INTRODUCTION
Nutrients are next to water the environmental components that greatly affect the productivity of plants (Lambers and Oliveira, 2019). In face of climate change, efficient nutrient use is important for successful fruit tree cultivation, whether to counteract drought conditions, high temperatures or increased CO2 concentrations (Fischer et al., 2022a). Achieving proper fertilization while transitioning to more sustainable agriculture demands our upmost attention (Eisa et al., 2022). In South America, several important crops do not have basic information to optimize fertilization. One of these crops is feijoa (Acca sellowiana [O. Berg] Burret), also known as pineapple guava or cold land guava.
Feijoa shrubs evolved in South America (Southern Brazil, Uruguay, the highlands of western Paraguay and northeastern Argentina) (Parra-Coronado and Fischer, 2013), providing fruits with magnificent characteristics, both for industrial and fresh consumption. A recent study reviewed several aspects, such as polyphenol diversity, nutrient and vitamin contents, and anti-carcinogen (Tortora et al., 2019), anti-inflammatory and antidepression effects, including the use of feijoa peel extract as a sunscreen protectant (Zhu, 2018), although many hours of direct sunshine can burn the skin of the fruit (Fischer et al., 2022b). Research has reinforced the health benefits and industrial qualities that make feijoa a special plant, not only because of the fruit product but also because the crop is desirable since feijoa plants are rustic and thrive in a range of environments (Donazzolo et al., 2019).
Feijoa plantations are found in Colombia, New Zealand, Georgia and Ukraine, and in smaller areas in the United States, Australia, New Zealand, Turkey and China, with orchards in Brazil (Sachet et al., 2019). Feijoa is commercially produced in Colombia in altitudinal regions of 1,800-2,700 m a.s.l., a temperature range of 13-21°C, a minimum of 1,000 direct sunshine hours year-1 (optimum ≥1500), an optimum precipitation of 700-1,200 mm year-1 (upper threshold 2,000 mm) and relative humidity (RH) of around 70% (Fischer and Parra, 2020; Parra-Coronado et al., 2022; Fischer et al., 2022c). In 2019, the departments with high production in Colombia included Boyaca (1,781 t) and Cundinamarca (626 t) with a total of 281 ha and an average yield of 8.57 t ha-1 (Agronet, 2023).
Among macro and micronutrients used in plant nutrition, Ca, Mg and B play substantial roles in growth and development. They are essential nutrient elements for optimal plant growth and are available as Ca2+ (125 mmol kg-1 of dry matter concentration), Mg2+ (80 mmol kg-1) and BO3 3- (2 mmol kg-1) (Kochhar and Gujral, 2020). Gómez (2012) observed that only Mg is mobile in the phloem of plants, and that Ca and B are immobile. However, Moreno-Echeverry et al. (2016) reported that B can be mobile in plants that produce high amounts of sugar alcohols (e.g. sorbitol, mannitol or dulcitol) and mobilize B through the phloem, while Marschner (2012) stated that the mobility of Ca in the phloem is severely low.
Calcium is well-known as a nutrient with hampered mobility in phloem, and its role is associated with structure-providing mechanical stability in tissue (Bautista-Montealegre et al., 2022), mainly because most of it is allocated to cell walls and middle lamella, playing an essential role in membrane permeability and cell integrity (Dwivedi and Dwivedi, 2012). Thus, Ca contributes to the formation of protective physical barriers against the penetration of fungal pathogens (Bautista-Montealegre et al., 2019). Once Ca enters the xylem, it is deposited on cell walls to bind cell wall layers. Afterwards, it is no longer available to the plant; therefore, it is not a recycling element (Brett and Waldron, 1996). Because of its low concentration in the symplast (0.1-0.2 µM), Hirschi (2004) observed that Ca is a major signaler between different tissue and cells (Knight, 2000). More recently, Ca was involved in wood development as a signaler in the lignification process, acting synergically with potassium concentrations (Fromm, 2010) and also to promote growth of salt stressed guava plants applying calcium nitrate (Fischer and Melgarejo, 2021).
