INTRODUCTION
Campomanesia lineatifolia R. & P., Myrtaceae family, known as champa or chamba, is a fruit tree grown in Colombia in areas with temperatures between 22 to 30ºC, with annual rainfall higher than 1,500 mm and in altitudes from 800 to 1,600 m a.s.l. The trees begin production at approximately 5 years and can be productive for more than 20 years (Villachica, 1996; Álvarez-Herrera et al., 2009a). The fruit are yellow berries at harvest, approximately 25 g, with a very good taste and aroma (Álvarez-Herrera et al., 2009a), which are consumed fresh or in derived products and are highly desired by consumers. C. lineatifolia has a high commercial potential, as seen in other myrtaceae such as arazá (Eugenia stipitata; Carrillo et al., 2011), gabiroba (Campomanesia adamantium; Do Santos et al., 2021), feijoa (Acca sellowiana; Fischer and Parra-Coronado, 2020; Fischer et al., 2020; Buitrago et al., 2021) and guava (Psidium guajava; Fischer and Melgarejo, 2021). It is being introduced in the different markets of Colombia (Balaguera-López and Herrera, 2012a; Porras et al., 2020). However, it has limitations and high perishability, which are responsible for high postharvest losses. Its shelf-life without postharvest treatments is 2 to 4 d (Álvarez-Herrera et al., 2009a, 2009b).
Some progress has been made in the post-harvest study of this species. In a preliminary study, it was found that weight loss increased with storage, as did total acidity, and soluble solids increased in the first days and then decreased slightly (Álvarez-Herrera et al., 2009b), while firmness decreased continuously to values close to 1 N. This parameter is one of the main indications of high perishability (Álvarez-Herrera et al., 2009a, 2009b). The ripening dynamics of champa fruit are closely related to the state of maturity at harvest, being slower in fruit harvested at an earlier stage of maturity (Balaguera-López and Herrera, 2012b).
In Myrtaceae, such as arazá, the short postharvest life is a problem that affects commercialization and can have different causes. The main ones are related to the high rate of respiration and ethylene production, high dehydration, dryness, rapid softening, and susceptibility to mechanical damage (Hernández et al., 2007; Carrillo et al., 2011). These causes could also be involved in the high perishability of champa fruit but they need to be studied in more detail. Recently, González et al. (2021) found that ethylene appears to regulate champa fruit ripening parameters such as respiration, firmness, color and acidity. Balaguera-López and Herrera (2012a) studied some biochemical changes during development and until harvest, such as respiration, ethylene production, polygalacturonase activity, organic acids, and soluble sugars. The present study aimed to report these parameters during postharvest, taking into account the importance of determining biochemical and physiological changes that occur in fruit during postharvest because these changes will be reflected in the physical and quality characteristics during marketing.
Therefore, the objective of this research was to study the behavior of different physicochemical aspects of champa fruit that determine the longevity and quality during postharvest, and that can explain its high perishability and industrialization alternatives and that are fundamental for the generation of effective conservation strategies.
MATERIALS AND METHODS
Plant material, localization, and storage
The champa fruits (C. lineatifolia R. & P.) were collected on the "El Mango" farm in the municipality of Miraflores (Boyaca, Colombia), located at 5º11'40” N and 73º08'44” W, at 1,432 m a.s.l., with precipitation between 2,000 and 3,000 mm, monomodal type, 22.3°C mean temperature and 80% relative humidity. The laboratory phase was carried out in the laboratories of Plant Physiology and Postharvest of the Faculty of Agricultural Sciences, in the laboratories of the Institute of Food Science and Technology (ICTA), in the laboratory of Tropical Fruit of the Faculty of Agricultural Sciences of the Universidad Nacional de Colombia in Bogota, and in the laboratory of the Amazon Institute for Scientific Research “Sinchi” in Bogota, Colombia.
