INTRODUCTION
Increased urbanization and industrialization have led to an increase in urban environmental pollution (Zwolak et al., 2019; Haider et al., 2021). Noonan and Barreau (2021) estimate that the world population will rise to 8.6 billion by 2030 and to 9 billion by 2050, with two thirds living in cities, requiring increased global food production on a large scale. In Latin America (including the Caribbean), 75% of the population lives in cities, and it is estimated that this number will increase to 83% by the year 2030 (Olivares et al., 2013).Increased urbanization means that the majority of the world’s population will live in cities and will require biological resource inputs and a healthy living environment (Yang and Yang, 2022).Meanwhile, the peri-urban zone represents the transition between urban and rural areas, playing an important role in the maintenance of urban ecosystems and safeguarding food security for the urban population (Jansma and Wertheim-Heck, 2022). Investigation results have found that urban systems provide many opportunities for the application of sustainable horticulture in urban areas through waste management and farming, that could together reinforce each other (Haitsma et al., 2022).
For these reasons, it is important to promote urban horticulture (the primary form of urban agriculture) (Crispo et al., 2021) and peri-urban horticulture, to supply the rapidly growing population of cities with fresh vegetables and fruits (Cohen and Wijsman, 2014); however, air, water and soil contamination are a barrier to achieving this goal (Miranda et al., 2008a).Heavy metal (HM) contamination of air, water, and soil strongly affect the food security of millions of people by causing reduced development of vegetables and, consequently, deficient crop yield (Elango et al., 2022).
Despite the positive perception of current urban horticulture and locally produced fresh vegetables, the possible food safety risks linked to these could be underestimated, inducing regulatory gaps (Buscaroli et al., 2021).
The United Nations (2020) classifies cities as one of the factors that most influences global warming, given that they use 78% of the world's energy and emit 60% of greenhouse gases, while covering less than 2% of the earth's surface. In addition, because of climate change, urban pollution increases with the increase in temperature (Santamouris et al., 2015), fostered by so-called "urban heat islands" (Ulpiani, 2021), as confirmed by Fischer and Beltrán (2021) by comparing three locations in Bogota (Colombia). In this context, urban horticulture is capable of mitigating the effects of climate change by growing the crops best adapted to local conditions, thus substituting a great part of the food produced from regular supply systems (Kulak et al., 2013), and sustaining various ecosystem services such as carbon storage (Dobson et al. (2021). The high temperatures in the urban area associated with the reduction of relative humidity also hinder agriculture because they can exceed the optimum ranges for plant growth in the city, e.g. in the case of the tomato, whose optimum temperature is18-27°C and cannot withstand temperatures that exceed 35°C (Vallejo and Estrada, 2004). In addition, high temperatures can accelerate the phenology of the plant, especially in short-cycle vegetables (Chaves-Barrantes and Gutiérrez-Soto, 2017). On the other hand, in temperate zones of the northern hemisphere, the increase in temperature in cities due to heat islands and global warming offer possibilities for cultivating new horticultural species in urban gardens, with higher yields than in the past (Waffle et al., 2017).
Among urban agricultural plants, vegetables rank first because they are nutrient-rich foods and occupy suitable soils most efficiently because they are perishable and high-value (Weidner et al., 2019; Hume et al., 2021). Vegetables constitute an essential source for human nutrition, providing vitamins, minerals and fibers, as well as having important antioxidant effects (Begum et al., 2019). However, in food, both essential and non-essential elements can be harmful to human health if consumed in high concentrations, particularly heavy metals (HM) (Marschner, 2012), including the metalloid arsenic (As). The properties of metalloids are intermediate elements between metals and non-metals (Nag and Cummins, 2022). In the text of this article, As is included in the group of HM, despite being a metalloid.
In nature, HM are widely distributed, which makes their presence in living beings essential (Miranda et al., 2022). The processes that control the mobility and availability of HM are complex, these being factors of geochemical, biological, climatic and anthropogenic origin (Kabata-Pendias, 2004).
As they are biologically toxic, non-degradable and persistent, HM have received much consideration (Yu et al., 2022). The uptake of HM by plants generates several dangers for the environment that McLaughlin (2002) classifies mainly as (a) incorporation of HM into the food chain, (b) detriment of plant cover induced by phytotoxicity and (c) the cycling of metals to the superficial layers of the soil by tolerant plants, causing toxic effects on fauna and flora.
The rapid growth of industry and, in many cases, disorganized urbanization, have created a considerably higher risk of environmental contamination through HM (Zwolak et al., 2019). This situation, together with the application of large amounts of fertilizers and pesticides in agriculture, leads to the accumulation of toxic substances in the air, water, and soil (Kumar et al., 2015; Rodrigues et al., 2017).
