INTRODUCTION
The worldwide use of strawberries (Fragaria spp.) either for direct consumption (fresh product) or in the manufacture of desserts, beverages, juices, and other products from fresh, dehydrated, frozen or preserved fruits has led to its cultivation as one of the fruits with the greatest levels of genetic improvement and for the cultural tasks of production, post-harvest handling and marketing (Afrin et al., 2016; Chen et al., 2022).
In addition to being a highly perishable product, producers face the problems of pests, weeds, and diseases during the cultivation, harvesting and post-harvesting of the fruit and these problems limit the production and rapidly diminish the commercial quality of the fruit, generating an increase in the costs of production due to the use of pesticides (Ruiz and Piedrahíta, 2012; Maurya et al., 2019). Consequently, farmers have always tried to minimize the losses caused by these problems with the application of apparently effective methods, based mainly on chemical control, which can be harmful both to the environment and to humans at the time of application or consumption of the fruit itself (Castaño, 2002; Orozco-Mosqueda et al., 2023). Therefore, the crop has been highly dependent on pesticides, with the aggravating effect that its application is directly and specifically on the fruits, which are unprotected structural tissues that can counteract the absorption of chemical molecules associated with the chemical control of diseases (Santra and Banerjee, 2020).
In the production system of high-altitude strawberries (altitudes ranging from 2,000 to 2,800 m a.s.l.), where the main characteristic is high relative humidity (RH greater than 70%), the most relevant disease is gray mold caused by Botrytis cinerea that can cause fruit losses between 40 and 50% of total production (Cano, 2013). B. cinerea is one of the most destructive pathogens during the development of the crop as well as during postharvest, with losses in worth millions of dollars or euros annually (Orozco-Mosqueda et al., 2023).
Even though this pathogen affects all the tissues of the plant (Poveda et al., 2020), the susceptibility of the fruits and the high presence of the pathogen in them due to its aggressiveness, makes it necessary for frequent applications of chemical synthetic fungicides in pre-harvest and post-harvest in order to prolong the useful shelf-life of the strawberry (Chen et al., 2022).
For the specific case of the control of gray mold, farmers use chemical controls with the application of the following active ingredients: captan, chlorothalonil, iprodione, procymidone, fenhexamid, diclofluanid, tebuconazole, methyl thiophanate, and pyrimethanil (FRAC, 2020; Orozco-Mosqueda et al., 2023). Due to the elevated risks caused by the use of these pesticides, such as the generation of resistance in the pathogens to some of these molecules, an urgent need has been generated to search for efficient and low control alternatives with little or no toxicity (Santra and Banerjee, 2020).
During the last two decades, the use of microbial inoculants for the purpose of biofertilization and/or biostimulation and biological control or bioprotection for the management of pests and diseases in crops has increased. This has led researchers to focus some work on the isolation, identification, characterization and large-scale production of these microorganisms as well as the use of mixed inoculations of biological consortiums on crops (Bell et al., 2022; Silva et al., 2022). In this sense, current research aims to evaluate the effect of the inoculation and co-inoculation of microbial consortiums formed by mycorrhizal fungi, antagonistic bacteria and Trichoderma harzianum for the biological regulation of gray mold (B. cinerea) in strawberries (Fragaria × ananassa Duch, var. Monterrey) and the influence of these applications on the production of healthy fruits. To study the effects of these applications spatial and temporal descriptive methodologies as well as the longitudinal modeling of the qualitative diagnosis of the disease by means of Generalized Estimating Equations (GEE) have been used. The results show a significant effect of the interaction between treatments and time of application.
MATERIALS AND METHODS
Area and time of study
We carried out this experiment on the campus of the Universidad de Ciencias Aplicadas y Ambientales (U.D.C.A), in the research and teaching center El Remanso, located at an altitude of 2560 m a.s.l. and with geographic coordinates 4º35′ N and 74º04′ W (Bogota, Colombia). An evaluation period was carried out between December 2014 and October 2015. We planted our cultivars in a production system with free exposure, with eight elevated beds, 0.6 m wide per bed and 0.4 m between rows, 41.22 m long, plants in a 3-roll arrangement of 0.3 m.
