INTRODUCTION
The cape gooseberry (Physalis peruviana L.) now occupies an important place in the global market for exotic fruits with a tropical origin because it has excellent nutritional characteristics since it is rich in proteins, vitamins, citric acid, phenols, carbohydrates and fiber (Maruenda et al., 2018). In addition, it is a source of minerals such as potassium, phosphorus (Mokhtar et al., 2018) and iron (Miranda and Fischer, 2021). These properties make the cape gooseberry fruit an ideal food that improvers the defenses of the human body and helps it to cope with diseases such as COVID-19 (Aman and Masood, 2020). Likewise, cape gooseberry fruits are a natural source of antioxidants and anticancer agents (El-Beltagi et al., 2019).
For more than 20 years, Colombia has remained at the forefront of global cape gooseberry production, and, in the last 10 years, the area planted with this crop has increased by 75%, reaching 1,311 ha by 2018 (Agronet, 2020). However, yields have decreased by 23% as the result of excessive costs for controlling pests and diseases and inadequate management of irrigation and fertilization, which causes fruit cracking and significant economic losses (Álvarez-Herrera et al., 2021).
In Colombia, the cape gooseberry enjoys good ecophysiological conditions for its commercial cultivation between 1,800 and 2,800 m a.s.l., with temperatures between 13 and 16°C and rainfall between 1,000 and 1,800 mm year-1 (Fischer and Melgarejo, 2020; Miranda and Fischer, 2021). Global warming, among others, increases the soil temperature causing water stress and thus yield and quality reductions (Bhatt et al., 2019; Fischer et al., 2022). Unfortunately, land at higher tropical altitudes is available if the temperature increases from climate change (Fischer et al., 2021a).
Given the demand for excellent quality fruits, in terms of color and size, farmers are forced to apply large amounts of fertilizers and water, which probably contribute to the physiopathy of cracking (Fischer et al., 2021b). In this regard, Álvarez-Herrera et al. (2012, 2014) found that cape gooseberry fruits present less cracking in the epidermis when they receive an adequate water supply.
Similarly, Gordillo et al. (2004) tested different irrigation levels in cape gooseberry plants and found that an irrigation coefficient of 1.2 increased the fruit yield per plant regardless of the applied fertilization treatments. Kochhar and Gujral (2020) affirm that Ca-deficient plants present little or no fruiting. And Álvarez-Herrera et al. (2015), with a net irrigation coefficient of 1.3 and 100 kg ha-1 of Ca under greenhouse conditions, observed a decrease in production, size and percentage of cracked fruits. Likewise, Fischer (2005) stated that cracking in gooseberry fruits is attributed to Ca deficiencies because this element has a large effect on the fruit quality because it maintains the structure and integrity of the cell wall and membrane and controls the levels of enzymes, such as pectinesterase (PE), that degrade the cell wall. In addition, Ca strengthens cell-to-cell adhesion (Kathpalia and Bhatla, 2018), which is important to reducing cracking in gooseberry fruits. Marschner (2012) stated that even a small increment of the Ca concentration in fruits are effective in reducing or preventing physiological disorders during postharvest.
To avoid cracking, irrigation and fertilization treatments have been visualized formerly that could reduce this physiological disorder; however, the impact that these applications will have on fruits at harvest must be known. The objective of this study was to characterize cape gooseberry fruits at harvest from plants watered with different irrigation regimes and calcium doses and to determine how much cracking affects fruit firmness, which will provide information for proper crop management.
MATERIALS AND METHODS
Location of the experiment
This experiment was developed in a greenhouse of the Faculty of Agricultural Sciences at the Universidad Nacional de Colombia, Bogota, which is located at an altitude of 2,556 m a.s.l., 74º5'20" W and 4º38'7" N. The mean temperature inside the greenhouse was 18°C, and the RH was 60%. The laboratory analyses were carried out in the Plant Physiology laboratory of the same Faculty at the Universidad Nacional de Colombia-Bogota.
Experiment design and treatments
A randomized complete block experiment design with 12 treatments was used. The blocking criterion was the irrigation frequencies (4, 9 and 14 d). The treatments had a 4×3 factorial structure. The first factor was the irrigation level (0.7, 0.9, 1.1 and 1.3 of the evaporation of the class A evaporimeter tank), and the second factor was the calcium dose (0, 50 and 100 kg ha-1). Each block corresponded to one repetition, which represented 36 experiment units (EU). Each EU consisted of two plants, which were planted in 20 L pots filled with white peat.