Mg is a major nutrient associated with photosynthesis because of its structural role in the chlorophyll, where Mg2+ fulfills a central position in the chlorophyll molecule, coordinating covalently with four nitrogen atoms from the porphyrin ring (Maathuis, 2009). 6 to 25% of the total Mg is attached to chlorophyll, with the majority remaining a soluble compound that differentiates metabolic processes (Marschner, 2012). Mg2+ also promotes the light reactions of photosynthesis in the stroma, activating RubisCo (Maathuis, 2009). 5-10% of the Mg in leaves is strongly bound to the pectin of cell walls (Marschner, 2012). Mg deficiency symptoms are initially noted as chlorosis on the margin towards the inside of fully-expanded and basal (older) leaves because of its high mobility (Gil, 2006; Marschner, 2012).
The deficiency of B is common in tropical areas (Cakmak et al., 2023). B plays an important role in many processes, including transport of sugars, carbohydrate metabolism, respiration, RNA metabolism, phenol and indol acetic acid metabolism, stability and function of cell walls and membranes, and lignification (Marschner, 2012; Cakmak et al., 2023). 90% or more of boron is found in cell walls (Hänsch and Mendel, 2009). Also, B is linked to membrane transport. B deficiencies see reduced H+-ATPase activity (Silva-Cardoso et al., 2014), as well as an altered H+, K+, PO4 3−, Rb+, and Ca2+ ion membrane flux (Brown et al., 2002). In addition, a B deficiency represses root and shoot elongation. The terminal apexes of shoots become necrotic because of inhibited cell division, deforming fruit and flower development; these parameters hamper metabolic communication (Rajendran et al., 2009; Marschner, 2012; Taiz et al., 2017).
A well-made visual diagnosis of the nutritional state of a given plant should include the mobility and physiology of the nutrient, its spatial distribution and location of the symptoms, along with senescence symptoms of the leaves (Gómez, 2012). Nutrient deficiency symptoms are widely used to determine the nutrient demands of a crop and to clarify nutritional disorders, especially when they are specific for a certain nutrient element (Rajendran et al., 2009). The first study on visual deficiency symptoms of nitrogen (N), phosphorus (P) and potassium (K) in feijoa was published by Buitrago et al. (2021).
The symptomatic effect associated with Ca, Mg and B nutrients is well-documented in several commercial crops (Prado et al., 2012; Silva-Cardoso et al., 2014; Cockson et al., 2019); however, this knowledge does not exist for feijoa. Therefore, the objective of this research was to identify and describe the symptoms and growth components associated with Ca, Mg and B deficiencies in young feijoa plants.
MATERIALS AND METHODS
Plants and establishment of the experiment
For accurate field results, this experiment was carried out under field conditions on the El Cortijo feijoa production farm, located at 4°55'9.59" N and 74°17'26.69" W in the municipality of San Francisco (Cundinamarca, Colombia) at an altitude of 2,283 m a.s.l., with an average temperature of 13.2°C, RH between 79 and 84%, and annual precipitation of 1,400 mm. The plants were in an area protected by a 6-gauge plastic sheet, covering only the roof and protecting the plants from direct rainfall. Inside the structure, the temperature reached a maximum of 22°C at noon.
The 'Quimba' variety (clone 41) was used. Seventy-two 9-month-old plants (including the border plants) from the farm's nursery were used, propagated with cuttings, with an average height of 1.25 m. The plants were transplanted into 10 kg plastic bags filled with inert substrate, pale gray quartz sand (grain size of 1.2 mm and CE of 0,011 dS m-1). To promote root emergence after transplant, a 2-month (June 30-August 31) adaptation period was used. The treatments started on August 31 and lasted until December 11. The plants were arranged in three rows, with 0.25 and 0.4 m between rows and plants, respectively.
Quantitative variables were evaluated every 15 d starting at day 39 of the treatments for apical growth, production of apical and basal shoots and leaf chlorophyll content, along with symptoms determined for each nutritional deficiency, including chlorosis, marginal necrosis, meristem wilt, and retarded growth. Chlorophyll content index (CCI) units were determined with a portable chlorophyll meter (CCM-200; Opti Sciences, Tynsgboro, MA). The procedure was done in triplicate for each replica in leaves from low, medium and high levels. The growth rate (cm d-1) was calculated based on Hunt (1990), determining the change in the length of lateral shoots per time unit. A description of the symptoms manifested in the plants was carried out with a photographic record. This activity was done every 8 d starting at 20 d after starting the experiment.
