INTRODUCTION
Irrigation is a fundamental element in agricultural production because it increases production, improving product quality, sustainable land use intensification, and food security (FAO, 2010). Mercado et al. (2011) saw inadequate irrigation management, excessive rainfall and soil type are factors that cause different degrees of drainage and salinization problems in agricultural areas, resulting in degradation and loss of the productive potential of soils and directly affecting the development of intensive agriculture. These problems threaten the sustainability of irrigated agriculture, especially in dry regions. The deterioration of irrigation water quality and excessive use of water for leaching lead to soil salinity problems (Devkota et al., 2015).
The mobilization of salts in soil is highly variable between different irrigation zones. According to Ben Ahmed et al. (2012), salinity depends on several factors, such as the amount of salt in the water, the texture of the soil, the distance from the intake to the district, hydrogeological characteristics, irrigation, drainage, and climatic conditions (rain regime, average temperature ) (Duncan et al., 2008). For the Institute of Hydrology, Meteorology and Environmental Studies (IDEAM), salinization is the process of increasing, gaining or accumulating salts in soil, that is, increasing salinity. Generally, increasing salts in soil and high concentrations affect the physicochemical and biological characteristics of soil and ecosystem functions, including the development of plants, especially crops and soil biota. Consequently, it is a soil degradation process (Colombia IDEAM et al., 2017).
Leached water contains agricultural additives (including toxic cations, nutrients, herbicides, and pesticides) and natural contaminants that would not have been mobilized without irrigation. Agrochemicals in water can cause mortality in fish, toxicity for humans, bioaccumulation in sediments, and eutrophication in lentic bodies, among other effects (Colombia IDEAM et al., 2019). Arevalo et al. (2012) stated that the agricultural sector is first in terms of water consumption, over other economic activities in Colombia. According to Colombia IDEAM et al. (2019), the cultivation of bulb onions had a water footprint of 15.9 million m3 year-1, 0.5% of the domestic use in agriculture, in 2018.
The onion (Allium cepa L.) is the most cultivated and important species of the Alliaceae family in terms of volume consumed and the economic value of its production. For Colombia, the Information and Communication Network of the Colombian Agricultural Sector (Agronet, 2021) reported that 2019 saw domestic production at 292,333.22 t in 14,188.95 ha, with a yield of 20.6 t ha-1. The Department of Boyacá contributed most to domestic production in that year, with 44.44%, 135,755.24 t in 5,798.30 ha, and a yield of 23.41 t ha-1. According to the results of the Municipal Agricultural Evaluations (EVA), this department had 43 municipalities that produced bulb onions in 2017. At the municipal level, the larger harvested area was in Tibasosa with 1,414 ha and Toca with 1,080 ha (Colombia MADR, 2019). For the municipality of Samaca, the production of bulb onions in 2019 was 13,480 t, with a yield of 22.47 t ha-1 (Agronet, 2021).
Castellon et al. (2015) reported that the use of water for agriculture is closely related to the concept of fertigation through the quality parameter, which encompasses the concentration of dissolved salts, electrical conductivity (CE), relative presence of sodium (RAS), content of carbonates and bicarbonates (which condition the pH), concentration of chlorine, boron, iron and manganese, and nutrients such as calcium, magnesium and sulfates, which determine the final balance in fertilizer applications when preparing a nutrient solution for fertigation.
The agricultural sector has the largest consumption of water worldwide; therefore, irrigated agriculture must be efficient to be environmentally friendly and achieve economic sustainability (Flórez-Tuta et al., 2013). Water quality monitoring is a high priority for determining current conditions and long-term trends for effective management (Nishanthiny et al., 2010). Therefore, this research sought to determine salt dynamics in leached water from two irrigation systems in bulb onion production (Allium cepa L.) in the Samaca Valley - Boyaca.
MATERIALS AND METHODS
Location and sampling
This study was carried out in the Tibaquira village of the Valle Centro sector of the municipality of Samaca in Boyaca, at N 5° 31'53.98'' and W 73°29'15.7'' (Fig. 1), between the months of January and August, 2021. A mobile climate station was installed for climate monitoring.
