test; CV - coefficient of variation.
The results of present study demonstrate that it is feasible to cultivate the basil genotypes ‘Alfavaca Basilicão’ and ‘Grecco a Palla’ at a spacing of 0.20 m between plants in the NFT or DFT hydroponic system. These results corroborate the findings of Walters (2015), who reported that there was no significant difference in the SDB of basil (Ocimum basilicum L., cv. ‘Nufar’) with different plant spacings (0.10, 0.15, 0.20, 0.25, and 0.30 m) in the DFT system in basins.
The absence of a significant effect of the types of water on the biomass production of basilgenotypesdemonstrates that it istechnically feasible to use treated domestic effluents to prepare a nutrient solution for cultivation under hydroponic conditions. The absence of a significant effect from the water type may be a consequence of the composition although the treated domestic effluent had nutrients such as N (21.85 mg L-1) and K (29.32 mg L-1) (Tab. 1) but their amounts did not interfere with the basil crop growth, as seen with the nutrient solution of Furlani et al. (1999), and adequately met the nutrient requirements of the plants. In a study on basil grown in pots with peat substrate, Riera-Vila et al. (2019) reported a higher dry biomass yield when plants were irrigated with wastewater, as compared to those irrigated only with public-supply water without nutrients.
In the present study, no evaluations were made for the microbial contamination of the biomass because Cuba et al. (2015) indicated that there were no such risks in lettuce cultivated hydroponically using treated domestic effluents.
Basil essential oil content and yield
In Experiment I, there was a significant interaction (P<0.05) between the factors only for essential oil content (OC) of genotype ‘Grecco a Palla’ (Tab. 4). For Experiment II (Tab. 5), the essential oil yield (OY) was significantly influenced (P<0.05) by the nutrient solution recirculation intervals for genotype ‘Grecco a Palla’.
Table 4. Summary of F test of the analysis of variance and mean values for essential oil content (OC) and yield (OY) of basil genotypes ‘Alfavaca Basilicão’ and ‘Grecco a Palla’ with different plant spacing (PS) in the hydroponic channels of the NFT and DFT systems in Experiment I.
Source of variation | ‘Alfavaca Basilicão’ | ‘Grecco a Palla’ | ||||
---|---|---|---|---|---|---|
OC | OY | OY | OC | |||
Systems | NS | NS | NS | NS | ||
PS | NS | NS | NS | NS | ||
Systems x PS | NS | NS | NS | * | ||
CV (%) | 11.30 | 23.96 | 23.26 | 14.29 | ||
Means | ||||||
Systems | OC (%) | OY (mL/plant) | OC (%) | |||
PS | ||||||
0.20 (m) | 0.30 (m) | 0.40 (m) | ||||
DFT | 1.64 a | 0.104 a | 0.102 a | 1.98 aA | 1.40 bB | 1.81 aAB |
NFT | 1.83 a | 0.115 a | 0.109 a | 1.52 bA | 1.92 aA | 1.75 aA |
PS | ||||||
0.20 m | 1.76 a | 0.112 a | 0.097 a | |||
0.30 m | 1.76 a | 0.113 a | 0.097 a | |||
0.40 m | 1.70 a | 0.104 a | 0.122 a |
Means followed by lowercase letters in the columns indicate significant differences; for OC of genotype ‘Grecco a Palla’, lowercase letters in the columns compare the means of the hydroponic systems in each plant spacing, and uppercase letters in the rows compare the means of the plant spacing in each hydroponic system, according to the Tukey´s test (P≤0.05); * significant at P≤0.05 and NS - not significant according to the F-test; CV - coefficient of variation.
Table 5. Summary of F test of the analysis of variance and mean values for essential oil content (OC) and yield (OY) of basil genotypes ‘Alfavaca Basilicão’ and ‘Grecco a Palla’ using tap water (TW) and treated domestic effluents (TDE) with different nutrient solution recirculation intervals (IRNS) in the DFT hydroponic system in Experiment II.