During the experiment, a mean annual temperature of around 15°C, precipitation of around 507 to 877 mm and relative humidity of 80 to 90% was recorded (Colombia IDEAM, 2016), conditions that allowed the occurrence of botrytis, due to the history of the pathogen in the study site.
Plant material and field distribution
Strawberry plants (Fragaria × ananassa) Monterrey variety were used, which have a neutral photoperiod, high flowering, intermediate foliage, are vigorous, and with production that can reach 51.72 t ha-1 under optimal conditions, and with individual plant production of fruit around 1,039 g/plant. The fruit possesses good firmness, greater postharvest durability and tolerance to diseases such as Phytophthora sp., Verticillium sp. and Colletotrichum sp. (Dominí, 2012). A total of 1,920 plants were distributed in eight blocks, and in each block eight treatments were applied with 30 plants per treatment in field conditions.
Crop management
Agronomic management of the crop was focused on a system of clean production in which the use of phytosanitary protection chemicals was completely restricted, and, in its place, only cultural practices were carried out such as pruning and handling of infected crop residues. In addition, microbial inoculants were added at the time of transplantation and a re-inoculation was performed 3 months after sowing, as a strategy for the regulation of pathogens. The cultivation system chose the elevation of beds or furrows covered with mulch in order to decrease the presence of pathogens and weeds. This is the same as is done in commercial crops in the Bogota Plateau. A drip irrigation system was implemented together with fertilization. Fertilization was reduced to 50% of the recommended dose according to soil analysis, due to the inoculation of biological products which, in most cases, are sensitive to optimal levels of fertilizers that decrease their effectiveness (Berruti et al., 2016; Silva et al., 2022). The first fertilization was done 3 months after sowing and for the study area used 250 g (N), 300 g (P) and 400 g (K), prepared in mixture in 11 L of water with a frequency of 5 min once a week for four weeks. The next fertilization was performed on the ninth month, increasing the dose of Ca (NO3) 2 to 300 g, 380 g of (NH4) H2PO4 and 480 g of KNO3, and with the same frequency of application.
Application of biological treatments
The following microorganisms were used: mycorrhizal fungi - M (a mixture of four species: Colombian Kuklospora, Glomus manihotis, G. intrarradices, and G. etunicatum), Trichoderma harzianum (T) and antagonist bacteria - B (a mixture of five plant growth-promoting bacteria and phytopathogen antagonists such as Azotobacter chroococcum, Pseudomonas aureofaciens, Bacillus licheniformis, B. megaterium, and B. subtilis [widely known as the biological controller of various plant pathogens and a growth promoter]). Water-soluble inoculations and co-inoculations (combinacion) were carried out in the following manner: control (no inoculations, water); T. harzianum (T); bacteria (B); mycorrhizal fungi (M); T. harzianum + bacteria combination (T + B); T. harzianum + mycorrhizal fungi combination (T + M); bacteria + mycorrhizal fungi combination (B + M) and, T. harzianum + bacteria + mycorrhizal fungi (T + B + M). The inoculations had a dose 5·108 conidia/g of mycorrhiza (Micorrizas Hidrosolubles Fungifert, Agrorgánicos del Llano, Colombia), 2·1010 viable CFUs per species/g antagonist bacteria (Savia Crecimiento, Fundación Semilla Nueva, Buicaramanga, Colombia) and 2 g of active ingredient/100 g of Trichoderma (Trichox, Semillas Valle, Yumbo, Colombia). Each plant per treatment was inoculated with 100 mL of solution Agrotin® (coadyudante) 2 cm3 L-1, at the time of the transplant and 3 months later the inoculation and co-inoculation was repeated.
Experimental design
We used a repeated measures design with inter-subject factors associated with a simple factorial arrangement (a single factor) in generalized randomized complete blocks with eight blocks and eight treatments with 30 plants per treatment. We used the intra-subjects factor at the time of evaluation at 90, 180 and 270 days after the transplant (dat) of the seedlings. We evaluated two response variables, one associated with the disease (absence or presence of botrytis symptoms rate in the fruits rate per plant) and weight of healthy fresh fruit per plant. Dead plants were excluded from the modeling process, since some of them died from causes other than disease, for example, those that died due to stress after the transplant.