Healthy fruit with a homogeneous size (23.4 g of fresh weight, and 36.3 mm of diameter approximately) were collected directly from trees with a yellow epidermis, which corresponds to consumption maturity, as reported by Balaguera-López and Herrera (2012b). Subsequently, the fruit were the fruits were packed and transported in plastic boxes. In laboratory fruit were taken and separated into four groups of 500 g. Each group was placed in polyethylene terephthalate packaging and corresponded to a repetition. Then, they were stored in chambers that allowed simulating the environmental conditions of the municipality of Miraflores as follows: average temperature of 22.3°C, relative humidity of 80%, and a photoperiod of 12 h light. Because this fruit is highly perishable, it was only possible to take measurements for 4 days after harvest (dah); these measurements were taken daily as described below.
Quantification of respiration rate, ethylene production, color index, firmness, and polygalacturonase activity
The respiratory rate and ethylene production were measured at four repetitions of approximately 50 g using gas chromatography, according to the methodology described by Hernández et al. (2007). Weight loss was estimated from the difference in fresh mass of the fruit measured on a digital scale with a sample of approximately 100 g of fruit (González et al., 2021). The epidermis color was determined with a digital colorimeter (Minolta CR-400); the parameters of the CIELab system "L*", "a*" and "b*" were determined for one fruit per repetition. L* coordinate represented the brightness, with values between 0 (totally black) and 100 (totally white). The coordinate a* assumed negative values to green and positive values to red, the b* coordinate with blue (negative values) to yellow intensity (positive values) (Abreu et al., 2020). Three readings were taken on the equatorial diameter of each fruit. The data were used to determine the color index reported by González et al. (2021). The firmness of the fruit (N) was measured in the equatorial part of two fruit in repetition with a digital penetrometer PCE-PTR200 with an approximation of 0.05 N. The activity of polygalacturonase was determined according to Espinal (2010) and expressed in nmol of galacturonic acid per mg of protein, per second (nmol PGA/mg of protein - s).
Quantification of soluble sugars, organic acids, soluble solids, total acidity, and pH
The content of soluble sugars (sucrose, fructose, and glucose) and organic acids (citric, malic, oxalic, and succinic) was quantified with high-performance liquid chromatography (HPLC) according to ICTA (2006) and Ávila et al. (2007); these analyses were done in triplicate. The soluble solids (SS, %) were quantified with a Hanna brand digital refractometer ranging from 0 to 85%; the total acidity (TA; % citric acid) was determined with an acid-base titration from 0.1 N NaOH with digital buret. The pH was obtained with 5 mL of champa juice, and it was measured with a potentiometer previously calibrated with buffer solutions of pH 7.0 and 4.0.
RESULTS AND DISCUSSION
Respiration rate and ethylene production
The respiratory rate of the champa fruit had a peak on the second day after harvest with a production of 106.43±5.16 mg CO2 kg-1 h-1, later, the respiration of the fruit decreased 89.99±5.01 mg CO2 kg-1 h-1. On day two after harvest, there was also an increase in ethylene production with 10.98±1.23 μL C2H4 kg-1 h-1, and the ethylene emission decreased in the following days (Fig. 1). This confirmed that champa fruit have a typical pattern that has been described for climacteric fruit (Saltveit, 2019; Botton et al., 2019). The observed climacteric point agrees with that reported by Álvarez-Herrera et al. (2009b) for this same species, determined using the respirometer method with a considerably lower respiratory rate. A discrepancy between two methods of measuring respiration was found in cape gooseberry (Physalis peruviana L.) fruit, where Villamizar et al. (1993) indicated that this fruit was non-climacteric with the volumetric method, while Novoa et al. (2006) determined that the cape gooseberry belongs to the group of climacteric fruit with gas chromatography, since this method is much more accurate.