Source of variation | ‘Alfavaca Basilicão’ | ‘Grecco a Palla’ | ||
---|---|---|---|---|
OC | OY | OC | OY | |
Types of water | NS | NS | NS | NS |
IRSN | NS | NS | NS | * |
Types of water x IRSN | NS | NS | NS | NS |
CV (%) | 16.59 | 37.89 | 14.88 | 27.22 |
Means | ||||
Types of water | OC (%) | OY (mL/plant) | OC (%) | OY (mL/plant) |
TW | 1.54 a | 0.137 a | 1.61 a | 0.159 a |
TDE | 1.65 a | 0.153 a | 1.81 a | 0.165 a |
IRNS | ||||
2 h | 1.58 a | 0.168 a | 1.82 a | 0.207 a |
4 h | 1.53 a | 0.129 a | 1.61a | 0.127 b |
6 h | 1.67 a | 0.138 a | 1.70 a | 0.151 ab |
Means followed by lowercase letters in the columns for each factor indicate significant differences according to the Tukey´s test (P≤0.05); * significant at P≤0.05 and NS - not significant according to the F-test; CV - coefficient of variation.
For the oil content of genotype ‘Alfavaca Basilicão’ in Experiment I (Tab. 4), the mean values were between 1.70 and 1.83%, regardless of the plant spacing in the cultivation channels and the hydroponic systems. For genotype ‘Grecco a Palla’, in the analysis of the interaction between plant spacing in the cultivation channels and the hydroponic systems, the highest plant density (spacing of 0.20 m between plants) in the DFT system led to a higher oil content (1.98%) than in the NFT system (1.52%). With the 0.30 m plant spacing, there was a higher oil content when the plants were cultivated in the NFT system (1.92%) than in the DFT system (1.40%). The largest spacing (0.40 m) had no significant differences (P>0.05) between the means obtained in the NFT and DFT systems, varying between 1.75 and 1.81%. When the hydroponic systems were analyzed individually, only DFT had a significant difference between the means as function of the plant spacing, with the lowest oil content observed in the 0.30 m spacing.
Based on the results, there were variations in the oil contents as a function of the basil genotypes according to the spacing used between plants. This type of behavior has been observed in other studies under field conditions, such as Daneshian et al. (2011), who studied two basil genotypes under three plant densities. Alemu et al. (2018) reported the highest mean oil content for genotype ‘BO-1’ at the smallest spacing between plants (0.20 m), whereas two other genotypes (‘BO-4’ and ‘BO-5’) with various plant spacings (0.20, 0.30 and 0.40 m) did not influence the oil content, and, in general, the mean oil content did not exceed 1%.
For Experiment II, the oil contents ranged between 1.53 and 1.67% for genotype ‘Alfavaca Basilicão’and between 1.61 and 1.82% for genotype ‘Grecco a Palla’, regardless of the type of water used and the nutrient solution recirculation intervals (Tab. 5). These values are within the same range observed in Experiment I (Tab. 4).
For oil yield in Experiment I, regardless of the spacing between plants and hydroponic systems, the mean values varied between 0.104 and 0.115 mL/plant for genotype ‘Alfavaca Basilicão’ and between0.097 to 0.122 mL/plant for genotype ‘Grecco a Palla’ (Tab 4).
For Experiment II (Tab. 5), the mean oil yield of genotype ‘Alfavaca Basilicão’ was between 0.129 and 0.168 mL/plant. For genotype ‘Grecco a Palla’, the highest oil yield (0.207 mL/plant)was recorded with the interval of 2 h.
Essential oil composition
In Experiment I (Tab. 6), 10 constituents were identified in the basil essential oil, namely: eucalyptol, linalool, methyl eugenol, trans-α-bergamotene, γ-cadinene, α-copaene, delta-guaiene, terpine-4-ol, β-elemene, and methyl cinnamate.Of the 10 constituentsfound in Experiment I, only methyl cinnamate was not found in Experiment II (Tab. 7). Some constituents were only found in some treatments, and,as a result, the statistical analysis only considered five main constituents: eucalyptol, linalool, trans-α-bergamotene, γ-cadinene andα-copaene for Experiment I; and eucalyptol, linalool, methyl eugenol(except for the genotype ‘Alfavaca Basilicão’),trans-α-bergamotene andγ-cadinene for Experiment II.
Table 6. Summary of F test of the analysis of variance and mean values of the follow-up analyses of the interactions between plant spacing in the hydroponic channels and systems using treated domestic effluents for essential oil compounds (%) of the basil genotypes ‘Alfavaca Basilicão’ and ‘Grecco a Palla’ in Experiment I.