Data capture and treatments
A water sample was taken from the farm reservoir (irrigation source), and a soil sample was taken before planting the crop for a chemical analysis in the exchangeable phase and soluble phase at the laboratory level. These data were used as an initial reference for the water quality and chemical properties of the soil (Tab. 1). The pH and EC values were 5.6-4.71 and 1.168-1.36 dS m-1 for the reservoir water and the exchangeable phase, respectively. The treatments corresponded to the two irrigation systems (Sprinkler and Drip): the sprinkler system had 9 m between emitters and 12 m between lines. The sprinkler capacity was 23.52 L min-1, which was a plastic Woobler type, 3/4 or 1/2. 5 self-compensating drip tapes were installed in each of the beds, with 10 cm between drippers and 40 cm between lines. Each dripper emitted 0.79 L h-1. The leached water samples for the chemical analysis were taken at three points in the crop cycle for both treatments: M1: 30 days after transplantation (dat), M2: 60 dat, and M3: 100 dat.
Table 1. Chemical parameters of irrigation water and soil.
Reservoir water (cmolc L-1) | Exchangeable phase (cmolc kg-1) | Soluble phase (mg kg-1) | |
---|---|---|---|
Ca2+ | 5.78 | 12.86 | 132.39 |
Mg2+ | 1.38 | 2.84 | 55.57 |
K+ | 0.3 | 1.08 | 54.46 |
Na+ | 2.56 | 0.94 | 11.14 |
SO4 2- | 7.22 | NA | NA |
Cl- | 3.96 | NA | NA |
HCO3 - | 0.5 | NA | NA |
NA: not applicable for determination.
The chemical characterization of the leached water to determine the electrical conductivity (EC), pH, sodium adsorption ratio (RAS), calcium (Ca2+), magnesium (Mg2+), potassium (K+), sodium (Na+), sulfates (SO4 2-), and chlorides (Cl-), was carried out in the soil and water laboratory of the Faculty of Agricultural Sciences of the UPTC. The techniques used a Hanna HI5521 for the pH and EC, an atomic absorption spectrometer for the bases Ca2+, Mg2+, K+, and Na+, titration with silver nitrate for chlorides, a thermoscientific genesis 10SVIS spectrophotometer for sulfates, and concentration of sodium compared to calcium and magnesium for the RAS. The classification diagram of the U.S Salinity Laboratory Staff 1960 was used as a reference to interpret the chemical quality, which establishes a relationship between the EC and RAS. The guides reported by Ayers and Westcot (1985) and Olías et al. (2004) were used to interpret the irrigation water.
Plant material and agronomic management
The Osaka F1 hybrid was used, which is a short-day hybrid adapted to cold and medium climates. It presents consistent bulbs, with excellent closure, a yellow color, globose shape, very fine, shiny cataphylls, and high tolerance to pink root (Pyrenochaeta terrestris). Its life cycle is 180 dat, which begins with the establishment of the seedbed, transplant at 60 d, and harvest at 120 - 140 dat. Pathogen control was guaranteed during the crop cycle and fertilization recommendations were made based on soil analysis. Edaphic fertilization was applied to both irrigation systems (Tab. 2). In the drip system, it was lower because the fertigation applications complemented its nutrition. During growth, the formula was, in mg kg-1, N:130, P2O5:50, K2O:90 and MgO:50; for maturation, it was N:120, P2O5:45, K2O:170 and MgO:45. The fertigation formulas were applied during growth (15 days after emergence, until day 60) and maturation (starting on day 61 until 20 days before harvest).
Statistical analysis
For the quantification of the response variables, three fiberglass lysimeters, 1×1×0.6 m, with a capacity of 0.35 m3, storage compartment, and outlet for water extraction, were placed in the field in each of the systems. The Sprinkler and Drip irrigation provided samples of leached water, obtained with suction probes (Irrometer hand vacuum pump), for a total of three sample units for each irrigation condition.
The data obtained from the response variables in the sample units were subjected to Box-plot analysis. Then, a hypothesis analysis was carried out by applying the t-student test for independent samples. These analyses were carried out with R version 4.0.1.
RESULTS AND DISCUSSION
Electrical conductivity and pH
The irrigation water had a pH between 5.6 and 6.01, within the optimal range according to Alarcón (2004) between 5.5 and 6.5, and an EC between 1.16 and 1.61 dS m-1, within the normal range of 0-3 dS m-1 proposed by Ayers and Westcot (1985). However, under the diagnostic classification of the SALSODIMAR model, it was identified as medium risk of salinization (Pla Sentís, 2014). Loera-Alvarado et al. (2019) found that water leached in reservoirs has a rapid neutralization of acid depositions, which generates pH between 7.5-8.3.