Statistical analysis
The descriptive component involved a visual representation of the location and status of the diagnosis of the disease (presence/absence) of each plant in each temporal evaluation. Subsequently, the incidences of the disease were tabulated by treatment applied by sampling time as well as the Area Under the Disease Progress Stairs (AUDPS) described by Simko and Piepho (2012) and together with the associated diagrams. For modeling of the temporal qualitative diagnosis, we used the Generalized Estimating Equations (GEE) using as an intra-subject factor the evaluation time (90, 180 and 270 dat) and as a between-subjects factor for the different biological treatments (Stokes et al., 2012). For modeling the fresh weight of the healthy fruits (g) in the temporal production, an analysis of variance was used for the design in repeated measures but now using the quantitative response. In both methods (GEE and rANOVA) assumptions of their application were reviewed, supported in R software libraries.
RESULTS
The spatial and temporal distribution of the dot pattern associated with the incidence of gray mold disease (B. cinerea) is found in figure 1. The spaces that do not show connectivity between circles identify the dead plants, which were eradicated without further reseeding. The red circles correspond to diseased plants and the green circles to healthy plants. Each treatment appears in the pair of aligned rows, using 30 plants per treatment in each double furrow, which refers to the blocks by spatial distribution resulting in an arrangement in complete and generalized blocks from the point of view of the experimental design. Regarding the design of treatments, only the biological treatment factor was involved with eight levels, with level "control" corresponding to the absence of microbial inoculant.
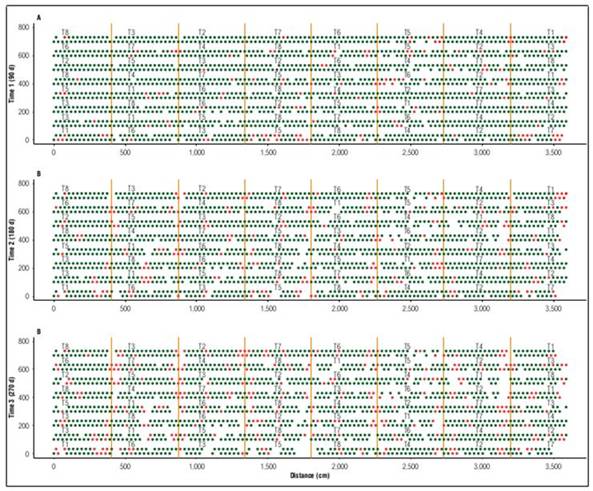
Figure 1. Temporal distribution of the progress of gray mold disease (B. cinerea) in strawberry cultivation var. Monterrey (three time), according to treatment with microorganisms: T1, control; T2, T. harzianum (T); T3, antagonistic bacteria - B; T4, mycorrhizal fungi - B; T5, T + B; T6, T + M; T7, B + M; T8, T + B + M. Green circles, healthy plants; red circles, disease plants; white spaces, dead plant and deleted. d, days after the transplant.
The increase in the incidence of the disease (gray mold) for all the treatments was evident and progressive over time. We also observed a close relation with the decrease in the number of plants for evaluation, increasing the discontinuity of points, most evident for the evaluation in time 3 (270 d). Dead plants are associated with the disease over time from the second evaluation (180 d). Table 1 presents the incidence values of gray mold for each time evaluation for the distribution of figure 1. For the case of control plants, the incidence had the highest values (6.59, 12.09 and 23.08%, at 90, 180 and 270 d, respectively). By contrast, we saw the lowest disease incidence by mycorrhizal fungi treatment with values of 0.89, 5.78 and 13.78%.
Table 1. Distribution of the incidence of B. cinerea in strawberry fruits var. Monterrey, applicating microbial antagonists.
Treatment | Evaluation times (days after the transplant) | ||
---|---|---|---|
90 | 180 | 270 | |
Control* | 6.59 | 12.09 | 23.08 |
T. harzianum (T) | 2.31 | 10.19 | 22.22 |
Antagonistic bacteria (B) | 3.23 | 8.29 | 18.43 |
Mycorrhizal fungi (M)** | 0.89 | 5.78 | 13.78 |
T+B | 4.02 | 8.04 | 17.59 |
T+M | 4.74 | 10.90 | 17.06 |
B+M | 4.61 | 11.06 | 18.89 |
T+B+M | 2.42 | 8.70 | 14.49 |
*Higher incidence rates of gray mold disease (B. cinerea), in Monterrey variety strawberry plants, without microbial inoculants (control).