Following Martínez et al. (2009), a modified Hoagland and Arnon solution was applied for each treatment (Tab. 1), suppressing one element in each treatment (Tab. 1). Element doses were used based on guava extraction levels and foliar analyses adapted from feijoa (Fischer et al., 2003; Natale et al., 2009; Gómez, 2012). The prepared mixture received 1 L concentrations, applying them twice a week with irrigation water.
Table 1. Missing nutrition treatments (mg L-1).
Nutrient | Treatment | ||||
---|---|---|---|---|---|
-Ca | -Mg | -B | T0 | H2O | |
N | 221 | 221 | 221 | 221 | 0 |
P | 30 | 30 | 30 | 30 | 0 |
K | 240 | 240 | 240 | 240 | 0 |
Ca | 0 | 150 | 150 | 150 | 0 |
Mg | 40 | 0 | 40 | 40 | 0 |
B | 0.53 | 0.53 | 0 | 0.53 | 0 |
The irrigation water was validated with a physicochemical analysis to prove its suitability for the experiment. The compounds were: calcium nitrate (Ca(NO3)2), ammonium phosphate ((NH4)3PO4), urea (CH4N2O), dipotassium phosphate (K2HPO4), potassium nitrate (KNO3), potassium sulfate (K2SO4), calcium chloride (CaCl2), magnesium sulphate (MgSO4), sodium borate (Na2B4O7·10H2O), and chelated compounds of EDTA for minor nutrients (Fe, Zn, Cu, Mn, Mo, Co).
Data analysis
A completely randomized design (CRD) was used, defining one plant as an experiment unit. Five treatments with nine repetitions were set up. Six samplings (five non-destructive and one destructive) were done at the end of the experiment. The data were analyzed using ANOVA and Tukey tests with a significance of 5% and SAS v. 9.2 for quantitative variables.
RESULTS AND DISCUSSION
Symptoms
Once 60 d of the treatments had passed, symptoms were detected, which were consistent for most nutrients. Only Ca had an earlier response, after 30 d of treatment, but it’s singular leaf symptom only appeared after 75 d. Mg and B deficiencies were associated with malformations in shoot and leaves. Additionally, B deprivation resulted in structural loss that was evident in the leaves, which became weary and faint. Figure 1 shows one representative plant for each treatment. Interestingly, most Mg deficient plants that were treated with water (negative control) released most of their leaves and become nearly naked. In the -Ca and -B plants, this also occurred, but only in the upper part, indicating a mobility effect with these two nutrients (Marschner, 2012). Citrus trees may defoliate completely under a Mg deficiency and high fruit production (Zekri and Obreza, 2013). New biomass production has a high demand for Mg, and a serious Mg deficiency culminates in early leaf shedding (Pallardy, 2010). In this experiment, early leaf shedding occurs specifically in Mg repetitions with no new shoots. Defoliation was also noted in the negative control. Dussán et al. (2016) reported that deficiencies had an effect on distribution matter in guava (Psidium guajava L.), where the Mg deficiency limited leaf production and resulted in the poorest treatment for foliage generation. Thus, similarities with other crops, not only with myrtaceous, were observed for feijoa.
Calcium
Symptoms started 30 d after applying treatments. As an early symptom, young leaves shriveled and struggled to expand. Early necrosis occurred, advancing from the leaf tips to the leaf base, encompassing the distal half (Fig. 2). This also occurred in young leaves at the plant base. The second symptom occurred after 75 d, with a visible symptom throughout all plants in the treatment group, reddish and black veins in young, mature leaves, advancing from the apical portion towards the central portion (Fig. 3).
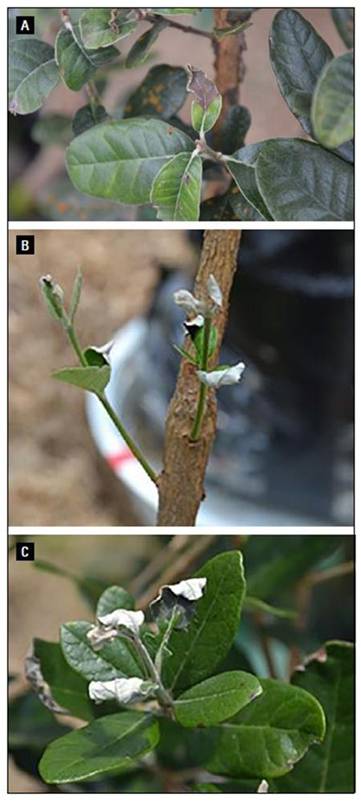
Figure 2. Evident symptoms associated with a calcium deficiency in feijoa. A. Distal necrosis in young leaves. B. Wilted tips in basal shoots. C. Wilted apical leaves.
