INTRODUCTION
Rice production worldwide has been severely affected by bacterial panicle blight (BPB), caused by the β Proteobacterium Burkholderia glumae (Kurita and Tabei) Urakami et al. BPB was reported for the first time in Japan in 1956 and was initially named bacterial rice grain rot. BPB was later found in rice-growing countries on continents such as Asia, Africa, and South and North America, including some US states (Ortega and Rojas, 2021).B. glumae attacks rice in two different stages of plant development, causing several symptoms, such as seedling rot during early stages of the growth cycle. Later, in the reproductive phase, symptoms, which give rise to the name bacterial panicle blight, include panicle sterility, grain rot, weight reduction and grain abortion. The BPB disease causes losses ranging from 10 to 75% depending on the cultivar (Pedraza et al., 2018).
However, not many alternatives are available for controlling this disease. Chemical control methods are not available although oxolinic acid is used as the principal BPB control in countries such as Japan (Xin-Gen, 2019). BPB control strategies include: exclusion, defined as any measure that prevents the introduction of a disease-causing pathogen into a region, farm or crop although this measure usually only delays the entry of a pathogen (Maloy and Baudoin, 2001); genetic resistance, however, no genes or QTLs associated with BPB resistance have been reported; chemical control but few chemical compounds have been described for controlling BPB apart from the commercial product Starner® based on oxolinic acid, however, B. glumae populations resistant to oxolinic acid have been reported since 1998; biological control with the use of a Pseudomonas sp. Strain and bacteriophages as antagonists or bacterial biocontrol for BPB, resulting in reduced BPB severity; and, finally, cultural practices but there are few studies on this aspect (Xin-Gen, 2019).
Burkholderia glumae is a Gram-negative seed-borne and rod-shaped bacterium. This bacterium grows in environments with high temperatures and humidity. Toxoflavin [1,6-dimethylpyrimidio (5,4-e)-1,2,4-triazine-5,7 (1H,6H)-dione] is its major virulence factor (Cho et al., 2007). This genus is widely distributed, covering various ecological niches, and two large phylogenetic groups are distinguished.This bacterium is a member of a phytopathogenic subgroup, and all identified strains of B. glumae cause panicle blight in rice, making it a growing problem around the world (Voget et al., 2015). In spite of its economic significance, neither effective control measures for this disease nor resistant rice varieties are currently available (Zhou-Qi et al., 2016). BPB has become a threat to rice cultivation in different regions of the planet, Coupled with climate change, this disease can become more prevalent, with greater economic damage in rice growing regions (Shew et al., 2019).
The use of nanotechnology-based tools has become a promising research area with different applications in the agricultural field, with a special emphasis on disease control in crop plants (Ahmed et al., 2021). Silver nanoparticles (AgNPs) have unique characteristics and stand out among other noble metals, including antibacterial and antifungal properties, in industrial, household, sanitary, cosmetic and orthopedic products to name a few (Zhang et al., 2016). AgNPs have been used against phytopathogens such as Ralstonia solanacearum (Smith) Yabuuchi et al. emend. Safni et al. (Hai-Jun et al., 2020; Khan et al., 2021), Pseudomonas rhodesiae Coroler, Bacillus licheniformis (Weigmann) Chester,Bacillus thuringensis Berliner, Streptomyces griseus (Krainsky) Waksman and Henrici, Achromobactersp. (Ali et al., 2020), plant pathogenic fungi such as Alternaria alternata (Fries) Keissler, Alternaria brassicicola (Schweinitz) Wiltshire, Alternaria solani Sorauer, Cylindrocarpon destructans (Zinssmeister) Rossman, Lombard and Crous, Fusarium solani (Martius) Lombard and Crous,and Monosporascus cannonballus Pollack and Uecker among others (Kim et al., 2012), and human pathogenic bacteria. They have become one of the most widely used nanomaterials in consumer products. AgNPs have shown efficiency in controlling phytopathogens and have been widely studied and tested in agricultural research with the aim of improving the production, efficiency and sustainability of agricultural crops (Gupta et al., 2018). AgNPs are synthesized by physical, chemical and biological methods, with electrochemical synthesis being the most popular and economical (Padilla-Sierra et al., 2021).