Generally, HM have been classified according to their specific density, as elements with a density ≥5 g cm-3 (Covarrubias and Peña, 2017; Lambers and Oliveira, 2019) or 4 ≥ g cm-3 (Londoño-Franco et al., 2016). However, another term to refer to HM is toxic metals, due to their toxicity in ionic form (Epstein and Bloom, 2004), with resulting negative consequences (Alloway, 2013).
There are multiple reports on the presence of HM, such as cadmium (Cd), lead (Pb), chromium (Cr) and mercury (Hg) in higher plants, mainly due to environmental contamination, variations between genotypes in the critical levels of toxicity of these HM in plants and the appearance of HM in the food chain (Marschner, 2012).
Zwolak et al. (2019) mention that in addition to their wide distribution in the environment, heavy metals are notable chemical contaminants in food. Zwolak et al. (2019) group heavy metals such as the so-called micronutrients Fe, Mn, Cu, Zn and Mo into essential elements for metabolic processes; in excessive amounts these are more harmful to plants than to animals, while elements such as Cd, Pb, Hg and As, even in low concentrations, are very harmful to animals and humans. Likewise, they affect, to a lesser degree, the growth and development of plants.
The Codex Alimentarius defines a contaminant as “Any substance unintentionally added to food as a result of the production (including operations carried out in crop husbandry, animal husbandry and veterinary medicine), manufacture, processing, preparation, treatment, packing, packaging, transport or holding of such food or as a result of environmental contamination” (FAO-OMS, 2009).
Due to its complexity, the topic “bioremediation”, which involves the use of plants (phytoremediation) or microbes (microbial bioremediation) and is one of the most efficient procedures to eliminate HM from the soil (Elango et al., 2022), is not included. Bhat et al. (2022) described these eco-friendly and sustainable methods of phytoremediation of HM in water and soil as amultidisciplinary approach.
Studies on HM in urban and surrounding areas are scarce but, due to the risks posed by HM to the health of citizens, they are imperative (Antisari et al., 2015; Augustsson et al., 2023a). The objective of this document is to provide a description of the impact of HM contamination on vegetables cultivated in urban and peri-urban areas, reviewing results from various countries and regions affected by this problem that affects the health of consumers.
METHODOLOGY
The information in the ScienceDirect database was used in accordance with the PRISMA guide, applying the modified methodology of Page et al. (2021). In a first step the keywords “vegetable”, “contamination” and “urban” were used for the last 10 years (2014-2023), which generated 361 articles, of which 25 (6.9%) were useful for this article. In a second step the keywords “vegetable” and “heavy metal” were used for the last 5 years (2019-2024), finding 1,640 articles of which 27 (1.6%) were included. Because of their importance, some studies from previous years and other databases, e.g. ResearchGate were also used.
HEALTH EFFECTS DUE TO THE CONSUMPTION OF CONTAMINATED VEGETABLES
To comply with the WHO/FAO recommendations to consume a minimum of 400 g of fruit or vegetables (excluding potatoes, sweet potatoes, cassava and other starchy tubers) per day to prevent chronic diseases, the consumption of vegetables is very important (WHO, 2020) and its demand, especially in cities, is high.
Studies have shown that vegetables grown in contaminated urban and peri-urban soils may contain high concentrations of trace metals that may exceed acceptable limits for food safety (Nabulo et al., 2010; 2012). The human body needs very low concentrations of iron (Fe), copper (Cu), cobalt (Co), manganese (Mn), zinc (Zn), molybdenum (Mo), strontium (Sr) and vanadium (V) (Londoño-Franco et al., 2016).
Within the HM found in foods from urban and peri-urban areas that affect the health of living organisms (including humans) Zwolak et al. (2019) and Filipiak-Szok (2015) established the following descending order: Hg > Cu > Zn > Ni > Pb > Cd > Cr > Sn > Fe > Mn >Al, which, in small amounts, represents the danger they pose to health. The minimum that the consumer should do is wash the vegetables before consuming them. Augustsson et al. (2023b) verified in their study with Pb that the average daily consumption of this HM would augment by 130% if contaminated vegetables are not washed before eating them.
According to the WHO (2021), HM such as Cd, Pb and Hg cause neurological and kidney damage (Tab. 1), and are even more harmful if consumed over time throughout prenatal development and childhood, leading to irreversible alterations in the central nervous system (Zwolak et al., 2019).Through evaluating reports of Cd content in spinach, potatoes, rice, oats, barley and wheat in the United States, Pokharel and Wu (2023) found that the infant and child age groups (6-24 and 24-60 months old) are the most vulnerable to Cd in these foods that surpasses the maximum tolerable intake amount.