** Minor incidence rates, stable during the evaluation times for the treatment plants inoculated with mycorrhizal fungi.
The increase of the gray mold was evident con the Area Under the Disease Progress Stairs (AUDPS) (Tab. 2), both by the absolute and relative measurement of the AUDPS in the three evaluations. The treatments Control, T. harzianum and B + M, presented the highest values with 3758.4, 3124.8 and 3110.4, respectively, showing the highest incidence of the disease. On the other hand, the treatments mycorrhizal fungi, T. harzianum + bacteria + mycorrhizal fungi and T. harzianum + bacteria with values of 1,840.5, 2,304.9 and 2,668.5, respectively, had the lowest values of incidence of the disease. As for the treatments T. harzianum + mycorrhizal fungi and bacteria, with values of 2,943.0 and 2,695.5, showed intermediate values.
Table 2. Area under the disease progress stairs of gray mold disease (B. cinerea) in strawberry var. Monterry fruit, applicating microbial antagonists.
Treatment | 90 dat | 180 dat | 270 dat | AUDPS absolute | AUDPS relative |
---|---|---|---|---|---|
Control | 593.1 | 1088.1 | 2,077.2 | 3,758.4 | 0.1392 |
T. harzianum (T) | 207.9 | 917.1 | 1,999.8 | 3,124.8 | 0.1157 |
Antagonistic bacteria (B) | 290.7 | 746.1 | 1,658.7 | 2,695.5 | 0.0998 |
Mycorrhizal fungi (M) | 80.1 | 520.2 | 1,240.2 | 1,840.5 | 0.0681 |
T+B | 361.8 | 723.6 | 1,583.1 | 2,668.5 | 0.0988 |
T+M | 426.6 | 981.0 | 1,535.4 | 2,943.0 | 0.1090 |
B+M | 414.9 | 995.4 | 1,700.1 | 3,110.4 | 0.1152 |
T+B+M | 217.8 | 783.0 | 1,304.1 | 2,304.9 | 0.0836 |
dat, days after the transplant.
Since the presence of the disease is of a categorical nature and the study is longitudinal, it was important to consider the special correlation between the measurements over time. In this sense we used the Generalized Estimating Equations (GEE) strategy developed by Liang and Zeger (1986) (Stokes et al., 2012), where the correlation is adequately modeled for discrete response data (counts of plants with presence or absence of mold), taking into account the structure of the covariance of the response (Tab. 3). The model used the multinomial distribution of the response because it considered healthy, diseased and dead plants and as a function of linking the accumulated logit. The data matrix involved 5,760 observations discriminated by evaluation times and treatments.
Table 3. Overall result of the estimation of generalized equations type 3 (GEE analysis), for control of gray mold in strawberry with eight treatments of microbial antagonists.
Source | DF | Chi-cuadrado | Pr>Chi Sq |
---|---|---|---|
Treatment | 7 | 39.98 | 2.0·10-16 |
Evaluation times | 2 | 260.60 | 2.0·10-16 |
Treatment * Evaluation times | 14 | 39.13 | 3.0·10-4 |
Table 4 shows a clear interaction between the evaluation time and the treatments, for this reason the significance of the main effects was omitted (Montgomery et al., 2012).
For the parallel execution of the analysis, we used the SAS GENMOD procedure (Statistical Analysis Software, v. 9.2) and we applied both the covariance matrix based on the model and the estimated parameters, resulting in similar values suggesting a correct specification of the selected correlation structure. In our case, we used the type (ind) as the working correlation matrix, which is associated with the independent type. We used the analysis as a reference level for treatment at the level associated with the control and for the time the first evaluation was used. Table 4 shows the interaction between treatments and evaluation time, something that is corroborated visually for almost all treatments except for mycorrhizal fungi and control (Tab. 2) for the case of incidence. Although GEE modeling involves plant counts according to their diagnosis, this is the long-term measure that allows one to obtain the incidence itself, so the information in table 2 is useful to understand the nature of the interaction between the factors between -subjects and intra-subjects. Although the lowest and highest incidence corresponded respectively to treatments mycorrhizal fungi and control, in the other treatments the interaction is clarified, since in the evaluation of the 90 dat, the second highest incidence was obtained for T. harzianum + mycorrhizal fungi, at 180 dat it turned out to be Antagonistic bacteria + mycorrhizal fungi and 270 dat T. harzianum. This change in the behavior of the treatments (in terms of the number of diseased plants and therefore the incidence) in the different evaluation times is what generates the presence of interaction, which in turn disables the interpretation of the main effects and treatment.