AgNPs provide a low-toxicity, low-spectrum biocide effect, with a low probability of developing microbial resistance, and are effective against both Gram-positive and Gram-negative bacteria, affecting their growth (Wang et al., 2017). AgNPs trigger an antibacterial mechanism by facilitating the entry of AgNPs into cells, followed by an explosive release of silver ions that cause a bactericidal effect (Avila-Quezada and Espino-Solis, 2020). The antibacterial activity of AgNPs is known to be shape, size, charge, and dose-dependent (Liao et al., 2019). Smaller nanoparticles enhance their stability and biocompatibility, requiring an appropriate size and shape design for a wider range of use. Compared to traditional antibiotics, AgNPs have antibacterial properties through different mechanisms, with the most prominent antimicrobial modes of action being adhesion to microbial cells, penetration into the cell, generation of free radicals and ROS and modulation of microbial signal transduction pathways (Dakal et al., 2016). Other researchers have proposed that AgNPs attach to the surface of bacteria, altering the properties of the membrane and causing dissolution, while DNA damage occurs inside the bacteria (Mikhailova, 2020).
This study aimed to determine the antibacterial effect of electrochemically synthesized AgNPs against the bacterium B. glumae, which causes bacterial panicle blight (BPB) in rice (Oriza sativa L.). Currently, there is no control method, and the study of new control methods is required. Better BPB management is needed to reduce the damage and economic losses caused by this disease and to obtain higher yields.
MATERIALS AND METHODS
Electrochemical synthesis of AgNPs
AgNPs were synthesized according to Khaydarov et al. (2009), with minor modifications (Khaydarov et al., 2009). Two high-purity silver cylindrical rods (Aldrich® 99.99%), 10 cm in length and 2 mm in diameter, were used as electrodes, which were separated by 2 cm and coupled to a DC power supply (UNI-T® model UTP3315FL) with a potential difference of 24 V. 225 mL of distilled water were used as the electrolyte. The total time for the synthesis was 1 h at room temperature. The concentration of AgNPs in the electrolyte was determined by measuring the total dissolved solids (TDS) with a SI-Analytics® HandyLab 680 FK multiparameter. The wavelength of maximum absorbance was determined using UV-VIS spectrometry (Genesys 10S-Thermo Scientific®), which was used to make the calibration curve of the concentration of the AgNPs and the absorbance according to the Beer-Lambert's Law (Swinehart). This wavelength corresponded to the surface plasmons resonance of the AgNPs. The morphology, size and structure of the AgNPs were analyzed with transmission electron microscopy (TEM-FEI Tecnai G2 F20) and ImageJ software (Schneider et al., 2012).
Bacterial culture
The growth inhibition tests of B. glumae by AgNPs were carried out with the certified strain ATCC 33617, which was kindly provided by the Federation Nacional de Arroceros (FEDEARROZ, abbreviation in Spanish). B. glumae was grown in King B medium and selective Tsushima's S-PG medium.
Minimum inhibitory concentration (MIC) and minimum bactericidal concentration (MBC)
For the MIC, nutrient broth dilutions were done in triplicate in 10 different tubes from a stock solution of 20 mg L-1 AgNPs, each tube containing 1 mL of AgNP solution with concentrations of 1, 2, 3, 4, 5, 6, 7, 8, 9 and 10 mg L-1, 1 mL nutrient broth and 100 uL of bacterial suspension with a 24-h incubation. At 625 nm and an absorbance between 0.08-0.1, the initial inoculum concentration was calculated at 1.5·108 bacteria. A nanoparticle-free or bactericidal tube was used as a negative control, and a tube with bacterial suspension and oxolinic acid was used as a positive control according to the manufacturer instructions (1 g L-1). The minimum inhibitory concentration was determined as the concentration of silver nanoparticles contained in the tube that inhibited the visible growth of bacteria.
To determine the MBC, 10 uL were taken from the tubes without visible growth of bacteria and inoculated into Petri dishes with King B agar and different concentrations of AgNPs. The analyses were carried out in triplicate. The concentration that showed no bacterial growth was considered the MBC.
Determination of percentage growth inhibition
B. glumae growth was detected in triplicate with optical density (OD) (Spectrophotometer genesis 10S, Thermo) at 625 nm, and the percentage bacterial growth inhibition was calculated with the formula: Percentage growth inhibition = (OD of control/(OD control - OD control of test))×100 (Banjara et al., 2012).
Statistical analysis
The assumption of normality was analyzed with the Shapiro-Wilk test, and the homogeneity of variances was determined with the Levene test. The absorbance data, a parameter for measuring bacterial growth, met these two assumptions. An analysis of variance (ANOVA) and comparison of means according to Duncan were performed. The analyses were done with 95% confidence (P-value 0.05) using the infoStat software, version 2017. The treatments consisted of ten concentrations of silver nanoparticles (1 -10 mg L-1 AgNPs), a negative control and a positive control (oxolinic acid).