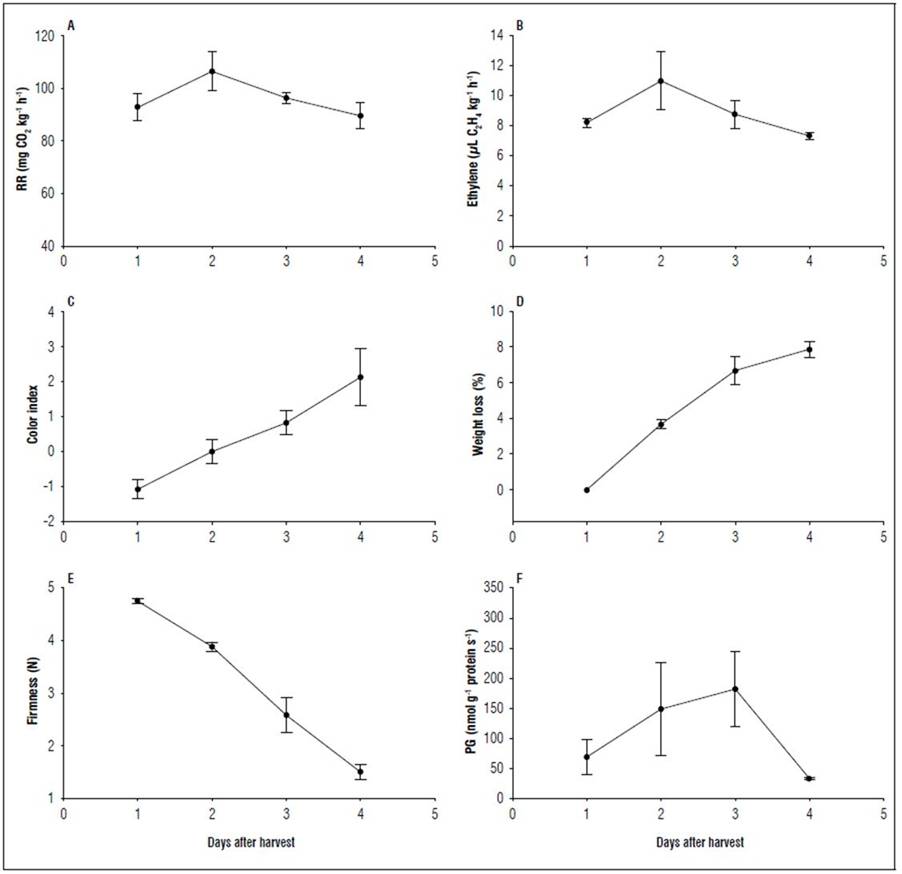
Figure 1. Respiration rate (RR) (A), ethylene production (B), epidermis color index (C), weight loss (D), firmness (E) and poligalaturonase activity (F) during postharvest of the champa fruit. The vertical bars indicate standard error (n=4).
Climacteric respiration is also present in other species of the Myrtaceae family, such as feijoa, guava, and arazá. However, the respiration of the champa fruit was higher than that reported in gabiroba (Do Santos et al., 2021) and lower than that of guava and arazá (Mercado-Silva et al., 1998; Hernández et al., 2007). The champa fruit has high perishability, similar to the arazá fruit (Hernández et al., 2007; Carrillo et al., 2011). These same authors stated that the short postharvest life of arazá is due to the high rate of respiration and ethylene production (determined by gas chromatography), severe dehydration, softening and susceptibility to mechanical damage, low dry matter content, and absence of support tissues (Hernández et al., 2007; Carrillo et al., 2011), which would also explain the short postharvest life of champa fruit, together with a very thin epidermis that does not offer enough protection but rather a greater permeability for gases and water vapor.
According to ethylene production, champa fruit can be classified as high producer of this hormone (Kader, 2002). Ethylene is synthesized from the amino acid methionine by the action of S-adenosyl methionine synthase, ACC (1-Aminocyclopropane-1-carboxylic acid)-synthase and ACC-oxidase. Then ethylene binds to a membrane receptor to trigger processes related to fruit ripening (Dubois et al., 2018). González et al. (2021) found that ethylene regulates several aspects of ripening in champa fruit, such as respiratory rate, epidermis color, and firmness. But, it can also be responsible for its high perishability. Hernández et al. (2007) observed higher ethylene values for arazá during postharvest than in champa fruit.