Table 4. Values of the multivariate test (Wilks Lambda).
Efect | Lambda | Value F | Gl of hypothesis | Gl of error | P value |
---|---|---|---|---|---|
Evaluation times | 0.001 | 1108557.65 | 2.00 | 1665.00 | 2.0·10-16 |
Evaluation times*Treatment | 0.072 | 646.64 | 14.00 | 3330.00 | 2.0·10-16 |
With regard to the production data of healthy fruits, they are also longitudinal in nature, but with a continuous quantitative measure, which required a multivariate analysis of variance approach for the design in repeated measures, using as an intra-subjects factor to the biological treatments and for the evaluation time as the inter-subject factor. The initial analysis involved the blocks, but they were eliminated from the final model because they did not show significant effects from the statistical point of view (something that also happened with the GEE methodology). The need for this approach was due to the non-compliance of the sphericity assumption, since in the case of multivariate normality (Royston's H test by the Royston R library (Korkmaz et al., 2015)) and equality of variance and covariance matrices (Box M test by the biotools library of R (Da Silva et al., 2017) were satisfied for a 99% confidence level.
The results presented in figure 2, are coherent with the commercial production of strawberries that shows a progressive production of fruit through time, achieving a stabilization of production approximately nine months after transplanting for the agroecological condition of this investigation. After 18 months the averages diminish dramatically, consequently the productive cycle can last a maximum of a year and a half for the height of the productive system of strawberries for acceptable production. The most interesting of the results presented in figure 2 is that the trend of production is like the trend of disease incidence for treatments mycorrhizal fungi and control. The superiority and inferiority pattern of both treatments was maintained respectively, with a significant increase in the production averages of healthy fruits at 90, 180 and 270 dat (with values of 241.4, 431.9 and 525.6 g) compared to the averages seen in the control plants of 179.3, 375.9 and 425.4 g, respectively. In figure 2, the increase in strawberry fruit production averaged over time can be seen for the three evaluations carried out. And the production pattern for all biological treatments showed higher averages compared to the plants that were not inoculated (control) even though this pattern changed over time for the inoculated and co-inoculated plants (except for the mycorrhizal fungi treatment that was the best production through the evaluations). For this case, a close relationship was observed between the low incidence of the disease and the high production averages reached by the mycorrhizal fungi treatment.
DISCUSSION
Worldwide, the problems of strawberry cultivation is the high incidence of diseases and for this case in particular, the disease of gray mold (B. cinerea) (Orozco-Mosqueda et al., 2023). A common denominator so that the high incidence of this disease is expressed in the crops and causes losses of between 40 and 60% is the high relative humidity that is the normal condition in the system of production of high-altitude strawberries. In addition, intensive strawberry cultivation for several years at the same site has a negative impact on soil quality affecting native microbial populations, which can be linked to the decrease in fruit yield (Lovaisa et al., 2017). Biologically different studies have been carried out aimed at the management of diseases in the pre- and post-harvest of strawberries, demonstrating that they can be controlled by the use of bacterial, fungal and yeast strains (Poveda et al., 2020). Extracts obtained from liquid mycelial fermentations of the Chilean fungus Stereum hirsutum (Sh134-11) showed antifungal activity against B. cinerea, resulting in the conclusion that the use of biofungicides is possible for the management of this pathogen (Aqueveque et al., 2017). The biocontrol potential of the BUZ-14 strain of Bacillus amyloliquefaciens was proven by the production of antifungal compounds against post-harvest orange diseases (Penicillium digitatum, P. expansum and P. italicum), apple (Monilinia fructicola, M. laxa) and B. cinerea in grapes (Calvo et al., 2017). Films based on thermoplastic starch/montmorillonite containing essential oil constituents (TPS / MMT / EOCs) carvacrol (CRV) and thymol (TML) have antimicrobial effectiveness on B. cinerea in strawberry, without altering quality parameters such as weight loss, firmness, external color, chemical properties, organoleptic properties has been confirmed after discriminant sensory analysis (Campos-Requena et al., 2017). The practical application of the strategy of biologic regulation of pathogens from the mixtures of the microbial inoculants used in this research are fundamental for expanding the range of possibilities for the management of this disease and are completely consistent with the common objective of all these investigations, which is the management of disease with alternatives other than chemical control (Siah et al., 2018).