Source of variation | F test | CV (%) | DFT | NFT | ||||||
---|---|---|---|---|---|---|---|---|---|---|
Systems (A) | ||||||||||
Plant spacings (B) | A | B | A×B | 0.20 m | 0.30 m | 0.40 m | 0.20 m | 0.30 m | 0.40 m | |
‘Alfavaca Basilicão’ | ||||||||||
Linalool | ** | ** | ** | 4.26 | 52.38 bB | 56.26 aB | 70.10 aA | 63.81 aA | 47.00 bC | 53.20 bB |
Trans-α-bergamotene | ** | * | ** | 10.52 | 16.74 aB | 20.74 aA | 12.18 aC | 11.50 bAB | 11.00 bB | 14.37 aA |
Eucaliptol | NS | ** | ** | 7.12 | 16.09 bA | 15.46 aA | 13.76 aA | 19.00 aA | 11.50 bC | 14.54 aB |
γ-cadinene | ** | ** | ** | 4.05 | 4.13 bA | 4.08 aA | 3.65 bB | 5.54 aA | 3.00 bC | 4.51 aB |
α-copaene | ** | ** | ** | 4.76 | 0.00 bA | 0.00 bA | 0.00 bA | 5.54 aA | 3.00 aB | 1.49 aC |
‘Grecco a Palla’ | ||||||||||
Linalool | ** | * | ** | 22.58 | 19.54 aB | 10.19 bB | 33.37 aA | 23.22 aB | 43.03 aA | 28.74 aB |
Trans-α-bergamotene | ** | * | ** | 25.39 | 35.03 aB | 58.15 aA | 19.74 aB | 23.92 aA | 16.06 bA | 24.06 aA |
Eucaliptol | NS | NS | * | 25.38 | 3.98 aB | 3.33 aB | 6.81 aA | 4.58 aA | 4.18 aA | 3.55 bA |
γ-cadinene | ** | * | ** | 11.65 | 7.55 aB | 10.58 aA | 5.42 bC | 6.28 aAB | 4.79 bB | 7.59 aA |
α-copaene | ** | ** | ** | 12.32 | 9.53 aA | 0.00 bC | 4.43 bB | 6.57 bB | 5.72 aB | 11.30 aA |
In the rows, the lowercase letters compare the means of respective plant spacing between hydroponic systems, and uppercase letters compare the means of the plant spacing in the respective hydroponic systems according to Tukey's test at P≤0.05; * and ** significant at P≤0.05 and P≤0.01, respectively, and NS - not significant according to the F-test; CV - coefficient of variation.
The five constituents of the oil evaluated in Experiment I (Tab. 6) were significantly influenced by the interaction between the factors in both basil genotypes. For Experiment II (Tab. 7), a significant interaction and single effects from the factors occurred for all constituents, except linalool(genotype ‘Alfavaca Basilicão’), trans-α-bergamoteneand methyl eugenol(genotype ‘Grecco a Palla’).
Table 7. Summary of F test of the analysis of variance and mean values of the follow-up analyses of the interaction between types of water and nutrient solution recirculation intervals (IRNS) for essential oil compounds (%) of the basil genotypes ‘Alfavaca Basilicão’ and ‘Grecco a Palla’ in Experiment II.