Table 1. Original sources and important effects on human health by HM.
Heavy metal | Sources | Health effects |
---|---|---|
Cadmium | Mining, plastic, refining, welding, fertilizers, pesticides | Kidney damage, gastroenteritis, bronchitis, bone marrow, cardiovascular disease, lung failure, cancer, itai-itai disease, hypertension, weight loss, nervous disorders |
Lead | Mining, pigments, paint, battery manufacturing, electroplating, welding, coal burning, pesticides | Neurological damage, anemia, general malaise, anorexia, liver, kidney and gastrointestinal damage, hypertension, encephalopathy in children, immune and reproductive system disorders |
Mercury | Mining, paint industry, paper industry, batteries, pesticides | Corrosive to skin, eyes and muscles, nervous system damage, protoplasm poisoning, pneumonia, kidney damage, dermatitis |
Arsenic | Mining, smelting, rock sedimentation, paints, pesticides | Bone marrow depression, bronchitis, hemolysis, dermatitis, cancer, hepatomegaly, carcinogenic, neurotoxic, and genotoxic disorders |
Zinc | Mining, refineries, plumbing, brass manufacturing, fertilizers | Gastrointestinal upset, short-term metal fume fever |
Copper | Painting, plating, construction, copper polishing, printing operations, fungicides | Neurotoxicity and acute toxicity, neonatal ataxia, heart failure, diarrhea |
Nickel | Non-ferrous metal, porcelain enameling, electroplating, paint formulation | Lung cancer, reduced lung function, chronic bronchitis |
Source: according to Londoño-Franco et al. (2016); Sandeep et al. (2019) and Zwolak et al. (2019).
Li et al. (2023) modeled the health risks from anthropogenic activities on soil contamination and found that, in a large Chinese city, atmospheric deposition with HM contributes more to the carcinogenic risk in children than to adults, while the intensity of traffic and agricultural activities also has an effect on adults. In the assessment of the non-carcinogenic and carcinogenic risks of the population from HM, calculations such as (1) the daily consumption of vegetables and (2) the estimated daily intake of HM relative to weight of the consumers are used, also considering the exposure during the days of the year for a total lifetime (70 years). For more details on the health risk assessment of HM, see, among others, Liang et al. (2018) and Sharma et al. (2009).
Due to the threat to human health from the ingestion of toxic metals in food, permissible levels in food, with an emphasis on processed foods, have been adopted by institutions and countries including the European Union (EU Commission Regulation No. 1881/2006 of 19 December 2006 and amending Regulation No. 420/2011 of 29 April 2011). The FAO/WHO publishes the Codex Alimentarius CXS 193-1995 (amendment 2019), which established the maximum levels for cadmium, lead, mercury, arsenic and tin in food, while Resolution Number 4506 of October 30, 2013 of the Ministry of Health and Social Protection of Colombia regulates maximum levels for cadmium and lead in vegetables.
For the different species and types of fresh vegetables, the maximum permissible concentrations with Pb and Cd have been established. Thus, EU Regulation 2021/1323 of August 10, 2021, for Cd, and EU Regulation 2021/1317 of August 9, 2021, for Pb, establish 0.02 and 0.05 mg kg-1 fresh weight for fruit vegetables, and 0.1 and 0.3 mg kg-1 fresh weight for leafy vegetables for Cd and Pb, respectively.
ORIGIN OF CONTAMINATION IN PLANTS
The HM in the soil can be geogenic or anthropogenically originated; those of geogenic origin come from the parent material in the earth's crust, from the leaching of mineralization or from volcanic activity (Galán and Romero, 2008; Zwolak et al., 2019). And thus, this type of pollution evolved into a global problem that severely hampered sustainable agricultural production and put at risk environmental safety (Wang et al., 2022).
The WHO (2020) points out that the existence of HM in the food chain is caused especially by air, water and soil contamination. Aubry and Manouchehri (2019) characterize the factors of exposure of urban agriculture to pollution with respect to the type of crop, the location of the crops, and the characteristics of the pollutant and the soil.
Antisari et al. (2015) report that pollutants in urban areas are commonly from anthropogenic sources, such as road traffic, prior industrial use of the lots, incinerators, and atmospheric deposition caused by industrial activities. In their study on spatial distribution of Pb in peri-urban soils Wu et al. (2023) found that Pb concentration was elevated in sites near industrial firms (<4 km) and gasoline stations (<1.5 km).