The analysis of the variables in the leached water showed that the EC had a similar behavior in the two irrigation systems, but statistical differences were found at 100 dat (P=0.0232). Walteros et al. (2018), under similar climate and soil conditions in the Alto Chicamocha irrigation district (Valle de Sugamuxi, Boyaca), reported an average EC of 6.34 dS m-1. This district has areas with acid sulfate soils, meaning that the chemical conditions of the water, soil and climate in the Samaca Valley can trigger chemical degradation and originate acid sulfate soils.
The leached water in the sprinkler irrigation had higher EC values, with an average of 3.54 dS m-1, reaching a higher value at 30 dat with 3.6 dS m-1. The drip irrigation had an average of 3.19 dS m-1; the highest value was at 30 dat with 3.92 dS m-1 (Fig. 2A), coinciding with the application of edaphic fertilization at the beginning of the crop in both irrigation systems and the beginning of fertigation. The values were within the normal ranges (0.5-5.0 dS m-1) according to Alarcón (2004), but, according to Castro et al. (2009), conductivities > 3.0 dS m-1 in irrigation water present risks of salinization problems.
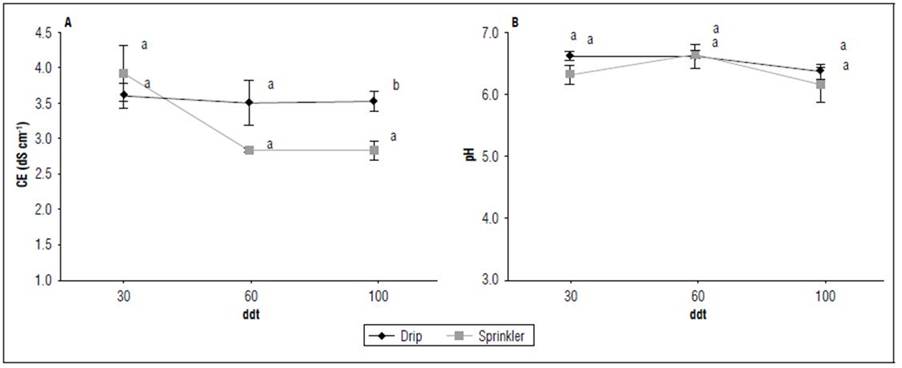
Figure 2. A. Electrical conductivity (EC). B. pH of the leached water for the two irrigation systems. Different letters at each sampling point indicate significant differences according to the t-student test (P≤0.05).
The EC had values > 3 dS m-1, which may be related to the presence of high contents of Na+ (0.94 cmolc), Ca2+ (12.86 cmolc) and K (1.08 cmolc) in the soil and the application of fertilizers in the form of salts that increased the osmotic pressure of its solution as a consequence of the concentration. Similarly, the irrigation water had an increase in EC, with an initial value of 1.16 dS m-1 and a final value of 1.61 dS m-1. Another important factor is soil, which was slightly saline according to Castro and Gómez (2010), presenting an EC ≥1.0 <1.5 dS m-1, a pH of 4.71 and a high sulfur value (479.65 mg kg-1), an initial condition for the formation of acid sulfate soils. Duncan et al. (2008) stated that the concentration of total salts dissolved in water directly affects the growth and quality of plants because of osmotic effects (reducing the availability of water for plants), with specific ion toxicities or nutritional imbalances.
During the crop cycle, precipitation was recorded with a climatic station installed in the plot (Fig 3). The months of March (128 mm) and May (105 mm) presented higher precipitation than the historical data of the IDEAM station (Villa del Carmen), with annual averages for March (80 mm) and May (78 mm). The variation for 2021 was, on average, 40% for these months. Therefore, in this study, the periods between March and April presented an average of 165.6 mm. At 30 dat, there was a precipitation of 73.6 mm; from 30 to 60 dat, it was 66.4 mm, which raised the water table and generated ponding, conditions favored by flat topography and high clay content (>60%), causing the salts to not be leached by the washing process but instead concentrate in the soil solution (Colombia IGAC, 2005).