Experiment setup
Colombia ecotype cape gooseberry plants were transplanted 45 d after germination with a spatial arrangement of 2 m between plants and 2 m between rows. The usual cultural practices of tutoring in high V, pruning, fertilization and phytosanitary management of commercial production areas were carried out (Fischer et al., 2021c). Fruits were harvested at the color stage 5 and 6 of the calyx (Colombia Icontec, 1999), from 19 weeks after transplanting. The fertilization was divided into six applications, at 0, 3, 6, 8, 10 and 12 months after transplantation, the sum of which was 150, 220, 150, 60 and 40 kg ha-1 of N, P2O5, K2O, MgO and S, respectively, while 1, 3, 2 and 0.5 kg ha-1 of B, Zn, Cu and Mn were applied, respectively.
An irrigation system with two drippers per plant, 4 L h-1, was used. The Ca2+ doses were applied in the crown form around the plant, incorporating it in the substrate, distributed on a monthly basis with three doses during the first three months because of the low mobility of calcium in the soil. The irrigation level was applied according to equation (1).
where, Etp was evapotranspiration in mm measured in a class A evaporimeter tank installed inside the greenhouse; C multiplier coefficient according to the treatments; A area of the pot (254.4 cm2);
Response variables
At the second crop harvest (19 weeks after transplantation), which had the highest production (Álvarez-Herrera et al., 2021), among ten harvests, response variables were measured. The total soluble solids (TSS) were determined, for which 5 mL of gooseberry fruit juice was taken from each EU and placed in a Hanna HI 96803 digital refractometer (Hanna Instruments, Spain).
The total titratable acidity (TTA) was determined by measuring the volume of NaOH used in the titration of a solution brought to pH 8.2, 5 mL of fruit juice with 3 drops of phenophthalein added. Equation (2) was used.
where, A was volume of NaOH used; B normality of NaOH (0.097 meq mL-1); C equivalent mass expressed in g of citric acid (0.064 g meq-1); D mass in grams of the sample used (5 g).
The maturity index (MI) was calculated by dividing the TSS by the TTA. The pH measurement was determined with a HANNA HI 8424 digital potentiometer (Hanna Instruments, Spain) in 5 mL of juice. The fruit firmness was measured in ten healthy fruits and ten cracked fruits per EU with a PCE-PTR200 digital penetrometer (PCE-Ibérica, Spain).
The calcium concentration [Ca2+] in fruits was quantified following the protocols for the repair of the plant tissue sample (POE-F001), calcination and digestion with HCl (POE-F002), and atomic absorption of Ca (POE F003), prepared by the Water and Soil Laboratory of the Faculty of Agricultural Sciences of the Universidad Nacional de Colombia.
Analysis of data
An analysis of variance (Anova) was carried out for a completely randomized block design with factorial treatment structure to elucidate the existence of significant differences between irrigation frequencies (blocks) and between treatments (water level x frequency) and factors for the measured response variables. Additionally, a Tukey mean comparison test (P<0.05) was performed to classify the levels of the evaluated factors with SAS v. 9.2.
RESULTS AND DISCUSSION
Total soluble solids (TSS)
The TSS were significantly affected by the increase in the amount of water applied to the cape gooseberry plants. The highest irrigation coefficient produced fruits with 10% less TSS (Fig. 1). The values ranged between 16.8 and 18.7%, higher than the 14.8% reported by Bazalar et al. (2019) and similar to the report by Bazalar et al. (2020) of between 14.0 and 18.7% and from 12 to 15% for fruits in 3 stages of maturity (Garzón-Acosta et al., 2014).
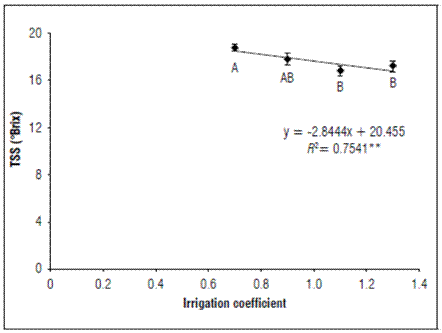
Figure 1. Effect of the irrigation coefficient on total soluble solids (ºBrix) in gooseberry fruits of plants planted in peat moss and subjected to different irrigation regimes and calcium nutrition. Means with different letters indicate statistical differences according Tukey test (P<0.05). Vertical bars indicate standard error (n=9).