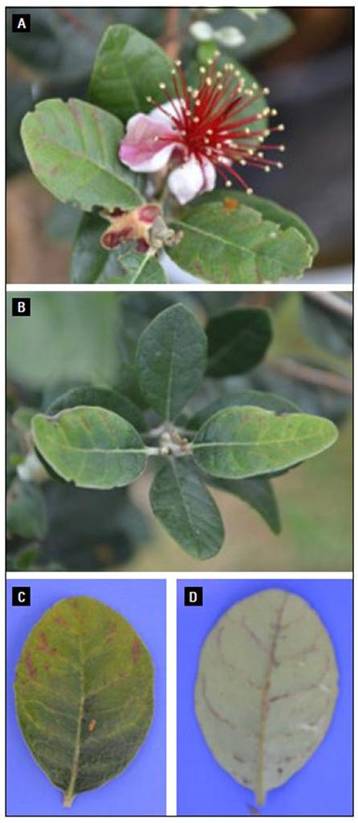
Figure 3. Evident symptoms associated with a calcium deficiency in Feijoa. A. Intravenous reddish in young, fully-expanded leaves. B. Intravenous reddish in young, fully-expanded leaves. C. Reddish veins in foliar adaxial view. D. Reddish veins in foliar abaxial view.
Tip necrosis occurs mainly because Ca is not sufficiently mobile in plants; hence, when limited, cell wall production in distal zones of young organs is impaired by a lack of cell-to-cell adhesion, and necrosis occurs (Marry et al., 2006), confirming its crucial role in strengthening cell walls and plant tissue (Marschner, 2012; Prabhakar et al., 2016). Additionally, Ca is only transported via xylem, and organs with a low transpiration rate, such as young leaves, are especially vulnerable to Ca deficiencies, leading to necrosis (Dwivedi and Dwivedi, 2012). Equally, in cape gooseberry, new leaves of Ca deficient plants crinkled and shriveled (Silva et al., 2017). Interestingly, visibly symptoms were broadly expressed in young and fully-expanded leaves; thus, its expression is an earlier indicator of Ca deficiencies. In crops, this symptom is easily noted and can be used as an indicator to correct Ca fertilization (Fig. 3) or add an extra foliar application. Unfortunately, these symptoms have not been reported before in similar crops, such as guava, or in eucalyptus, another member of the Myrtaceae family, but the apical bud died in both eucalyptus and feijoa (Silva-Cardoso et al., 2014).
Magnesium
Magnesium symptoms were mainly black spots in the leaves (Fig. 4 and 5A) and malformations at growth points. In the early treatment stage, the leaves exhibited interveinal chlorosis (<30 d), similar to avocado (Hurtado et al., 2019), followed by black spots and malformations and necrosis spreading to different growth points. Interestingly, several leaves were not able to expand properly and developed an invagination in mature leaves. This might have occurred because Mg is essential to ribosomes integrity, so cell division and protein biosynthesis disruption result in an inability to complete organogenesis (Pallardy, 2010). This was also observed in eucalyptus (Silva-Cardoso et al., 2014). Figure 5A shows a common response to meristematic death, which in turn produces an overproduction of shoots downward to the apex. These symptoms have been reported with a B deficiency by Shireen et al. (2018).
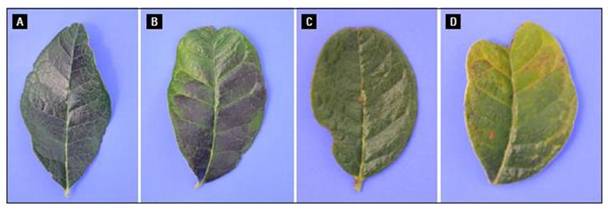
Figure 4. Evident symptoms associated with a magnesium deficiency in Feijoa. A. Black spots in an old leaf. B. Black spots advancing acropetally. C. Malformations caused in young leaves. D. Reddish veins in foliar adaxial and tip malformations.