According to Colombia IGAC (2005), the soil cartographic unit (VMA) in the Samaca valley has imperfect to very poor drainage and fine textures with frequent flooding. On the other hand, the period of low precipitation was from 60 to 100 dat, with 15.2 mm. These conditions favor the rise of salts through evapotranspiration processes. Delgado-González et al. (2022) reported that the cause of salinization processes can be multifactorial, occur naturally in arid and semi-arid areas, and are characterized by low rainfall and high evaporation rates. Another factor is the use of irrigation water with a high content of salts, inappropriate cultivation practices and excessive use of chemicals, among others.
The pH value for the leached water in the sprinkler irrigation system was 6.16 and, in the drip system, was 6.28 (Fig. 2B), appropriate for irrigation according to Alarcón (2004), who indicated that a pH between 5.5 and 6.5 is optimal. Likewise, Decree 1594 of 1984 for the use of water and liquid waste in Colombia states that the pH of water for agricultural use must be between 4.5 and 9.0 (Castro et al., 2009). The data were lower than those reported by Medina et al. (2016), who found a pH in irrigation water between 6.9 and 8.4. Mercado et al. (2011) cited high EC values in the La Doctrina Irrigation District (>3 dS m-1) and a pH in the range of 7-8, which showed the presence of highly soluble and mobile salts such as sodium chloride, contrary to the report by Walteros et al. (2018) for the upper Chicamocha irrigation district with an average pH of 3.12. Together, the EC and pH refer to the soil-plant relationship and the quality and availability of water and nutrients for plants (Romero et al., 2009).
Ionic concentrations
Figure 4A shows the behavior of the cation concentrations in the leached water for the two irrigation systems, with a similar trend but without significant statistical differences although, at 100 dat, the value was P=0.0693. The calcium (Ca2+) of the reservoir had a value of 5.78 cmolc L-1, normal and within the range of 0-20 cmolc L-1 (Ayers and Westcot, 1985). The Ca2+ values in the leached water increased by 34% during the three sampling periods (30, 60 and 100 dat), as compared to the reference value of the reservoir water. This increase can be related to the high content of Ca2+ in the soluble phase (169 mg kg-1) and in the exchangeable phase (12.86 cmolc kg-1) of the soil (Tab. 1) and to the content in the irrigation water.
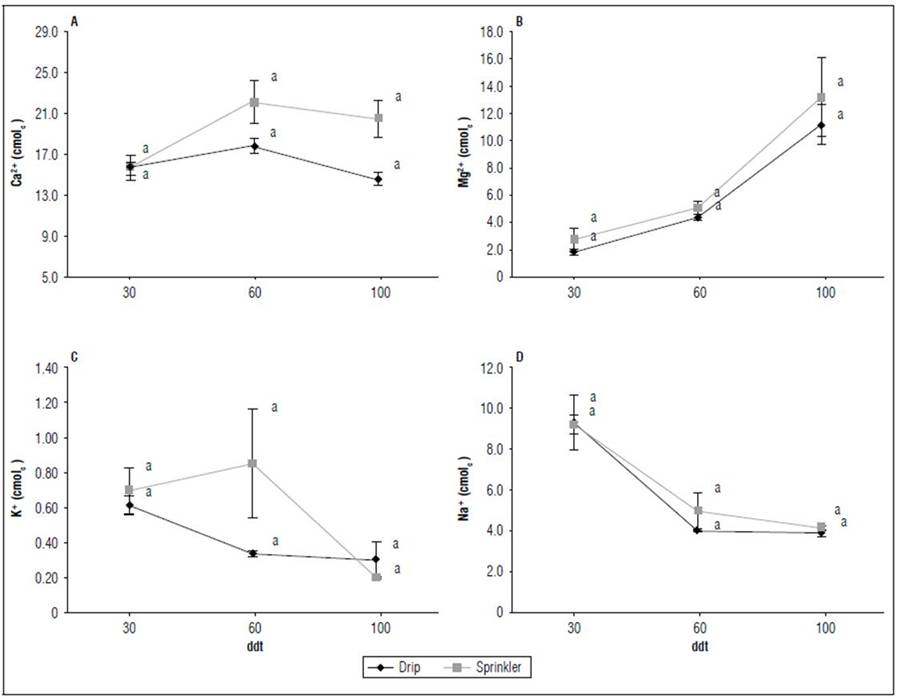
Figure 4. Concentration of cations present in the leached water of the irrigation systems. A, Ca2+ content; B, Mg2+ content; C, K+ content; D, Na+ content. Different letters at each sampling point indicate significant differences according to the t-student test (P≤0.05).