The irrigation frequency showed significant differences in the TSS of the cape gooseberry fruits (Fig. 2). As the irrigation frequency became longer, the TSS increased since, when going from an irrigation frequency of 4 d to 14 d, the TSS increased approximately 7% on average. This increase in TSS as a result of a decrease in the irrigation level has been reported by several authors for different fruits, such as the apple (Soliman et al., 2018), peach 'Rubirich' (Guizani et al., 2019), melon (Özbahçe et al., 2014), grapevine (Cataldo et al., 2021) and tomato (Agbemafle et al., 2014). Similarly, decreasing the irrigation frequency increased the TSS in pepper (Marín et al., 2009).
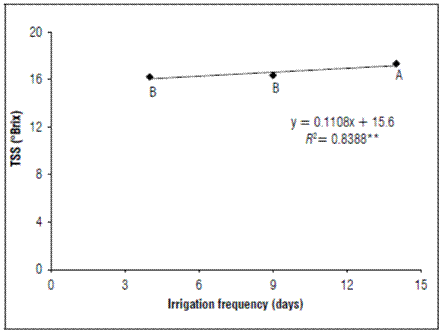
Figure 2. Effect of the irrigation frequency on total soluble solids expressed in Brix degrees in gooseberry fruits of plants planted in peat moss and subjected to different irrigation regimes and calcium nutrition. Means with different letters indicate statistical differences according Tukey test (P<0.05). Vertical bars indicate standard error (n=9).
The TSS increase was probably due to the fact that a stress-induced osmotic adjustment occurred, generating a greater split of reserve carbohydrates to the simpler sugars used in respiration (Yahia et al., 2019). The effect results from a lower water supply, so the fruits have a lower moisture content; therefore, the concentration of dry matter increases (Ripoll et al., 2014) and the TSS are greater, known as a “concentration effect”. Increases in free sugars in fruits occur through the hydrolysis of starch reserves caused by α-amylase, β-amylase and starch phosphorylase, enzymes whose activity increases dramatically during fruit ripening (Yahia et al., 2019). Under conditions of water stress, sucrose phosphate synthase increase their activity (Duan et al., 2021), thus increasing the sucrose concentration gradient between leaves and fruits, which results in greater transport of photoassimilates towards the fruits, and a possible increment of TSS. Also, the TSS values were high, probably because the greenhouse temperature was between 3 and 5°C on average higher than the outside temperature; therefore, the water stress reduced the absorption of water by osmosis but not with the same proportion as the amount of sugars, so their concentration was higher in the fruits (Marín et al., 2009).
The calcium doses did not affect the TSS concentration in the fruits. There are reports where pre-harvest applications of calcium did not affect the TSS in blueberry fruits (Lobos et al., 2021), apples (Fallahi and Mahdavi, 2020) or pepper (Marín et al., 2009); however, according to Rahman et al. (2016), fruits generally present a higher amount of TSS when they do not receive calcium applications, as seen in this research, where the TSS value was 15.7% without calcium applications but, when calcium was applied in doses of 100 kg ha-1, the TSS value was 15.3%. Also, Khan and Ali (2018) reported that the results of preharvest Ca applications, in many cases, are erratic and even contradictory, possibly because of genotypic differences among varieties and fruit species or Ca concentrations and formulations used (Lara, 2013).
Total titratable acidity (TTA)
The irrigation level significantly (P<0.05) affected the TTA of the gooseberry fruits. The TTA ranged between 2.1 and 2.8% (Fig. 3). The TTA presented a linear adjustment with respect to the increase in the application of water, as the irrigation coefficient increased, the TTA decreased. The irrigation frequency did not present significant differences in the TTA. When water was supplied to the crop every 4 d, the fruits presented an acidity of 2.13% but, when it was done every 14 d, the TTA was 2.16%. Marín et al. (2009) applied different irrigation frequencies to pepper and found no differences in the TTA. The calcium applications to the soil did not affect the TTA of the cape gooseberry fruits, as reported for pepper (Marín et al., 2009), blueberries (Lobos et al., 2021) and pear (Wojcik, 2012). However, a calcium deficiency can decrease the integrity of the cell membrane; thus, the respiration will increase, and the concentration of acids will be lower (Bhatla, 2018).