Another symptom was observed in mature leaves: swelled central tissue with a chlorotic leaf edge (Fig. 5B). This particular symptom might be associated with an incapacity to transport substance between tissue. Mg is critical to loading sucrose into phloem for long distant transport (Cakmak and Yazici, 2010). Given its ligand property with other compounds, Mg facilitates several communication functions (Marschner, 2012). For instance, the Mg/ATP and H+-ATPase substrate drive sucrose export from foliar cells to the phloem (Tränkner et al., 2018). A Mg deficiency sees sucrose and starch accumulation in the leaves and reduced CO2 fixation (de Bang et al., 2021). Hence, an accumulation of certain substance might indicate a symptom.
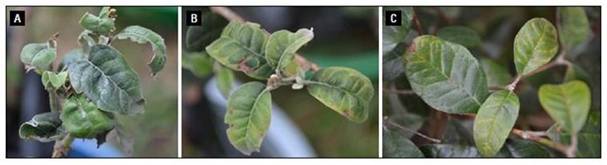
Figure 5. Evident symptoms associated with a magnesium deficiency in Feijoa. A. Slight curled malformation associated. B. Leaves with interveinal chlorosis and swelled tissue in the inward portion. C. Intervein chlorosis in fully-expanded leaf.
Several leaves had black spots in expanded leaves and necrosis in folded leaves (Fig. 6). Black spots in expanded leaves appeared to be a l symptom of particular to the Mg deficiency in feijoa, different from many other fruit species, and could also be related to the light intensity in the growth chamber of the experiment (Cakmak and Yazici, 2010). Necrosis has also been reported in avocado but with a different pattern (Barnard et al., 1991) that does not reach unfolded leaves but has small necrotic spots on the tip of fully-expanded leaves. During the last part of the experiment, several Mg deficient leaves started to fall, leaving the plants naked. De Bang et al. (2021) also reported results where a Mg deficiency induced root stress via sugar deprivation, producing ethylene and leading to leaf detachment. Ethylene production has been reported as a consequence of Mg deficiencies, inducing root hair growth more than defoliation (Liu et al., 2017). In this experiment, only the -Mg plants and negative control presented strong leaf loss; thus, ethylene overproduction might not be a suitable explanation because of its gaseous form; no other treatment plants experienced this (Adams and Yang, 1981). Recently, Mg was associated with leaf senescence and loss, oxidative damage causing changes in redox states, metabolic alterations and photosynthesis disruption that underpins defoliation (Tanoi and Kobayashi, 2015).
Boron
A boron deficiency predominantly damages actively growing organs, such as shoot and root tips, so the whole plant may be stunted (rosetting) as Han et al. (2008) observed in boron-starved citrus cuttings.
The first symptoms associated with a B deficiency are related to chlorosis and disruption of leaf expansion. Leaf turgid was ameliorated, and chlorosis occurred in several young leaves. In mature, young leaves, chlorosis occurred in the second-half, veins and margins (Fig. 7). Interveinal chlorosis was noted, with some necrosis at the distal part in young leaves. Interestingly, leaves became wilted and thinner than in other treatments, which might confirm the role of B as a structural element in the membrane and in lignification (Brown et al., 2002; Cakmak et al., 2023). During the study, there was no effect on growth in the control plants; perhaps the experiment was not long enough to induce a stunting effect.
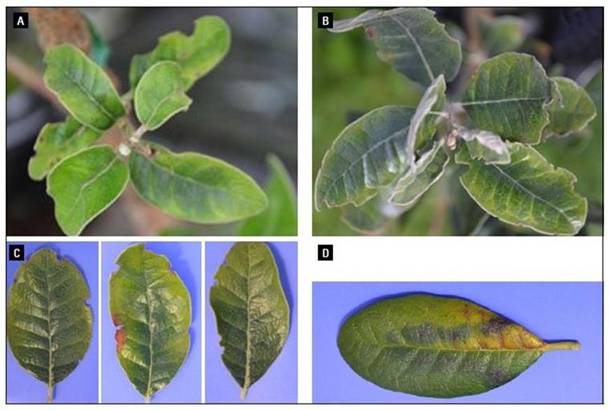
Figure 7. Evident symptoms associated with a boron deficiency in Feijoa. A. Chlorosis in, overall and margins, young leaves. B. Intervein chlorosis in fully-expanded, young leaves. C. Chlorosis and degraded green in fully-expanded, young leaves. D. Uncommon pattern associated with a B deficiency.