The leached water from the sprinkler irrigation system reached values of 15.58 to 22.10 cmolc, and the drip irrigation was from 15.69 to 17.82 cmolc, optimal and normal values according to (Olías et al., 2004) and Alarcón (2004), but high values compared to those reported by Castellón et al. (2015). Ca2+ is important in the stability of the physical properties of the soil and especially of the hydraulic conductivity; its availability is affected by solubilization and precipitation in the presence of bicarbonates (Can et al., 2008).
Magnesium (Mg2+) in water used for irrigation obtained a value of 1.38 cmolc, considered normal since it was between 0-5 cmolc (Olías et al., 2004). It showed a similar behavior in the leached water of the two irrigation systems during the development of the crop, with values between 1.82 and 13.20 cmolc (Fig. 4B) according to Alarcón (2004). Values up to 40 cmolc are normal and optimal between 4-10 cmolc. At 100 dat, a high trend was observed in the two irrigation systems with a concentration of 11.20 cmolc in drip and 13.20 cmolc in sprinkler, high values (> 5.0 cmolc) according to Olías et al. (2004). This can be related to the high content of this element in the exchangeable phase of the soil (Tab. 1), to lower precipitation (15.2 mm), higher evapotranspiration, and application of fertilizer during the crop cycle, conditions that facilitate the rise of salts.
Potassium had a value of 0.30 cmolc in the reservoir water, values of 0.20 to 0.85 cmolc in the sprinkler leached water, and 0.30 to 0.61 cmolc in the drip irrigation ( Fig. 4C), ranges that are considered normal by Alarcón (2004) since they were not >1 cmolc. There were no significant statistical differences at 60 dat (P≤0.1473) despite the fact that, in the sprinkler irrigation, the increase in concentration was 0.85 cmolc when compared to the drip irrigation with 0.34 cmolc. The increase of this element in the sprinkler irrigation was possibly due to the application of 50% of the fertilization at the beginning of bulbification, where the potassium based edaphic fertilizers leached at depth, while the fertigation in the control with bulb wetting did not reach depth.
The value of sodium (Na+) in the reservoir water was 2.56 cmolc in the leached water, the sprinkler irrigation obtained values of 4.14 to 9.21 cmolc, and the drip irrigation had values between 3.91 and 9.30 cmolc L-1 (Fig. 4D). According to Alarcón (2004) and Olías et al. (2004), these values were normal since they were within the range of 0-40 cmolc. This element should be kept as low as possible with adequate drainage systems. These values were high compared to those reported by Bonet and Ricardo (2011), who found between 3.9 and 4.8 cmolc in their research. El Gomez et al. (2010) stated that Na+ is a dominant cation that disperses clay and organic matter, leading to destruction of the structure. On the other hand, the hydrolysis of sodium clays leads to alkalinization of the profile, and this causes intense mineral alteration.
Nishanthiny et al. (2010) defined quality in agricultural water with the concentration of specific ions, Ca2+, Mg2+, Na2+ and K+ as cations and CO3 2-, HCO3 -, Cl- and SO4 2- as anions. These elements originate in the dissolution or weathering of rocks and soils, are transported by currents, both surface and underground, and are deposited in the soil naturally or through irrigation.
Sulfates showed significant differences at 100 dat (P≤0.059), obtaining values of 7.22 cmolc, considered normal in the reservoir water. In the leached water in the two irrigation systems, there was a similar behavior, with values between 1.83 and 1.94 cmolc at 30 dat and > 20 cmolc L-1 at 60 and 100 dat (Fig. 5A). The latter values were high and represented a risk of salinization (Ayers and Westcot, 1985) since normal ranges are between 0-20 cmolc. The increase in this ion was due to the high concentrations of sulfur (479.65 mg kg-1) in the exchangeable phase of the soil, the content in the irrigation water and the periods of less precipitation (60 to 100 dat) with 15.2 mm. The Sulfate levels were high compared to those reported by Castellón et al. (2015), with a range between 0.03 and 1.80 cmolc, and those reported by Herrera et al. (2018) in irrigation water in Chile.