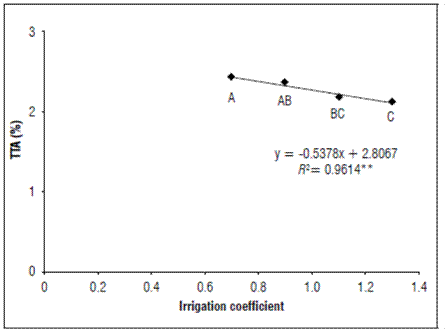
Figure 3. Effect of the irrigation coefficient on total titratable acidity in gooseberry fruits of plants planted in peat moss and subjected to different irrigation regimes and calcium nutrition. Means with different letters indicate statistical differences according Tukey test (P<0.05). Vertical bars indicate standard error (n=9).
During ripening, a decrease in organic acids occurs in many fruits (Vallarino and Osorio, 2019). This loss is mainly due to the use of these compounds as respiratory substrates and as carbon skeletons for the synthesis of new components. With a water deficit, fruits probably present a higher respiration, which causes the conversion of acids to sugars more quickly (Bhatla, 2018) or the higher respiration affects citric and malic acids content directly because of its function in the Krebs cycle (Lambers and Oliveira, 2019). However, according to the results, the fruits from plants with a greater application of water had a greater amount of water in their tissues, which probably caused the concentration of acids to decrease as a result of the dilution effect (Fischer and Martínez, 1999). Thus, the amount of acids was similar in all treatments. In cape gooseberry fruits, citric acid constitutes 85% of the organic acids (Novoa et al., 2006). Medyouni et al. (2021) reported that, when tomato plants suffered water stress, they reduced water absorption and increased the production of acids in the fruits as a mechanism of adaptation to stress, where ascorbic acid and total carotenoids stand out. A similar result was found for the cape gooseberries in this study and in another study on the tomato (Patané et al., 2011).
Maturity index (MI)
The level and the frequency of the irrigation presented statistically significant differences for the maturity index (Tab. 1), which presented an average of 7.89. This MI value was within the range reported by Novoa et al. (2006), from 7 to 8. Batista-Silva et al. (2018) stated that, during ripening, the proportion of acids decreases and the concentration of sugars increases, making the MI the degree of maturity higher. The calcium doses applied to the cape gooseberry plants did not have a significant effect on the MI values of the fruits (Tab. 1), which agrees with Arah et al. (2015) who reported that calcium has little influence on MI but has a large influence on prolonging postharvest life. Similarly, Marín et al. (2009) tested different calcium doses and did not find significant differences in TTA, TSS or MI pepper; however, they stated that these values varied when the state of maturity changed.
Table 1. Effect of the irrigation coefficient, calcium dose and irrigation frequency on the total soluble solids (TSS), total titratable acidity (TTA), maturity index (MI), pH and firmness of gooseberry fruits from plants grown in the greenhouse.
Factor | Level of the factor | TSS (°Brix) | TTA (%) | MI | pH | Firmness of healthy fruits (N) | Firmness of cracked fruits (N) |
---|---|---|---|---|---|---|---|
Irrigation coefficient | 0.7 | 18.73±0.30 a | 2.42±0.08 a | 7.76±0.19 b | 3.92±0.02 a | 9.30±0.42 a | 7.29±0.36 a |
0.9 | 17.75±0.50 ab | 2.35±0.05 ab | 7.56±0.27 b | 3.92±0.02 a | 9.61±0.38 a | 6.21±0.41 a | |
1.1 | 16.80±0.42 b | 2.18±0.05 bc | 7.71±0.20 ab | 3.94±0.03 a | 9.84±0.33 a | 6.70±0.35 a | |
1.3 | 17.15±0.44 b | 2.11±0.07 c | 8.13±0.29 a | 3.92±0.02 a | 9.59±0.35 a | 6.35±0.33 a | |
Calcium doses (kg ha-1) | 0 | 17.78±0.39 a | 2.27±0.06 a | 7.82±0.21 a | 3.92±0.02 a | 10.59±0.26 a | 6.89±0.25 a |
50 | 17.66±0.37 a | 2.29±0.06 a | 7.70±0.18 a | 3.92±0.02 a | 10.76±0.34 a | 6.71±0.41 a | |
100 | 17.40±0.48 a | 2.24±0.06 a | 7.76±0.24 a | 3.92±0.02 a | 10.69±0.36 a | 6.31±0.26 a | |
Irrigation frequency (days) | 4 | 17.22±0.44 b | 2.13±0.07 a | 8.08±0.21 b | 3.87±0.01 b | 9.43±0.32 a | 6.83±0.25 a |
9 | 17.06±0.36 b | 2.16±0.06 a | 7.89±0.10 b | 3.90±0.01 b | 9.77±0.33 a | 6.97±0.41 a | |
14 | 18.54±0.30 a | 2.16±0.05 a | 8.58±0.18 a | 3.98±0.02 a | 9.55±0.31 a | 6.12±0.26 a |
Means with different letters in the same column and classified by factor indicate significant differences between the factor levels according to Tukey's test (P≤0.05).