In guava, B deficiencies are expressed differently, with stunted growth and buds that fail to open, while others open but then shrivel and die (Kumari et al., 2018). In other crops, such as blackberries, major disorders were mentioned, including multiple architecture shoot disorders with a higher production of rosette-shaped shoots in the entire plant (Castaño et al., 2008). Although this abnormal growth has been noted in many production sites, during the experiment, this result did not occur with the B deficiency. Instead, it was seen with the Mg deficiency, which might be explained by small quantities of B that remained in cuttings from earlier growth, which overcame the deficiency limitations throughout the experiment (Fischer et al., 2003; Fischer et al., 2020). Young leaves require boron because of its role in cell division and elongation (Shireen et al., 2018). In feijoa, new leaves also showed strong chlorosis, as noted in Figure 7A, and showed misshaped leaf blades (Cakmak et al., 2023).
Additionally, an unusual pattern was noted in B deficient plants (Fig. 8), with a mosaic of integrating patterns. Marginal and central vein chlorosis were observed after black patches in the leaf base (Fig. 8B). This was due to B’s functional role in membranes. Studies have indicated alterations of H+, K+, PO4 3−, Rb+, and Ca2+ ions with a B-deficiency in Vicia faba (Robertson and Loughman, 1973), which produced a camouflaged deficiency of those elements. Also, B is involved in phenolic metabolism (Cakmak et al., 2023), and, as mentioned, the feijoa plant is a high phenol producing plant, not only in the fruits but also in the leaves (Zhu, 2018). Thus, black patches are probably the result of oxidation caused by elevated polyphenol concentration. This substance is metabolized by the B-sugar cis-diol complex, which regulates phenol accumulation and maintains its proper metabolism. Hence, because of the absence of B, this metabolism is disrupted, and oxidation damage appears (Wimmer et al., 2019). Marschner (2012) reported that a B deficiency decreases usage of absorbed light in photosynthesis, generating phenolics oxidation and hindering the defense mechanism of antioxidants in plants, causing a higher susceptibility of plants to excessive light intensity and ROS formation.
Effect on some growth variables
Nutrition in plants is essential to obtaining the desired yield. Plant production requires all essential nutrients to develop all functions that provide a product with known quality (Rajendran et al., 2009). Because each distinct nutrient plays a strategic function in the plant, there is a physiological expression caused by its particular depletion (van Maarschalkerweerd and Husted, 2015). To test growth, several quantitative variables were measured to provide information on plant performance with a deficiency.
The apical, longitudinal growth did not show differences between the distinct treatments; however, decreased growth was recorded for all treatments. Surprisingly, B had the smallest effect, possibly because of the short experiment time (Fischer et al., 2020), followed by magnesium and calcium. Apical growth requires healthy photosynthesis to meet the demand for energy (Pallardy, 2010). With 4.17 cm, Ca showed less apical elongation. de Bang et al. (2021) reported that a deficiency produces meristem death and necrotic lesions on leaf margins and tips, which was also reported in this experiment. This result is supported by the Ca structural function in budding and rooting (Marschner, 2012).
For apical shoot production, only Mg presented a significantly reduced result, which is underpinned by its role in root growth, transport and carbon allocation, which are linked to photosynthesis (Cakmak and Yazici, 2010; Marschner, 2012). These functions were disrupted, halting shoot meristems production. Interestingly, neither Ca nor B had a significant reduction in shoot production. Both calcium and magnesium are widely known as cooperative nutrients that have a complementary role (Rajendran et al., 2009). Differences in cell wall chemistry based on Mg and Ca concentrations allow plants to execute distinct stoichiometric equations. Thus, feijoa plants are more sensitive to Mg absence because of its role in carbon allocation (de Bang et al., 2021). The Mg deficiency was observed early despite its property of fast ligand exchange kinetics, which result in easy substitution with several positive ions, including Mn2+, Ca2+, Fe2+, Co2+, Cu2+ and Zn2+ (Allnér et al., 2012). These authors indicated that hampered growth may still occur, mainly because Mg substitution slows down enzyme activity (Schmidt and Husted, 2019).
Basal budding occurred in feijoa; however, in each treatment, different tendencies occurred because of the lack of significance in the results (Tab. 2). Plants without Ca in the nutrient solution tended to produce 19 times more basal shoots than the negative control. In previous experiments, a lack of nitrogen resulted in an almost total remove of basal shoots, while a K deficiency increased the number. This was associated with an osmotic disruption caused by K deprivation that promoted shoots at the plant base (Buitrago et al., 2021).