Weight loss and epidermis color index
Weight loss increased continuously during storage; each day, the fruit lost 2.66% of its weight. At the end, the loss reached 7.88±0.45% (Fig. 1). This loss rate was slightly higher than that found by Álvarez-Herrera et al. (2009b)), and similar to that observed in feijoa (Castellanos et al., 2016a). Since weight losses greater than 5% causes quality deterioration in most agricultural products (Díaz-Pérez, 2019), the champa fruit must be marketed no later than 2 dah or subject to postharvest treatments that maintain quality for longer (González et al., 2021). Weight loss in champa fruit during postharvest is due to the loss of substrates, caused by respiration and water loss through transpiration (Lufu et al., 2019). This last process causes oxidative damage through desiccation and, further, antioxidant constituents are lost, generating quality losses (Lufu et al., 2019).
The color index increased during postharvest from -1.07±0.27 on day 1 to 2.12±0.80 on day 4 (Fig. 1). This increase was also corroborated by González et al. (2021). This color change is similar to that of guava fruit, explained by the degradation of chlorophylls through chlorophyllase enzymes, and also by the increase in carotenoid synthesis (Kumari et al., 2020), which favor the characteristic yellow color of champa (Álvarez-Herrera et al., 2009a). This last factor is the most determining because the fruit showed an intense yellow color at harvest; therefore, it was presumed that the chlorophyll content was very low. It has been reported that color change is a process associated with the presence of ethylene in this species (González et al., 2021).
Firmness and polygalacturonase activity
A rapid loss of firmness in the champa fruit was observed as the postharvest time elapsed; on day 4, the fruit presented a firmness of 1.5±0.14 N (Fig. 1). This value is somewhat higher than the one reported for this same species by Álvarez-Herrera et al. (2009b) with 1 N on 4 dah. The load that fruit can withstand is equivalent to 70% of its resistance (Márquez et al., 2007), indicating that champa fruit cannot be practically packed in bulk in boxes. Much higher firmness values were found in feijoa fruit (Castellanos et al., 2016b) and in arazá (Hernández et al., 2007; Carrillo et al., 2011). Rapid softening during ripening is one of the main causes of the short postharvest life of fruit; therefore, any reduction in softening can be of significant commercial importance. González et al. (2021) significantly decreased the loss of firmness by applying 1-MCP and cooling at 2ºC.
Álvarez-Herrera et al. (2009a, 2009b) showed that low firmness in champa fruit during ripening and postharvest is an indication of their high perishability and is one of the main limitations of handling during this stage, which limits commercialization as fresh and transformation into pulp for derived products. Alós et al. (2019) stated that oversoftening produces important economic losses since it reduces transportability, storage time, and postharvest shelf-life.
The reduction in firmness observed is mainly a consequence of modifications in the carbohydrate metabolism of the cell wall and its structure. So, the rate of degradation of pectic substances is directly correlated with the rate of softening of fruit (Wills and Golding, 2016). In many cases, the changes observed in firmness during ripening are mainly due to the hydrolysis of pectin by the action of PG (Pareek, 2016). Also, alterations in the turgor of the cell and degradation of starch reserves lead to loss of firmness in fruit (Pareek, 2016). Álvarez-Herrera et al. (2009b) found a significant and inverse correlation between weight loss and firmness.
An increase in polygalacturonase activity was observed from the first day after harvest to day 3; on this day, it presented the highest value with 181.92±62.92 nmol PG g-1 protein s-1 or PGU (PG units). For the fourth day, the activity decreased (Fig. 1). The PG activity found in the champa fruit agrees with that reported for guava (Espinal, 2010). This author found values of PG activity higher than those registered in this study, with values that ranged between 100 and 500 PGU.
PG catalyzes the hydrolytic cleavage of galacturonide bonds in the pectin matrix. The substrates for PG in cell walls are mainly desesterified homogalacturonans; therefore, the action of PG requires pectins previously desmethyl esterified with the PME enzyme (Pareek, 2016; Sañudo-Barajas et al., 2019). The end result of the action of PG is the loss of cellular integrity and an increase in the concentration of soluble polysaccharides. Thus, PG and PME affect the integrity of the middle lamella of cell walls, which controls cell-to-cell adhesion, which is why PG is involved in fruit softening (Pareek, 2016), including champa fruit. This enzyme may be responsible for rapid softening.