Source of variation | F test | CV (%) | Tap water | Treated domestic effluents | ||||||
---|---|---|---|---|---|---|---|---|---|---|
Types of water (A) | ||||||||||
IRNS (B) | A | B | A×B | 2 h | 4 h | 6 h | 2 h | 4 h | 6 h | |
‘Alfavaca Basilicão’ | ||||||||||
Linalool | NS | NS | NS | 5.47 | 63.64 aA | 59.84 aA | 59.47 aA | 62.88 aA | 58.27 aA | 61.67 aA |
Trans-α-bergamottin | ** | * | * | 11.93 | 14.75 aAB | 19.15 aA | 13.91 bB | 15.98 aB | 20.10 aAB | 22.49 aA |
Eucaliptol | ** | NS | ** | 5.35 | 9.96 bC | 14.12 aB | 18.40 aA | 13.18 aA | 9.36 bB | 6.55 bC |
γ-cadinene | NS | ** | * | 4.51 | 4.44 aB | 5.06 bA | 5.49 aA | 4.45 aB | 5.66 aA | 5.28 aA |
‘Grecco a Palla’ | ||||||||||
Linalool | NS | NS | * | 28.93 | 43.27 aA | 19.94 bB | 53.37 aA | 24.96 bA | 33.15 aA | 28.47 bA |
Trans-α-bergamotene | NS | NS | NS | 21.03 | 20.48 aA | 15.85 aA | 15.50 aA | 19.59 aA | 16.85 aA | 18.76 aA |
Eucaliptol | ** | * | ** | 36.30 | 8.49 aA | 3.23 bB | 11.50 aA | 0.00 bB | 7.42 aA | 4.67 bA |
Methyl eugenol | NS | NS | NS | 42.83 | 7.93 aA | 14.43 aA | 9.76 aA | 11.01 aA | 15.96 aA | 20.32 aA |
γ-cadinene | ** | ** | ** | 8.84 | 6.56 aA | 4.77 aB | 4.07 bB | 0.00 bC | 4.52 aB | 7.16 aA |
In the rows, the lowercase letters compare the means of the respective recirculation intervals of the nutrient solution between types of water, and uppercase letters compare the means of the recirculation intervals of the nutrient solution in the respective type of water according to Tukey´s test at P≤0.05; * and ** significant at P≤0.05 and P≤0.01, respectively, and NS - not significant according to F-test; CV - coefficient of variation.In Experiment I (Tab. 6), genotype ‘Alfavaca Basilicão’ had a higher percentage of linalool (70.10%) in the DFT system with the spacing of 0.40 m, while the NFT system had the highest percentage of linalool (63.81%) at the smallest spacing (0.20 m). For genotype ‘Grecco a Palla’, the NFT and DFT systems had the highest percentages of linalool, 43.03 and 33.37%, respectively, at the spacings of 0.30 and 0.40 m.
According to the literature, the chemical composition of basil essential oil is quite complex (Daryadar, 2017). In the present study, the chemical composition of the oil varied because of the genotypic differences of the basil, which partially explained the results. A complementary explanation of these differences (plant spacing in the channels of hydroponic systems) may be related to the inter or intraspecific competition of plants for light, air, water and nutrients (Maboko and Du Plooy, 2013; Skrypnik et al., 2019), modifying the assimilation capacity and, therefore, the secondary metabolism in favor of biosynthesis and the accumulation of different volatile compounds (Hassanpouraghdam et al., 2010; Ciriello et al., 2020).
In the study conducted by Arabaci and Bayram (2004) on basil cultivation in soil, linalool was the major constituent, varying according to the spacing used from one cycle to the other; in the first cycle, the highest percentage (76.46%) was obtained at a spacing of 0.20 m between plants under nitrogen applications, while, in the second and third cycles, the highest percentages (70.47 and 71.43%, respectively) were obtained at a spacing of 0.40 m without nitrogen applications.
For Experiment II (Tab. 7), regardless of the type of water and the nutrient solution recirculation intervals, the percentages of linalool varied between 58.27 and 63.64% for genotype ‘Alfavaca Basilicão’. For ‘Grecco a Palla’, there was no significant difference between the means of linalool percentages as a function of the recirculation interval using domestic effluent, ranging from 24.96 to 33.15%; however, when tap water was used to prepare the nutrient solution, the linalool percentages were higher with the intervals of 2 and 6 h, 43.27 and 53.37%, respectively.
CONCLUSIONS
It is technically feasibleto use treated domestic effluents to prepare nutrient solutions for the hydroponic cultivation of basil. In general, the shoot dry biomass and the oil content and yield of both basil genotypes were not influenced by the hydroponic systems (NFT or DFT), plant spacing (0.20, 0.30, and 0.40 m), or type of water (tap water or treated domestic effluents). The genotypes showed a mean oil content between 1.6 and 1.7%. Genotype ‘Grecco a Palla’ was more sensitive to the nutrient solution recirculation intervals, and higher means of biomass production and essential oil content were observed with the interval of 2 h. Linalool was the major constituent in the essential oil in the two basil genotypes.