Table 2. Variables of growth and chlorophyll content in feijoa cuttings after 102 d in the experiment, with missing elements of -Ca, -Mg and -B, control (complete nutrition) and only water (negative control).
Variable | - Calcium | - Magnesium | - Boron | Control | Only H2O (negative control) |
---|---|---|---|---|---|
Apical growth (cm) | 4.17±3.87ab | 5.50±3.20 ab | 8.08±5.11 ab | 9.14±4.53 a | 1.94±4.91 b |
Number of apical shoots | 9.88±6.64 ab | 4.67±3.31 bc | 8.44±7.43 ab | 12.89±5.46 a | 1.00±2.00 c |
Number of basal shoots | 2.11±2.03 ab | 1.22±1.50 ab | 0.78±1.10 ab | 1.56±1.59 ab | 0.11±0.33 b |
Chlorophyll content index (CCI) | 83.9±11.83 a | 86.11±15.17 a | 79.78±15.13 a | 86.66±11.24 a | 67.01±13.50 b |
Growth rate (cm day-1) | 0.036±0.043 c | 0.044±0.051 bc | 0.110±0.064 ab | 0.161±0.11 a | 0.067±0.024 b |
Averages with different letters in the same row indicate a significant difference according to the Tukey test (P<0.05).
Chlorophyll content is a common measure used to gain information on the nutrition status of a plant (Roveda-Hoyos et al., 2022). Only the negative control accumulated a significantly lower content in this variable (Tab. 2). Mg is a main component of tetrapyrrole ring in chlorophyll (Taiz et al., 2017); however, its content was not lessened with the Mg deficiency in this experiment. This might have occurred because only 5-25% of the total absorbed Mg was established in the chlorophyll ring (de Bang et al., 2021). The second role of Mg is related to the cation exchange capacity of the cell wall, modulating Ca and Mg and additional signals (White et al., 2018).
The depletion of Ca and Mg strongly and significantly impaired growth rate, which decreased 27 and 22% from the control treatment, respectively (Tab. 2). This reaction occurred because Ca not only belongs to the cell wall as a structural element but also influences cell wall permeability by creating bridges with phosphate and carboxylate groups in lipids and proteins (de Bang et al., 2021). Calcium is important as a signaling substance, sensors include calmodulins (CaMs) and CaM-like proteins (CMLs), which promote protein activation with Ca binding, which in turn induces gene regulation (La Verde et al., 2018). Hormones are also related to Ca signaling. For instance, the ABA action is Ca dependent, via CPKs/CDPKs and calcineurin B-like (CBL) receptors that allow ABA to act on guard cells. Mutants lacking CPK do not react to ABA stimulation or, therefore, to droughts (Geiger et al., 2010). For magnesium, the Mg-starved plants showed not only a significantly lower apical shoot production but also a lower growth rate than the control plants. As previously stated, a Mg deficiency can decrease growth because of the deficient activation of enzymes involved in photosynthesis, respiration, and DNA and RNA synthesis (Taiz et al., 2017; Schmidt and Husted, 2019). In addition, its role as the central Mg atom of chlorophyll molecules that absorb photons and initiate electron flow (Marschner, 2012) means that any deficiency of this nutrient affects photosynthetic performance. On the other hand, Cakmak and Yazici (2010) reported a high accumulation of sucrose in Mg-deficient leaves, indicating severe inhibition of sucrose transport out of Mg-deficient leaves, impeding photosynthesis.
CONCLUSION
This experiment offered a first approach to exposing symptoms related to calcium, magnesium, and boron deficiencies in feijoa plants. The Ca deficiency was noted early in the shoot tips and later in fully-expanded, young leaves. Mg-deficiency symptoms were seen in older and fully-expanded leaves, mainly as interveinal chlorosis. The B deficiency produced a variety of symptoms, including interveinal and total chlorosis in fully and not fully-expanded leaves and a mosaic of symptoms in older leaves. The number of apical shoots was reduced by the Mg deficiency, and the seedling growth rate was lower in the Ca- and Mg-starved plants. In future experiments, the authors suggest an experiment period longer than 102 d to achieve more conclusive results, using cuttings that are no more than 4-months old.