In guava, PgPG1 presented its highest expression in the mature stage of the fruit (Reyes et al., 2013). In this species, the behavior of PG in ripening and its relationship with softening has also been reported (Abu-Goukh and Bashir, 2003). It was observed that the highest PG activity coincided with the maximum climacteric. In addition, it has been reported that ethylene has a certain influence on the activity of this enzyme (Botton et al., 2019). Mondal et al. (2008) observed that, in guava, the climacteric generated a loss of firmness through activation of the PG and PME enzymes by ethylene.
Soluble sugars and soluble solids
The predominant sugar was sucrose, followed by fructose, and finally glucose. Sucrose presented a high value (61.42±11.6 mg g-1 of fresh weight, FW) on 1 dah. This value decreased for day 2, remained stable on day 3, and then increased slightly for day 4, reaching a value of 44.06±3.67 mg g-1 FW (Fig. 2). Glucose and fructose had similar behaviors: on day 1, the values were 8.89±0.92 mg g-1 FW and 18.15±3.12 mg g-1 FW, respectively; then, they increased in a representative way for day 2, stabilized on day 3, and increased until day 4, with the highest concentration, 24.75±0.71 mg g-1 FW for glucose and 42.22±0.96 mg g-1 FW for fructose (Fig. 2). These results differed from those found in fruit of other species of Myrtaceae, such as arazá (Hernández et al., 2007) and feijoa (Parra-Coronado et al., 2022; Rodríguez et al., 2006), in which the predominant sugar is fructose.
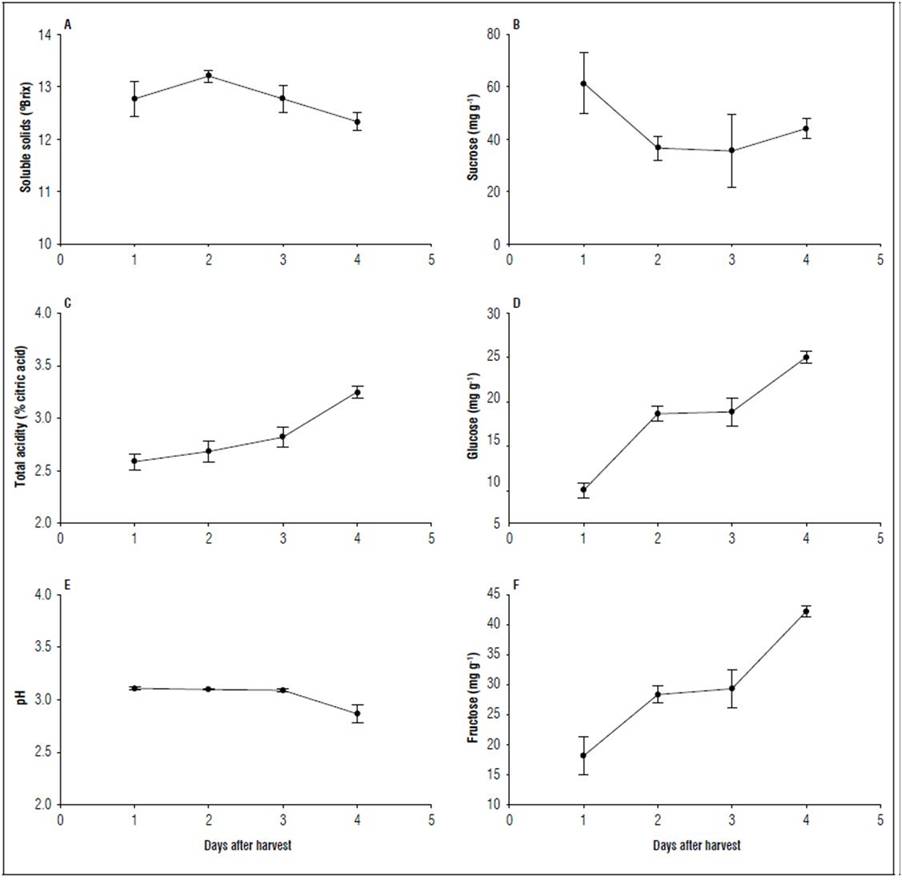
Figure 2. Soluble solids (A), total acidity (C), pH (E) and soluble sugars (B, D, F) during postharvest of the champa fruit. The vertical bars indicate standard error (n=4), in soluble sugars (n=3).