RESULTS
Silver nanoparticles synthesis
The growth behavior of the AgNPs as a function of time is shown in figure 1, which shows the best linear adjustment (R=0.997) to the experiment data, obtaining a slope with a value of 0.99749 mg L-1-min, representing the synthesis speed of the AgNPs in this electrolytic.
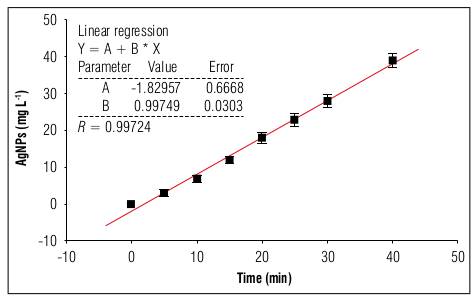
Figure 1. Concentration of AgNPs as a function of time. The line represents the best linear fit to the experiment data.
In 1 h of synthesis, 58±4 mg L-1 of AgNPs were obtained in the aqueous solution. The UV-Vis absorption spectrum for AgNPs at 40 mg L-1 is in figure 2, showing a value of λ=410 nm of maximum absorbance. This value corresponds to the surface plasmon resonance wavelength of the AgNPs at this concentration.
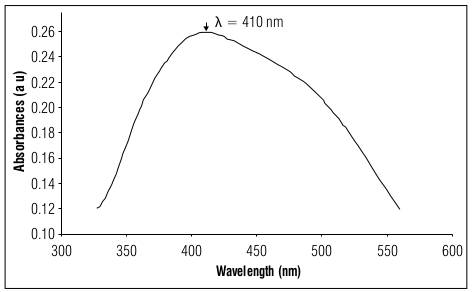
Figure 2. UV-Vis absorption spectrum for the AgNPs sample diluted to 40 mg L-1, in the range of 300 to 600 nm.
The calibration curve of the absorbance for λ = 410 nm of the different dilutions is shown in figure 3, where the linearity followed Lambert Beer ́s law. The adjustment correlation coefficient was very close to one (1.0), giving validity to the experiment data.
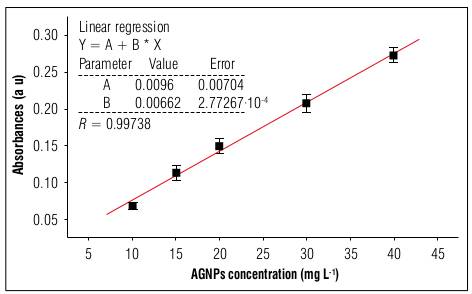
Figure 3 Calibration curve of the absorbance as a function of the AgNP concentration, obtained with absorption spectroscopy in the UV-Vis range, for λ = 410 nm. The solid line represents the best fit to the experiment data with R= 0.99738.
Figure 4 shows a TEM image at 145 kX of the morphology for the AgNPs, as well as the image of the treatment using ImageJ to determine the average Feret diameter or size of the AgNPs, where the diameter was 3.01 nm, with a circularity of 0.71, a minimum size of 0.16 nm and a maximum of 20.97 nm.
Minimum inhibitory concentration (MIC)
Tests on B. glumae with different concentrations of AgNPs and 24 h in constant agitation at 37ºC showed turbidity at concentrations 1-4 mg L-1, where the CMI corresponded to the concentration of 5 mg L-1. At this concentration, no turbidity was observed, indicating growth inhibition of the microorganism (Fig. 5).
Minimum bactericidal concentration (MBC)
In this study, abundant bacterial growth was observed in Petri dishes at a concentration of 1-4 mg L-1 of AgNPs. Nevertheless, no bacterial growth was shown at a 5 mg L-1 concentration tube of AgNPs, which was equivalent to the minimum bactericidal concentration (Fig. 6).
Statistical analysis
Significant differences in the level of absorbance were a parameter to measure the percentage of inhibition of B. glumae at different concentrations of AgNPs. The highest bacterial growth was recorded in the negative control group. The concentrations from 1 to 4 mg L-1 of AgNPs provided higher readings than the commercial antibiotic-based positive control, with an absorbance level of 0.015. Starting at 5 mg L-1 of AgNPs, the absorbance level was negative, indicating that the growth of B. glumae (minimum inhibitory concentration) was inhibited starting at this concentration.