Cape gooseberry fruits have an MI that increases linearly during the different ripening phases (Fischer and Martínez, 1999). This results from the fact that the TSS increase from the green maturity state (0) to the maturity state 5 (Herrera, 2000), then decline until harvest. The TTA decreases constantly throughout the ripening period (Fischer, 1995). This behavior includes decreased acid levels and increased sugars because of the increase in the translocation of sugars from the leaves and towards the fruits and the mobilization and degradation of the starch reserves within the fruits (Yahia et al., 2019). However, the MI may vary when the cape gooseberry plants receive different irrigation coefficients; that is, increasing the amount of water applied decreases the MI decreases, which suggests that applying a larger irrigation level (coefficient of 1.3) affects the concentration of sugars to a greater extent than acidity. The MI is similar to that of tomato fruits since, when plants are subjected to water stress, the MI increases (Beckles, 2012).
pH value
This variable showed significant statistical differences between the applied irrigation frequencies. The irrigation frequency of 14 d presented the highest pH value (Tab. 1). Neither the irrigation level nor the calcium dose significantly affected the pH behavior of the gooseberry fruits at harvest. The pH averages found for the cape gooseberry fruits were similar to those obtained by Bazalar et al. (2019), who reported average values of 3.92, were lower than those reported by Mubarok et al. (2019), from 5.0 to 5.2, and were higher than those registered by Herrera (2000), who found values that ranged between 3.5 and 3.7 for fruits at maturity stage 5 and 6, respectively.
Vallarino and Osorio (2019) reported that the acidity of fruits depends on the content of organic acids stored in the vacuole, which decrease as the fruit matures because many are transformed into sugars, a process called gluconeogenesis. During fruit filling, a large part of the accumulation activity is given by simporte, where H+ ions play an important role since they are part of the formation of substrates, such as sucrose and glucose. Their concentration at the vacuolar level decreases, and the pH is slightly increased (Lal, 2018). As the irrigation frequency became longer (14 d), the pH of the fruits increased, so they were less acidic, as reported by Marín et al. (2009) in pepper, who also found no effect from calcium doses on pH. No significant effect of the irrigation coefficient and the calcium doses was registered (Tab. 1). Likewise, as there was no effect of the calcium doses in the TTA, it did not affect the pH significantly.
Firmness
The water level and the frequency of the irrigation did not present significant differences in the firmness of the cape gooseberry fruits (Tab. 1), which had an average value of 9.91 N and a standard deviation of 0.55, lower than that found by Amézquita et al. (2008) and Balaguera-López et al. (2021), 11.19 and 15 N, respectively, and that obtained by Ciro et al. (2007), 13.1 N for ripe fruits, 16.8 N for dark fruits and 23.2 N for green cape gooseberry fruits.
Some authors have confirmed that reducing irrigation increases fruit firmness in the pear (Bayona-Penagos, 2017), blueberry (Almutairi et al., 2017), apple (Faghih et al., 2021), and peach (Zhou et al., 2017; Rufat et al., 2010). This decrease is attributed to the fact that water stress reduces the size of fruits, and smaller fruits tend to be more compact and firmer than large fruits (Faghih et al., 2021). Likewise, Patané et al. (2011) reported that tomatoes increase firmness when smaller amounts of water are applied because the turgor pressure decreases, which results in less pressure on the cell wall, making the epidermal elasticity higher. However, Porro et al. (2010) found that, in vines, a decrease in the amount of water applied reduced the firmness of the fruits, as did García-Tejero et al. (2010), who reported that a water deficit during the growth of citrus fruits generates a breakdown of the cell wall polymers and a greater reduction of the osmotic potential of fruits and their firmness.