Microbial inoculations carried out in this research partially reestablish beneficial microbiota for the crop. This directly affects the close relationship that exists between the higher incidence of disease and the decrease in the production of healthy fruits in plants that have not been subjected to biological treatments and the opposite results for biological treatments over time (i.e., low percentages of incidence and higher fruit production, in different proportions) (Tab. 2 and Fig. 2). These inoculations also protect plants from early disease infections due mainly to the presence of mycelia and sclerotia that are present in the dead or deteriorated residues of the previous crop, which is one of the most common forms of contamination (Poveda et al., 2020). In our investigation, it occurred in this way, since gray mold disease appeared spontaneously without the inoculation of B. cinerea, because in the study area it was cultivated with the Albion strawberry variety, which presented losses greater than 30%.
In this research the lower incidence of the disease caused by B. cinerea was in strawberry plants inoculated with mycorrhizal fungi, the biological treatment that also showed the highest average healthy fruit production with consistent results over time (90, 180 and 270 dat) for these two completely interrelated variables. Several authors agree that these results can be achieved with the application of microbial inoculants, which show beneficial effects for plants by stimulating production and regulating the population of pathogens in crops (Maurya et al., 2019; Santra and Banerjee, 2020; De Tender et al., 2021; Sangwan and Prasanna, 2022). They mention that one form of direct biological regulation of pathogens exerted by mycorrhizal fungi is competition for space and nutrients. However, considering that an evaluation of the incidence was made in the fruits, the theory that has a better approximation to what happened could be that the increase in the absorption of nutrients and water provided a better physiological balance and stimulated the plant defense system (Berruti et al., 2016; Maurya et al., 2019; Poveda et al., 2020; De Tender et al., 2021; Sangwan and Prasanna, 2022; Orozco-Mosqueda et al., 2023). This was mainly due to the increase in surface area absorption of root hairs, thanks to the coverage produced by the growth of the hyphae of the fungus, which manage to extend beyond the area of depletion of nutrients and water from the roots. Also, the effect of the inoculation with AMF (arbuscular mycorrhizal fungi), was an establishment of a symbiosis that can be expressed in the plant through an increase in the absorption of nutrients and water, and this provides a better physiological balance, in addition to stimulating the defense system. Therefore, the tolerance to biotic and abiotic factors that affect the normal growth of the plants is the result of a physiological balance that improves the health of the crop and not another control mechanism such as the production of toxins or mycoparasitism (Berruti et al., 2016; Sangwan and Prasanna, 2022).
Regarding the co-inoculation (mixture of several microorganisms) the first moment of evaluation was significant for the low incidence of the mixture of all the microbial inoculants. But the treatment Trichoderma + bacterias + mycorrhizal fungi with a value of 2.42%, in the second and third evaluation showed the best results with the treatment Trichoderma + bacteria, for the second evaluation, with a percentage of 8.04% and the Trichoderma + bacteria + mycorrhizal fungi for the third evaluation, with a value of 14.49%, compared to the values of the control plants (Tab. 2) discussed above.
One of the concerns of the implementation of biological control measures is the compatibility of these with the activities inherent to the crop whether they are cultural, chemical or biological control or between themselves, if co-inoculations. It has been found in several studies that the compatibility of these inoculants with the integral management of crops is related to the complementary effect of management and not as a suppressive of other control measures (Siah et al., 2018; Santra and Banerjee, 2020). In this research, we observed a complementary effect between the microbial consortiums and the cultural control (phytosanitary pruning) for the regulation of gray mold in terms of the low incidence of the disease compared with the control plants. The mixture of microbial inoculants is not a common practice for farmers, especially when many of the multiple benefits for plants and the multifunctionality of rhizospheric microorganisms are not known or understood, specifically on the effects of the biological regulation of phytopathogens and on the biostimulation of plant growth.