HM sources that generate contamination during the production of horticultural crops are grouped by Li et al. (2018), Rai et al. (2019) and Thakali and MacRae (2021) as:
- Proximity to industrial areas, mining, and atmospheric deposition
- Automobile sources on highly trafficked roads that contaminate neighboring crops
- Industrial and road runoff
- Irrigation with wastewater from urbanized and/or industrial areas
- Excessive application of fertilizers, especially phosphoric types
- Livestock manure
- Sewage sludge-based amendments
- Application of metal-based pesticides
Marschner (2012) adds to these sources of HM high soil-plant transfer, especially to the harvest organ.
Air
Polluted air is one of the most important issues that affects the health and well-being of the urban population (Fischer and Beltrán, 2021). Urban areas have a substantial impact on air quality at all levels (Folberth et al., 2015). Airborne particles from anthropogenic sources usually transport trace elements (such as HM), salts (sulphates and nitrates) and organic compounds adsorbed on their surface (Izquierdo-Díaz et al., 2023). Due to their ability to associate with air masses, HM are recirculated by winds, depositing in areas far from their origin, either on the ground or on plants in urban and peri-urban areas (Imperato et al. 2003; Ferreira et al., 2018a). Cleaner air alone could provide up to 20% yield increase in important crops such as soybean and corn (Lobell and Burney, 2021). Augustsson et al. (2023a) found in a farm in southeast Sweden that leafy vegetables continue to hold 0.05-1.3 wt% of adhered particles after washing and, through ingesting 0.5% of these particles, considering a mean intake of vegetable food, an adult person could consume 100 mg of particles daily.
The level of pollutants decreases not only with distance from main roads but also with height, as Aubry and Manouchehri (2019) report for the city of Paris, in which pollution is markedly reduced at the fourth floor of buildings, making vegetables grown at these heights much more innocuous. Ercilla-Montserrat et al. (2018) proved the feasibility of growing leafy vegetables on the rooftops of Barcelona and its surroundings using soilless systems in high-traffic areas, with low HM contents in edible parts of lettuce.
Most metals, such as Cd, Cu, Pb, Zn and Ni, are 75-90% bound to the PM10 fraction of particulate matter (Mohanraj et al., 2004).
In neighborhoods with a high population and few green areas in Bogota, such as Kennedy (960,797 inhabitants and 80 ha of tree cover), Fischer and Beltrán (2021) report a much higher concentration of PM10 (43.7 μg m-3) than in the greener Usaquen (499,829 inhabitants and 120 ha of tree cover), with 24.7 μg m-3 PM10, for the year 2019. Kumar et al. (2019) highlight the potential of urban trees to decontaminate the air due to the deposition of particulate matter on their leaves.
In a study in cities with high vehicular activity in the state of Zulia in Venezuela, concentrations of Pb and Ni were measured that exceeded the permissible levels of the WHO (Machado et al., 2008). Meanwhile, in the city of Manizales (Colombia), the concentrations of Hg, Ni, Cr, Pb and Cd did not exceed the limit values established by the WHO and the EPA (Velasco, 2005). In the highly industrialized area of Bogota, Puente Aranda, the main metals in the atmosphere were Fe and Pb (≤4,000 ng m-3), while intermediate concentrations were measured for Cr, Cu, Ni, Zn, and Mn (50-700 ng m-3) and the lowest levels were found for Cd and Ag (Pachón and Sarmiento, 2008).
In Bologna (Italy), high HM concentrations (160 mg kg-1 dry weight [DW]) were measured near the road in lettuce, while 210 mg kg-1 DW was found in basil (Antisari et al., 2015). In this same study, comparing the accumulation of HM in tomato and zucchini fruits grown 10 or 60 m away from the main road, significantly higher concentrations of Cd, Cr, Ni and Zn were found in tomatoes and in zucchini of Ba, Ni, Sn and Zn at 10 m from the road, compared to plants of the same two species at a distance of 60 m.
Säumel et al. (2012) found in Berlin (Germany) that the aerial deposition of HM is high within the city, meaning that consumption of vegetables grown in the city, especially those grown near roads, would mean a high risk of exposure to toxic metals. Due to this situation, Säumel et al. (2012) highlight the importance of barriers, including buildings, between crop plots and roads, which reduce crop contamination. Likewise, ornamental trees in the city contribute to the reduction of air pollution due to the deposition of particulate matter (including HM) on their leaves, highlighting the importance of their proximity to urban vegetable crops (Antisari et al., 2015; Fischer and Beltrán, 2021). Sharma et al. (2009) point to the danger of contamination by atmospheric deposition during the production, transport and sale of products in urban areas. These authors found a higher concentration of Cd, Cu and Zn in vegetables in marketplaces in Varanasi (India) than in production sites, especially during the months of November to February.