For the cape gooseberry, the firmness could have increased with the treatments with less water application; however, they had smaller fruits, resulting in similar values since smaller fruits generally tend to present greater firmness because of the higher cell density, which makes cells more compact and rigid, and the greater availability of calcium assigned for the composition of the cell wall, which increases fruit firmness and resistance (Lima et al., 2021). This probably explains why there were no significant differences between the different irrigation regimes.
The calcium doses applied to the cape gooseberry plants did not significantly influence the firmness of the fruits at harvest. However, when Ca2+ was applied, the fruits presented 1.6% more firmness. Despite the absence of differences in the levels of resistance to penetration, the fruits that received Ca2+ showed 11% less cracking (Álvarez-Herrera et al., 2012), which highlights the importance of calcium applications in the quality of fruits since it has the ability to provide greater resistance to the cell wall and thickens it. Likewise, calcium has a large effect on firmness because this element maintains the integrity and structure of the cell membrane and decreases the activity levels of enzymes that degrade the cell wall, such as pectinesterase (PE) because it strengthens cell-to-cell adhesion and maintains the relationship of stiffness and extensibility (Kathpalia and Bhatla, 2018).
The application of calcium did not have a marked effect on the firmness of the fruits since it had a reduced effect when combined with irrigation treatments because these conditions may have decreased as a result of the excess or deficient water, the quantity of calcium absorbed by the plant, the amount of calcium, and the water supplied to the fruit, as reported by Agbemafle et al. (2014) in tomatoes. In general, the Ca-doses did not affect the measured fruit quality parameters, and also Garzón-Acosta et al. (2014) found only 0.79% of cape gooseberry fruits cracked of plants which were grown without Ca applications.
When evaluating the firmness of the cracked fruits, the Anova did not showed significant differences for any of the studied factors (Tab. 1). However, firmness of the cracked fruits per treatment presented average values of 6.64 N, 33% lower than the firmness of the healthy fruits. This strong tendency in firmness decrease of the cracked fruits is attributed, according to Jiang et al. (2019), that prior to cracking, increases in the activity of enzymes such as polygalacturonase and expansins occur during maturation, which together disassemble the polysaccharide network of the cell wall allowing softening. Likewise, these authors mention that the incidence of cracking was directly and significantly correlated with the low solubility of the cell wall pectins and the cellulose content, but not with the concentration of Ca2+. On the other hand, Niu et al. (2020) stated that the metabolic pathways of galactose and phenylpropanoid biosynthesis are involved in fruit ripening and cracking. However, some authors have found effective mechanisms to reduce cracking, by bagging fruits, spraying calcium chloride, applying 6-Benzyl amino purine in tomato (Wang et al., 2021) and applying of gibberellins in cape gooseberry (Amézquita et al., 2008).
Calcium concentration in the fruits
The Ca2+ in the cape gooseberry fruits did not show significant differences between the calcium treatments and maintained an average percentage of dry mass value, 0.07663±0.00215. This behavior is similar to that found in sweet cherries by Winkler and Knoche (2021), who tested different applications of combinations of Ca2+ and surfactants and found no differences in the calcium concentration in the fruits. Likewise, Poovarodom and Boonplang (2010) stated that calcium applications to the soil decreased the presence of physiological disorders in mangosteen; however, the Ca2+ in the pulp was similar to that of the control, as reported by Pessoa et al. (2021) for pears, where Ca2+ applications could be more effective in the early stages of fruit development and where the accumulation of calcium in fruits depends mainly on the Ca2+ transported through the xylem because of the low mobility required in the phloem. Hocking et al. (2016) reported that an increase in calcium supplements in fertilization allows an increase in Ca2+ in the leaves but not necessarily in the organs with little transpiration, such as fruits, since the plants have developed mechanisms to restrict the transport of Ca2+ to these organs, which have a low calcium requirement and a high membrane permeability that favors rapid cell expansion.
CONCLUSIONS
The firmness of the cape gooseberry fruits was not affected by the application of different water levels and irrigation frequencies. Cracked gooseberry fruits are less firm than healthy fruits. The highest irrigation coefficient applied to cape gooseberry plants (1.3) produced fruits with 10% less TSS and caused a decrease in the TTA. The irrigation frequency of 14 days generated an increase in the TSS and pH values. The calcium doses did not affect the TSS, TTA, and pH values. Therefore, it can be concluded that incremented irrigation coefficients (up to 1.3) increase the quality of cape gooseberry fruits.