Based on the absorbance level of the negative control group, it was determined that the highest level of inhibition (%) occurred with the use of the commercial antibiotic (positive control), with an average of 93.53%, which was statistically homogeneous to the data obtained with the concentrations from 5 to 10 mg L-1 of AgNPs, determining the minimum bactericidal concentration as 5 mg L-1 , equivalent to the inhibitory results of the commercial antibiotic (Fig. 7).
DISCUSSION
Nanoparticles are increasingly used to target bacteria as an alternative to bactericides (Wang et al., 2017). The widespread use of antibiotics has led to the emergence of resistant bacterial strains. Most antibiotic resistant mechanisms are irrelevant for nanoparticles because the mode of action of NPs is direct contact with the bacterial cell wall, without the need to penetrate the cell (Lallo Da Silva et al., 2019). This is why nanoparticle-based antibacterial activity materials are a viable alternative for disease control (Wang et al., 2017).
This study provided evidence of the effectiveness of silver nanoparticles against the Gram-negative bacterium B.glumae. Silver nanoparticles are known to be highly toxic to Gram-negative and Gram-positive microorganisms, including multidrug resistant bacteria (Losasso et al., 2014). Preliminary studies have shown the strong antimicrobial activity of AgNPs. For example, AgNPs synthesized with a chemical reduction method with sodium borohydride as the reductant and starch as the stabilizing agent were effectively used against Gram negative Escherichia coli (Migula) Castellani and Chalmers and Gram-positive Staphylococcus aureus Rosenbach. A study also indicated its use as an antibacterial coating in food (Vu et al., 2018). In another study, silver nanoparticles were biologically synthesized using Planomicrobium sp., and were tested against a wide variety of food-borne bacteria, including Bacillus subtilis (Ehrenberg) Cohn, Raoultella planticola (Bagley) Drancourt, Klebsiella pneumoniae (Schröter) Trevisanand Serratia nematodiphilaZhang, with a decrease in the growth rate when silver nanoparticles were added to the culture medium (Rajeshkumar and Malarkodi, 2014).
The MIC and MBC AgNPs values of 5 mg L-1, which inhibited the growth of B. glumae, in this study were similar to preliminary studies that found a MIC and MBC of AgNPs against E. coli, K. pneumoniae, Salmonella typhimurium (Löffler) Castellani and Chalmers,and Salmonella sp. of 7.8, 3.9, 3.9, and 3.9, and 7.8, 3.9, 7.8 and 3.9 mg L-1, respectively (Loo et al., 2018). The authors suggested that the greater effectiveness of AgNPs in Gram-negative bacteria is due to the structure of the cell wall. In this study, AgNPs showed good antibacterial activity against the Gram-negative rice infecting bacterium B. glumae. Indeed, the minimum inhibitory concentration and minimum bactericidal concentration assays with AgNPs in this causal agent of bacterial panicle blight (BPB) in riceindicated that, at a concentration as low as 5 mg L-1, the bacterium was not allowed to grow, demonstrating the great potential of these nanoparticles in controlling a disease that is challenging for rice growers.
Silver nanoparticles were electrochemically synthetized in this study to evaluate their effectiveness against bacteria and had the average size reported for NPSs, with a high proportion of Ag atoms on the surface of nanoparticles, suggesting that they can be employed in a wide range of technological applications, including the treatment of pathogens such as B. glumae, which mainly affects rice and agro-processing industries (Dakal et al., 2016; Shanmuganathan et al., 2018; Khalil et al., 2019; Vila Dominguez et al., 2020).
Although BPB significantly affects rice production worldwide, little research has been done to reduce its impact. The lack of chemical or biological control methods and the lack of resistant cultivars make this disease a challenge in terms of management and control (Ortega and Rojas, 2021). For B. glumae, the literature on antibacterial activity using AgNPs is insufficient; however, studies have been conducted using zinc oxide nanoparticles, with promising results for controlling the BPB disease in rice at concentrations of 50 µg mL-1 (Ahmed et al., 2021). The development of new control strategies such as AgNPs to reduce the economic impact of BPB losses provides important advances.
CONCLUSSION
This study showed the potential antimicrobial activity of electrochemically synthesized silver nanoparticles against the bacterium B. glumae. The use of silver nanoparticles at concentrations of 5 mg L-1 ensured inhibition of bacterial growth with results similar to those obtained with a commercial antibiotic. The results of this study revealed that AgNPs are promising nanopesticides for controlling the BPB disease in rice.