High levels of sucrose at harvest in champa fruit may be due to the metabolism of structural and reserve polysaccharides at this stage which is favorable from the point of view that this disaccharide stores carbohydrates more efficiently than monosaccharides (Fischer et al., 2015), a process catalyzed by mainly hydrolytic enzymes, such as amylases and glucosidases (Pareek, 2016). But, it can also be due to importation from leaves in the final stage of fruit growth (Fischer et al., 2015), which is directly related to the increase in the activity of sucrose phosphate synthase. For their part, the increases in glucose and fructose as a function of the maturation process could be explained by greater activity of the acid invertase enzyme, whose substrate is sucrose (Vallarino and Osorio, 2019).
The soluble solids (SS) showed a slight increase from day 1 to 2, when they reached the maximum value (13.2±0.11%), followed by a not very representative decrease until day 4 (Fig. 2). SS play an important role in improving fruit quality and gives a rough idea of the sweetness (Kumari et al., 2020). Thus, the values indicated that the champa fruit had a significant content of SS, which is considered a determining factor for the pleasant flavor of this fruit, although it was higher than that reported for C. rufa (Abreu et al., 2020). Close but lower values were reported by Kumari et al. (2020) for various guava cultivars. From harvest to climacteric, the content of SS increased, possibly because of the hydrolysis of various structural polysaccharides such as starch, pectins, and other oligosaccharides of the cell wall, to their basic monomeric components, which, when solubilized in the aqueous phase, began to form part of the juice (Menéndez et al., 2006). In the case of the champa fruit, it was explained by increases mainly in glucose and fructose but also by increases in total acidity (Fig. 2).
After climacteric, SS decreased possibly because sucrose was used as a respiratory substrate in the fruit. Rodríguez et al. (2006) reported an increase in SS in feijoa until one day after the climacteric and then a decrease that may be associated with high respiratory rates. In overripe guava, SS also decreased (Mercado-Silva et al., 1998). The decrease in SS in champa fruit at the end of postharvest may be associated with the loss of flavor.
Organic acids, total acidity, and pH
The predominant acid during postharvest was citric acid, which presented a continuous rise as storage increased. The concentration was 14.04±0.68 mg g-1 of fruit 1 dah, and the maximum value found on 4 dah was 22.33±0.35 mg g-1 of fruit. For its part, oxalic acid increased considerably from 1 dah (0.74±0.03 mg g-1 FW) to 2 dah (3.45±0.45 mg g-1 of fruit) and then drastically decreased on 3 dah (0.013±0.003 mg g-1 of fruit). On 4 dah, it was no longer detected. For malic acid, it was detected only on 3 and 4 dah, changing from 12.13±0.368 mg g-1 to 3.5±0.189 mg g-1 of fruit, respectively. Finally, succinic acid had the highest concentration on 1 dah (2.96±0.54 mg g-1 of fruit). On 2 dah, there was no presence of this acid but then it was identified again on day 3 and increased until the fourth day (Fig. 3).