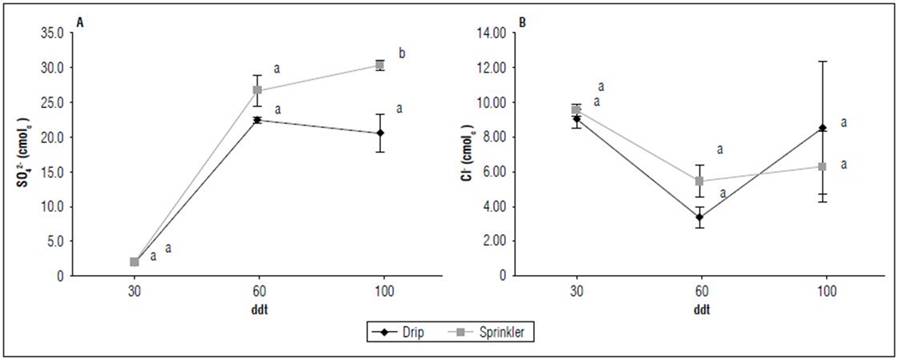
Figure 5. (A) SO4 2-and Cl- (B) concentration in leached water for both irrigation systems. Different letters at each sampling point indicate significant differences according to the t-student test (P≤0.05).
The chlorides (Fig. 5B) in the irrigation water were 3.96 cmolc, normal values. In turn, the leached water in the two irrigation systems had a similar behavior, with ranges between 3.36 and 9.52 cmolc, with a high tendency at 30 dat in the two irrigation systems and with values >8 cmolc in the drip irrigation at 100 dat. According to Olías et al. (2004) and Alarcón (2004), these values were normal since they were in the range of 0-30 cmolc. However, irrigation water should keep chlorides as low as possible. Bonet and Ricardo (2011) confirmed that high levels of Na2+ and Cl- in irrigation water are potentially toxic for crops.
In the study area, the ions that presented the highest concentrations in the irrigation water were SO4 2- and Mg2+, forming magnesium sulfate salts, MgSO4. These salts can be concentrated, mainly in the lower geomorphological zones, which are fed by runoff waters that reach the most depressed area and remain there with imperfect soil drainage, which contributes to increased salt concentrations. The most notable effects of the misuse of irrigation water are salinization and sodification of soils, which lose their structure and ability to support new agricultural cycles (García, 2015).
Anions modify soil properties directly by increasing salinity and indirectly by affecting the proportions of exchangeable sodium, calcium and magnesium (Medina et al., 2016). Gómez (2004) stated that salt dynamics cannot always be associated with the materials from which the soils come. Therefore, it is important to look for climatic, topographic, hydrological and anthropic factors. The salinity problem is generally related to the presence of different types of salts, whose degree of solubility from higher to lower is as follows: nitrates > chlorides > bicarbonates > sulfates > carbonates (Castro et al., 2009).
For Colombia IGAC (2005), the presence of these ions is directly related to the quality of water that reaches the ground, type of relief in the first level aggradational (depositional) terrace, flat topography, with slopes 1-3%, and frequent flooding. According to the Planning Development of the municipality of Samaca (Colombia Alcaldía Municipal de Samacá, 2020), the water that feeds the Valle district comes from two lake-type reservoirs, Gachaneca I and II, which store rainwater that falls directly on the surface and water collected by runoff from the Rabanal páramo. The Gachaneca I reservoir transfers to Gachaneca II, which is responsible for distributing to the system. The outlet of Gachaneca II is initially to the Gachaneca River and then passes through coal burners, which provide significant concentrations of sulfur that is released into the Ruchical and Pataguy channels. Guerrero-Dominguez et al. (2021) determined that the risk of salinization is conditioned by the ions dissolved in the water by the CEi (specific electrical conductivity), as well as by the contributions of organic matter from the environment, which can dissociate as weak acids with high CEi. This causes hydronium ions to be released. These variations in electrical conductivity and pH are dependent on the hydrosystems evaluated, rivers, dams, or streams, where intervention normally occurs through contributions of various materials of anthropic origin, affecting the quality of irrigation water.