Water
There are many differences in the susceptibility of different types of plants to air and soil contaminants, considering that irrigation water can contaminate both the soil and the plant (Miranda et al., 2008b; Aubry and Manouchehri, 2019). The United Nations (2019) stated that water and food security are of global interest and crucial to the achievement of sustainable development objectives. In urban areas and, particularly, in peri-urban areas, where rivers are used to irrigate vegetables, contamination can be high, as is the case of the Bogota River (Miranda et al., 2008a; Bello and Lesmes, 2011) or the Atoyac River (Puebla, Mexico) (Mora et al., 2021). Various studies found that the Bogota River contained toxic elements such as heavy metals and metalloids, for example, cadmium (Cd), arsenic (As), cobalt (Co), mercury (Hg), chromium (Cr), lead (Pb), tin (Sn), iron (Fe), copper (Cu), manganese (Mn), nickel (Ni) and zinc (Zn), the last five being essential elements for the development of plants, in very low concentrations (Miranda et al., 2008a).
Municipal and industrial wastewater are an important source of HM that contaminate rivers and thus irrigation water for neighboring crops. Zhao et al. (2022) classified municipal wastewater as the primary source of wastewater employed directly in vegetable crops. The use of treated or untreated wastewater for irrigation to relieve the deficit of freshwater resources has been very common in developing countries (Aftab et al., 2023; Ruan et al., 2023; Yahaya et al., 2023). This situation is further aggravated if there are not enough wastewater treatment plants along the waterway, as is the case for the Bogota River (Díaz-Martínez and Granada-Torres, 2018).
Irrigation with wastewater that has contaminated rivers leads to significant contamination with toxic metals in soils and in vegetables grown in these sites (Zwolak et al., 2019) as well as with fecal coliforms (Ferreira et al., 2018a). Aubry and Manouchehri (2019) attribute the risks to food grown in the urban area mainly to bacteriological causes due to the use of wastewater by residents and, in addition, to crops located in low-lying areas prone to flooding.
Corrales et al. (2018) found that irrigation water taken from the middle basin of the Bogota River contained bacteria harmful to humans such as Escherichia coli, Proteus vulgaris, Serratia marcenscens, Brevibacillus cereus and Enterobacter cloaceae, as well as bacteria phytopathogenic for crops such as Aspergillus fumigatus, A. flavus, Rhizopus sp., Mucor sp., Fusarium sp. and Penicillium sp. A tomato crop irrigated with industrial wastewater produced the same yield as plants irrigated with clean groundwater, but with lower marketable fruit yields and with a fecal E. coli and Enterococci load that exceeded allowable limits (Gatta et al., 2015).
Rai et al. (2019) concluded that irrigation with insufficiently treated effluent or sludge represents the primary origin of contamination for vegetable crops in developing countries. In sewage sludge, the most commonly detected HM are Cr, Fe, Mn, Ni, Co, Cu, Hg, Zn, Pb, Cd, As and Se (Buta et al., 2021). Irrigation with reclaimed water has a much lower pollution risk than untreated wastewater and, moreover, regenerated water contains organic matter and essential nutrients (N, P, K, among others) that can lead to optimal plant growth (Zhao et al., 2022). Panhwar et al. (2022), based on their experience using treated wastewater for growing healthy okra crops, suggest that reclaimed industrial wastewater could be an important and useful source for agricultural objectives, which would reduce the discharge of freshwater resources and contribute to protecting the environment.
Several studies in plantations near the Bogota River that measured the concentration of these elements in the river water and the soil, and vegetables irrigated with it found that the concentration of the pollutants in the water and soil were still within the level allowed by international standards (Miranda et al., 2008a). However, in the case of lettuce and celery plants, Cd concentrations exceeded the maximum limit allowed by the European Union (EU), and in the four vegetables in the study (lettuce, celery, cabbage and broccoli) the amount of Pb exceeded the maximum level permitted by the EU in foods for young children including infants (Miranda et al., 2008a). Similar results, exceeding the European norm, were recorded by Bello and Lesmes (2011) in chard and lettuce irrigated with water from the Bogota River, and chard exceeded the permissible limit for Cr, while in celery no contamination by Cd, Pb, Cr or As was found, as observed by the authors.
As well as industrial activities, technified agricultural production, especially in the vicinity of rivers, can produce high amounts of HM that will continue to accumulate in the river water, contaminating it (Yu et al., 2022). To avoid disasters due to flooding and waterlogging through rivers (with little pollution), the development of agricultural irrigation is one of the most sustainable solutions (Yu et al., 2022).
Soil
Previously, water and air pollution were considered more important than soil pollution. Recently, however, soil contamination has become severe, and now attracts global attention given the implications of this for the safety of agricultural products (Shukla and Jain, 2022).