The above, agrees with that reported by Orozco-Mosqueda et al. (2023) who mentioned that despite the difficulty of selecting a multifunctional microbial inoculum, microbial combinations might be recommended as biotechnological inputs related to increasing the yield of plants. The authors indicated that the microbial component of soil could play an essential role in the growth and development of plants. However, it is important to consider the specific functionally compatible relationships between the selected microbial consortiums. Microbial combinations could determine greater competitiveness of plants in the field. The activity of beneficial microorganisms in the rhizosphere that are symbiotic and free-living is not only a determinant for the growth and development of plants, but it is also an important potential source for stimulating the resistance of plants to pathogens (Santra and Banerjee, 2020; De Tender et al., 2021). These organisms may also produce biologically active compounds (vitamins, growth regulators, antibiotics, siderophores and plant nutrients) that improve the quality of the cultivated soils and the growth and yield of crop plants (Maurya et al., 2019). Inoculating microbial consortia may increase microbial diversity in the soil, especially in poor or depleted soils, allowing the use of the mineral wealth of nutrients and may improve the growth conditions of crops (Bell et al., 2022; Silva et al., 2022).
There is a growing interest in improving the quality of agricultural products and specifically food safety due to health-related implications, with the consequent added product value and an income increase for farmers. In this sense, the results of this research indirectly related to these issues of quality and safety of strawberries are demonstrably effective, because they produce healthy fruits of high quality, and they reduce the use of agrochemicals. These results include a biological strategy for co-inoculation with mycorrhizal fungi, antagonist bacteria and T. harzianum. Other authors agree with these results when using the biological control strategy such as co-inoculation with mycorrhizal fungi (AMF) and two strains PGPB that resulted in greater production of flowers and fruits strawberry, a larger and firmer fruit size, higher concentrations of sugars, ascorbic acid and folic acid, and a reduction in the acidity of the fruit compared with fruits from un-inoculated plants (Merchán-Gaitán et al., 2014; De Tender et al., 2021). Similarly, Bona et al. (2017) verified that the application of microbial inocula with AMF and PGPB allowed a reduction of chemical inputs and positively influenced the quality of the tomato. In this regard, Sangwan and Prasanna (2022), indicated that bacteria from the rhizosphere stimulate the development of mycorrhizal fungi favoring the growth and yield of many plant species. The growth and proliferation of these bacteria also depends on other microorganisms and the organic compounds present in the soils (Maurya et al., 2019; Orozco-Mosqueda et al., 2023). Also, some of the Trichoderma species are able to colonize the root surfaces and cause substantial changes in the plant metabolism, increasing the absorption of nutrients by the plant and, in turn, the growth of the same, improving fruit production in terms of mass and quality (Poveda et al., 2020; De Tender et al., 2021).
Today, there is no doubt that the search for alternatives for the management of phyto-sanitation, requires us to continue research to find viable and multifunctional alternatives of biological regulation and stimulation of plant growth, factors closely linked, and that resolve more than one of the problems that arise in production. Global warming, climatic irregularity with its various phenomena, rapid, and even more worrisome evolution of species and especially those that are subject to a high anthropic selective pressure, such as insect pests and crop diseases become the greatest challenges for the present for companies producing, marketing and distributing products for the protection of crops. Researchers, instructors and producers are finally called to include the option of biological regulation, as one of the principal options for amending and mitigating the high negative impacts generated by the excessive use of pesticides in agriculture and livestock production.
This research demonstrated that the inoculation and co-inoculation of antagonistic microorganisms is a viable alternative for reducing the incidence of gray mold disease (B. cinerea) in strawberry plants (Monterrey variety) and it increases the production of healthy fruits. The closely related variables of lower incidence of disease, greater production of healthy fruits and vice versa can be compared to the non-application of microbial inoculants. The microbial consortium containing mycorrhizal fungi (individually) stands out against the other biological treatments and is consistent with results in terms of lower percentages of incidence of gray mold disease and a higher average healthy fruit production and demonstrates that the functionality of the mycorrhizal fungi is not limited to favoring the nutrition of the plants and their water status, but they also play a fundamental role in stimulating plant defense and the regulation of pathogens.