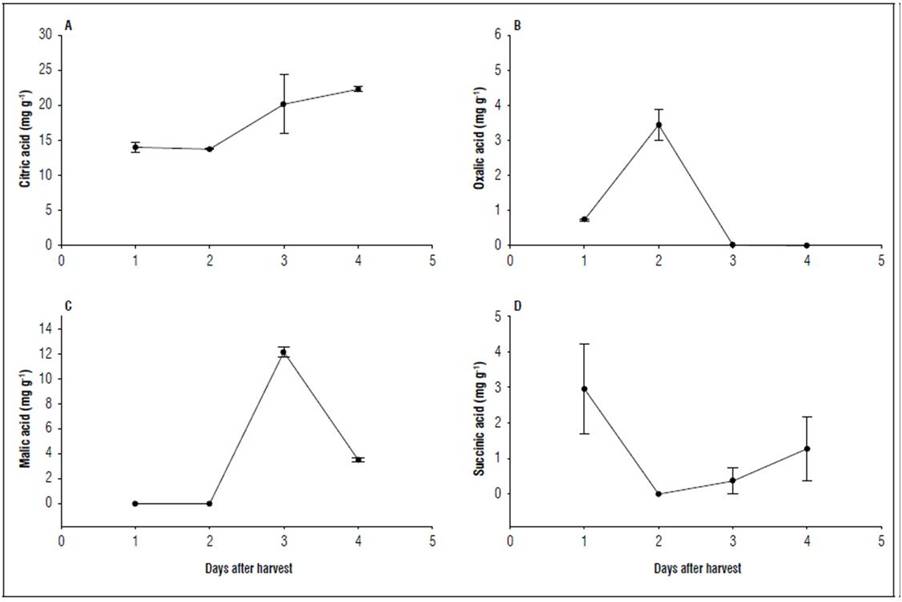
Figure 3. Organic acids during postharvest of the champa fruit. The vertical bars indicate standard error (n=3).
As found for the champa, in guava, the predominant acid is also citric (Mercado-Silva et al., 1998), as in C. pubescens (Silva et al., 2009), but it differs from arazá, which has the predominant malic acid (Hernández et al., 2007; Carrillo et al., 2011).
The content of organic acids was determined by the balance of synthesis and degradation (Tang et al., 2010). The immediate precursors of organic acids are other organic acids and sugars. It is possible that the synthesis of citric acid, from 2 dah was given from oxalic acid, and, from 3 dah, it depended on malic acid. This would indicate that, in champa fruit, the decrease in malic acid and the increase in citric acid may be due to an increase in the malic enzyme. In Eriobotrya japonica fruit, Chen et al. (2009) found that this enzyme plays an important role in reducing malic acid. Citric acid is synthesized by the condensation of oxaloacetic acid by the enzyme citrate synthase; this enzyme catalyzes the first step in the citric acid cycle; however, the enzyme PEP carboxylase can also play an important role in the biosynthesis of this acid (Etienne et al., 2013; Vallarino and Osorio, 2019).
Malic acid is one of the most widespread acids in nature, being the predominant one in a large number of vegetables and fruit. It is a weak acid and not very resistant to oxidative respiration (Rizzon and Sganzerla, 2007), and this may in part explain the low or no concentration of this acid in the postharvest of the champa fruit. In this stage, the degradation of malic acid is more important than synthesis, which determines the reduction of the content of this acid, which is also faster the higher the temperature is (Rizzon and Sganzerla, 2007). In Eriobotrya japonica fruit, the malic acid concentration decreased linearly with an increase in the activity of the malic enzyme (NADP-ME) and was directly proportional to the enzyme NAD-malate dehydrogenase (NAD-MDH) (Chen et al., 2009). It is possible that, in champa fruit, these enzymes regulate the synthesis and degradation of malic acid. On the other hand, in fruit, a part of the malic acid can come from the oxidation of glucose in respiration (Etienne et al., 2013).
During the postharvest of arazá fruit, it was found that malic and citric acid decreased slightly, while succinic acid increased (Carrillo et al., 2011), which partially coincides with that found in the champa fruit in this study, Hernández et al. (2007) also found a decrease in malic acid in arazá, as did Castellanos et al. (2016b) for feijoa fruit. The increase in the acidity of the champa fruit was largely dependent on the increase in citric acid and is a process that has been reported in other Myrtaceae, such as feijoa (Parra-Coronado et al., 2022; 2015). For oxalic acid, the champa fruit presented contents higher than those found in the cape gooseberry. Apparently, in this fruit, this acid increases during storage (Novoa et al., 2006). The succinic acid content in general, decreased in champa fruit, which agrees with that reported for arazá (Hernández et al., 2007). The absence of succinic acid on day 2 after harvest could be because that this acid can be rapidly synthesized and degraded to other acids of the Krebs cycle. So, in pomelo fruit, a strong negative correlation was found between succinic acid and acid citric, and positive correlation with malic acid (Pan et al., 2021).