In general, urban soils have been moved, leveled and/or compacted over the years (González et al., 2021), and therefore vary widely in their physical-chemical characteristics (Meftaul et al., 2020). They stand out for their low level of organic matter and, in addition, high pollutant content, normally due to air deposition (Pavao-Zuckerman, 2008). Ferreira et al. (2018a) mention that soil pollution is one of the greatest risks of urban agriculture, while Kabata-Pendias (2004) and Haider et al. (2021) attribute the soil as the main sink for pollutants, especially HM for plants.Contamination of soil by HM and pesticides leads to loss of fertile land and endangers vegetable production and quality (Naidu et al., 2021).
Contamination of urban soils results from vehicle emissions, industrial processes, application of herbicides and pesticides, and inappropriate waste treatment (Li et al., 2018). In many cases, urban soils contain toxic elements such as HM and polychlorinated biphenyls (PSBs) that can contaminate vegetable and fruit plants produced in urban horticulture, putting human health at risk (Ferreira et al., 2018b). Several studies showed the accumulation of toxic metals in vegetables grown in urban soils (Nabulo et al., 2012). In urban soils in Chengdu (China), the highest HM contamination measured originated from atmospheric deposition and traffic activities in areas of high and medium urban intensity. Cd was the highest pollutant in high intensity areas (Li et al., 2023).
Growing crops in peri-urban areas is an essential contribution to the provision of vegetables to urban residents (Hu et al., 2023). A recent worldwide study by Liu et al. (2023) shows that contamination of peri-urban soils with metal(loid)s, pesticides and microplastics is similar to that of soil in urban green areas, that is, natural peri-urban areas have the same contamination as green areas in the city. Likewise, Kumar et al. (2022) and Li et al. (2019) mentioned that pressures from industry, urban growth, transportation and agriculture led to a decrease in soil quality in peri-urban areas and thus to an accumulation of HM in these soils (Hu et al., 2023).
Lora and Bonilla (2010) warn of the need for remediation of soils adjacent to contaminated rivers due to the medium to high concentrations of Cd and Cr in soil samples between 0 and 20 cm deep in the route of the Bogota River between Villapinzon and Bosa (Cundinamarca).
The availability of HM for plants is largely conditioned by the cation exchange capacity, the clay content, humus and the pH of the soils (Umar et al., 2005). Kabata-Pendias (2004) generalized that in acidic, well-oxygenated (oxidizing) soils, several HP, especially Cd and Zn, are rapidly mobile and available to plants; while in neutral or alkaline soils with poor aeration (reducing), metals would be significantly less available. This implies that acid pH of the soil is the most important factor influencing the high absorption of HM by vegetables; Kabata-Pendias (2000) report that, in alkaline soils (pH 7.1-8.1), HM have a lower bioavailability for plants and the application of organic matter can reduce the absorption of HM from the soil solution.
In waterlogged or flooded soils, plants suffer from hypoxia stress due to the low level of oxygen (Habibi et al., 2023), an increasingly frequent situation due to extreme events of climate change in plantations near rivers or other bodies of water and their surrounding cities. Due to this adversity, the redox potentials decrease, generating an increase in the solubility of nutrients such as Fe2+ and Mn2+, reaching phytotoxic concentrations in the rhizosphere (Marschner, 2012; Fischer et al., 2023); we assume that some non-essential HM can also reach these harmful levels under waterlogging and inundation conditions. For Spartina alterniflora, a species from estuarine wetlands, Xu et al. (2018) observed that the accumulation of Pb, Cr, Zn and Cu in the plant was proportional to the exchangeable fraction of these HM in the sediment, which increased with the time of flooding. In two tomato varieties placed under combined Cd and waterlogging stresses, Zhou et al. (2023) found that synergistic regulation between the antioxidant systems maintained the ROS at a normal level, but that damage from the two stresses to the structure and function of the chloroplasts generated a lower photosynthetic capacity, decreasing the biomass production of the plants.
In general, the use of interior urban spaces for agriculture reduces plant contamination by soil or air; however, contamination by soil increases if it has been used for industrial purposes (Aubry and Manouchehri, 2019). A study in Ohio (USA) confirmed that urban orchards managed for several years contained far fewer contaminants than soils not used for this purpose in the city, which contained higher levels of Pb, Zn, Cd, Cu and polyaromatic hydrocarbon; this was due to microbial activity and degradation as well as to the dilution of HM by mixing with clean soil (Kaiser et al., 2015). However, Säumel et al. (2012) found in the center of Berlin a significantly higher Pb content in vegetable species grown near busy roads than in vegetables sold in supermarkets, most likely coming from outside the city. For contaminated urban soils, Antisari et al. (2015) recommend hydroponic cultivation, for example, in the case of rosemary, as it is a HM accumulator plant.In urban horticulture, soilless cultivation systems can prevent the introduction of HM into vegetable plants because these processes can guarantee unpolluted substrates (Ercilla-Montserrat et al., 2018).