Sodium adsorption ratio
The classification of the leached water in the two irrigation systems was done according to the relative concentration of Na+ with respect to Ca2+ and Mg2+ (RAS) (Fig. 6). The results for 100% of the analyzed samples were classified as C4-S1 according to the Riverside classification, indicating a very highly saline water (C4). It is not suitable for irrigation under ordinary conditions but can be used occasionally in very special circumstances. Soils must be permeable, with adequate drainage, water must be applied to achieve good washing, and crops that are highly tolerant to salts and low sodium (S1) water must be selected so that this water can be used for irrigation in soils with little probability of reaching dangerous levels of exchangeable sodium. Therefore, frequent monitoring of water sources used for irrigation and the EC of the soil must be carried out.
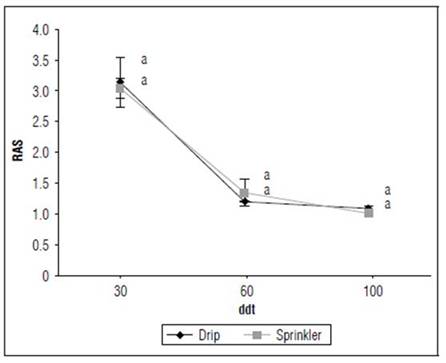
Figure 6. RAS in leached water from irrigation systems. Different letters at each sampling point indicate significant differences according to the t-student test (P≤0.05).
The RAS results did not present significant differences for the two irrigation systems (Fig. 6) and had higher values than those of Castellón et al. (2015), who reported RAS values between 0.60 and 1.18 in water used for agriculture in Mexico. Quinteros et al. (2019) studied the Togllahuayco River in Ecuador and reported RAS values of 3.94 cmolc L-1 and electrical conductivity of 324 µS cm-1, with a low saline hazard value and an average sodium hazard value, allowing sustainable water resource management.
In turn, the irrigation water (reservoir) was classified as C3-S1, highly saline water (C3), and cannot be used in soils where drainage is poor, even with adequate drainage; special control practices may be needed for salinity. Low Sodium Water (S1) can be used for irrigation in most soils with little chance of reaching dangerous levels of exchangeable sodium. According to the results, these waters can affect crop production and soils and contaminate aquifer areas.
Soil structural problems induce waterlogging once surface salts are leached, for example, by electrolyte-poor rainwater. Clay in the plow sole becomes a kind of permanent choke for the downward movement of water in saline-sodic soils, especially under irrigation with a high soil adsorption rate (RAS) or residual alkalinity waters (Minhas et al., 2019). The removed salts eventually find their way to the surface and groundwater resources, often degrading water resources used for agriculture downstream. A major problem related to salt leaching in arid areas, where large amounts of water are introduced, is the accumulation of salts in the groundwater (Minhas et al., 2020).
CONCLUSIONS
There is a high risk of salinization in the soils and water for the onion-producing area of Samaca because of the EC > 3.0 dS m-1 in the leached water and EC of 1.36 dS m-1 in the soil, along with the presence of sulfates, sodium and high concentrations of Mg2+. The leached water in the two irrigation systems was classified as C4-S1 according to the RAS and EC; the water had a very high risk of salinization. The irrigation water (reservoir) was classified as C3-S1, with a high risk of salinization.
The sprinkler irrigation system presented the highest values of concentrations in all variables because of the higher percentage of edaphic fertilization and a volume of water that facilitated leaching. In the drip irrigation, the fertigation application was done with less water, trying to manage the humidification bulb up to 20 cm. However, with the soil conditions with high clay and low permeability and the quality of the irrigation water, drip irrigation is not recommended since, over time, it can accelerate salinization processes.
The dominant ions in the leached water were sulfate and magnesium, which, when joined, form magnesium sulfate, which is dissolved and transported by the water table because of poor drainage that promotes its rise in winter.
Therefore, for the El Juncal farm in the Samaca valley, general monitoring of the irrigation reservoir should be done, and the quality of the water that recharges the reservoirs in the irrigation and drainage canals should be determined. Fertilization practices should be improved to optimize use and lower the quantity used, avoiding unnecessary recharging of ions in the soluble and exchangeable phases of the soil.