Organic acids in champa fruit, as in other fruit, can have various functions: they can participate directly in aerobic and anaerobic respiration in the tricarboxylic acid cycle, in many fruit, they form the second most important energy deposit (after carbohydrates), and are the base and intermediate of the products for synthesis in the primary and secondary metabolism of fruit (Saltveit, 2019). Additionally, organic acids provide hydrogen ions that modify pH, where a higher acid content means a lower pH.
During postharvest, the TA of the champa fruit increased. On day 4, it presented the maximum value with 3.25±0.05% (Fig. 2). This result agrees with that reported by Álvarez-Herrera et al. (2009b) for the same fruit. Organic acids are important substrates for fruit respiration; several of these acids are essential components in the respiratory cycle of tricarboxylic acids (Pareek, 2016). Therefore, it would be expected that TA would decrease during the postharvest of champa fruit. However, the observed behavior could be associated with increases in citric acid, as previously reported, but also with the reserves of starch and polysaccharides in champa fruit, which would be sufficient to cover most of the energy requirements of the plants. Reactions that occur in the climacteric and could also give rise to organic acids (Saltveit, 2019; Wills and Golding, 2016). This added to a possible increase in pectic acids from the degradation of cell walls (Wills and Golding, 2016), which could contribute to the increase in TA observed during climacteric. On the contrary, in feijoa, TA decreased during postharvest (Rodríguez et al., 2006; Parra-Coronado et al., 2018). Apart from their biochemical importance, organic acids contribute largely to flavor, in a typical relationship between sugars and acids in different species of fruit trees (Wills and Golding, 2016).
Hernández (2001) stated that the fruit of some Myrtaceae with a climacteric respiratory pattern rapidly unfold their reserves (organic acids) during the respiratory maximum in response to the increase in metabolism, with increases in SS and TA. However, in champa, there was only evidence of an increase in AT with a decrease in SS after day 2. This could indicate that the acids present in champa fruit may originate from sugars through the process of glycolysis and the Krebs cycle (Etienne et al., 2013).
In the first 3 days, the pH remained almost constant but, on day 4, the lowest value was 2.87±0.05 (Fig. 2). According to Rodríguez et al. (2006), the pH value is important when fruit is being industrialized because it is related to the transformation costs of the product. The pH value for the champa fruit was lower than those reported for C. pubescens by Silva et al. (2013). It is important to mention that, when acidity is higher, there is a greater possibility of the industrialization of the fruit in the form of sweets. One of the most common uses of champa fruit is the production of homemade juices; however, Balaguera-Lópezet al. (2009) stated that this use is due to its exquisite flavor but not to its efficiency in the yield of the pulp. However, the results in figure 2 confirm that, industrially, the champa would also have great acceptance because of its high acidity.
During the postharvest of feijoa, Rodríguez et al. (2006) found pH values higher than 2 but lower than 3.5, with a tendency to increase in the final stage of storage, like the trend reported for C. pubescens by (Silva et al., 2013), but contrary to that observed in the champa fruit.
CONCLUSION
Champa fruit, according to the respiration pattern, presents a typical behavior of climacteric fruit, with a respiratory peak of 2 days after harvest, accompanied by an increase in ethylene production on the same day, reaching levels that classify them as high producers of ethylene. The polygalacturonase activity increased until the third day and subsequently decreased. The activity of this enzyme may be related to the loss of firmness. A high rate of weight loss and a continuous increase in the color index were also observed. The biochemical changes had some atypical behaviors. SS and pH increased until climacteric and then decreased; TA increased considerably. Citric acid was the predominant acid and rose continuously during storage; succinic acid also increased, while oxalic and malic acids decreased. The content of organic acids in the fruit was high. Sucrose was the predominant sugar, but it decreased with maturation, followed by fructose and glucose, sugars that increased in the postharvest content.