Vega and Vega (2021) examined 49 organically managed urban orchards in the city of Bogota, near high vehicular traffic, finding that the concentration of Pb in soil, irrigation water and the plant did not exceed national and international standards. These authors also mentioned that the total level of Pb in the soil is not always fully available, since absorption, transport and bioaccumulation of this element by plant tissue are related to various variables.
Pesticides and fertilizers
The incidence of pests in crops has increased, for reasons including climate change, which has resulted in an increase in the use of pesticides (Wenning et al., 2010). One of the predominant environmental problems associated with pesticides is infiltration into aquifer complexes (Mora et al., 2021). In small scale crops in the urban environment, owners often inadvertently apply excessive doses of pesticides, leaving residues that constitute a threat to the area (Meftaul et al., 2020). Eijsackers and Maboeta (2023) reported that the heavy metal-containing fungicide copper oxychloride, applied under field conditions, can damage soil life.
Contamination of vegetables results from the excessive use of fertilizers because they can increase the accumulation of HM (Marschner, 2012; Zwolak et al., 2019). Near Bologna (Italy) an accumulation of 1.2 mg kg-1 PS of Cd was found in tomato fruits due to crop fertilization over many years, a higher concentration than in tomatoes from urban agriculture (Antisari et al., 2015). Also, in greenhouse tomato cultivation, Gil et al. (2004) report that, due to the specific use of substrates and fertilizers, in addition to the reuse of water and nutrients, the accumulation of Cd and Pb can increase. However, Wan et al. (2022) compared the soil of 60 greenhouse vegetable plots (5-20 years old) with those of 20 adjacent arable land plots of vegetable production, finding that the Pb and Cr soil concentrations were lower in greenhouses, possibly due to the protection provided by the greenhouse roof, which kept soils away from atmospheric depositions emitted by neighboring industries.
In peri-urban agriculture or in rural areas close to cities, the use of fertilizers and pesticides is generally higher due to the high demand of growing cities (Li et al., 2023).The excessive use of phosphate fertilizer is the primary source of high Cd concentrations in agricultural soils (Niu et al., 2023), which contain Cd concentrations between trace and 27.20 mg kg-1 (Li et al., 2018), while the application of diammonium phosphate (DAP) can increase the mobility of Cd, Ni, Cu and Zn (Jalali and Moharami, 2010). Liming soils can reduce Cd uptake by increasing pH and absorption competition between Ca and Cd, but, conversely, liming can also increase Cd uptake by reducing Zn levels and thus competition for Cd uptake (Marschner, 2012). Meanwhile, the foliar application of “InCa” which activates Ca transport in vegetable species containing among other substances Ca(NO3), decreased root Pb concentration in tomato and cucumber plants to 73% and 60%, respectively (Wierzbicka et al., 2023). In cauliflower genotypes Ma et al. (2022) observed that the application of nitric oxide relieved Cd-induced decrease of biomass and growth. They attributed this positive response mainly to the reduction of Cd uptake, sustaining essential minerals content, increasing the action of antioxidant enzymes and thereby inhibiting ROS content and protecting proteins and photosynthetic pigments.
In organic agriculture, the use of synthetic pesticides and fertilizers, emissions in general and nutrient runoff are reduced by using organic household debris and other available sources (Aubry and Manouchehri, 2019), but this can also decrease crop yields (Weidner et al., 2019). As mentioned before, biological management of plantations is very important around urban and suburban rivers (Yu et al., 2022). Also, in organic production, the natural phosphorous source in fertilization must not exceed 90 mg kg-1 of P2O5 according to the Codex Alimentarius.
EFFECT OF HEAVY METALS ON PLANTS
Heavy metal toxicity impairs the yield potential of many vegetable crops. It hampers physiological functions of plants, decreases seed germination, impedes the photosynthetic functionality, and generates oxidative stress (Elango et al., 2022).
In order for HM to accumulate in plant cells, they must be mobilized in the soil and taken up by the roots, where they are loaded into the xylem to be transported in the stem to the aerial parts of the plant, then distributed to the tissue of the leaves or fruits (DalCorso et al., 2014). For each of these steps, as reported by Clemens (2001), a complex interaction between chelating compounds and metal transporters is needed that affects the rate of accumulation of these metals.
Elango et al. (2022) described a non-linear response of increasing HM absorption, that depended on factors such as the availability and solubility of these elements in the soil, the leaf and the leaf type, fertilization, growing environment, redox potential, soil moisture, and others. Additionally, the latter authors mentioned that factors such as the form in which they are present in the soil, i.e. bound with soil particles or easily available in soil solution, determine the availability of these HM for plant uptake.
In most cases, the roots are damaged first and very severely, because the HM that are absorbed accumulate over time in this organ, which affects the general growth of the plant (Umar et al., 2005), hindering its supply of water and nutrients (Ernst, 2003). In the absorption of metals such as Cd, Xin et al. (2015) found that, in varieties of paprika (Capsicum annuum), the type of efflux of low molecular weight organic acids by the roots determined the difference in the volume of Cd accumulated between varieties with high and low Cd content. It is noteworthy that the translocation of the HM from the roots to the stem affects the senescent leaves more than the young leaves (Ernst, 2003).
According to Marschner (2012), there are still no convincing studies on the beneficial effects of HM such as Cd, Pb, Cr and Hg on higher plants and how they affect their growth and development. On the other hand, there are studies that show some effectiveness in reducing the level of toxic metals in plant species through the symbiosis between plants and fungi that form arbuscular mycorrhizae (AMF) (Aguirre et al., 2011).
In general, increased HM in vegetable crops has several effects on the physiological and biochemical functions of plants (Zulfiqar et al., 2019; Haider et al., 2021) (Tab. 2), which differ according to the phenological state of the plant, the concentration and the type of metal (Epstein and Bloom, 2004). Functional impairments from metal excess are associated with membrane damage, oxygen radical production, inhibition of key enzymes, and inactivation of photosynthesis and the respiration electron transport chain, as well as restriction in the absorption and assimilation of N, the last of which greatly affects growth (Larcher, 2003).
Table 2. Symptoms of toxicity by important HM in plants.
Heavy metal | Critical foliar concentration in plants | Symptoms | Author |
---|---|---|---|
Nickel (Ni) | 10-50 μg g-1 DW | Inhibition of root elongation, reduced stem growth, interveinal chlorosis | Marschner (2012); Kabata-Pendias (2000) |
Copper (Cu) | 20-30 μg g-1 DW | Inhibition of root growth before stem growth, oxidative stress | Marschner (2012); Epstein and Bloom (2004) |
Zinc (Zn) | 100-300 μg g-1 DW | Inhibition of root elongation, chlorosis in young leaves, decreased photosynthesis | Marschner (2012) |
Manganese (Mn) | 200-1380 mg kg-1 DW | Brown spots on mature leaves, interveinal chlorosis and necrosis, deformation of young leaves, loss of apical dominance | Marschner (2012) |
Iron (Fe) | >500 mg kg-1 DW | Bronzing, especially in waterlogged soils | Rengel et al. (2022) |
Cobalt (Co) | 0.4-few mg kg-1 DW | Foliar chlorosis, reduced biomass accumulation and nutrient uptake | Marschner (2012); DalCorso et al. (2014) |
Aluminum (Al) | Inhibition of root elongation, nutrient and water uptake; inhibition of cell division and elongation | DalCorso et al. (2014); Casierra-Posada and Aguilar-Avendaño (2007) | |
Lead (Pb) | Physiological alterations in enzyme activities, water potential, mineral nutrition, hormonal status, electron transport and membrane structure; chlorosis, inhibition of root elongation | García (2006); Miranda et al. (2008b) | |
Mercury (Hg) | 0.5-1 μg g-1 DW | Physiological changes such as aquaporin shutdown, leaf chlorosis, severe stunting of seedlings and roots | Miranda et al. (2008b); Kabata-Pendias (2000) |
Arsenic (As) | Morphological changes in the plant, physiological alterations, decreased yields | Miranda et al. (2008b) | |
Cadmium (Cd) | 5-10 μg g-1 DW | Morphological changes in the plant, atrophied roots, minor root elongation, leaf chlorosis, brown leaf margins, reddish veins, deterioration of xylem tissues, alterations of physiology and of enzymatic activities, reduced plant growth and biomass, alterations of photosynthetic pigments and gaseous exchange, mineral uptake and antioxidative defense machinery. | Miranda et al. (2008b); Kabata-Pendias (2000); Bautista et al. (2013); Ma et al. (2022) |
Chromium (Cr) | 1-2 μg g-1 DW | Foliar chlorosis, reductions in chlorophyll synthesis, CO2 fixation and carbohydrate metabolism, root damage, decreased Ca, K, P, Fe and Mn incorporation caused by Cr6+ | Bello and Lesmes (2011); Lora and Bonilla (2010); Kabata-Pendias (2000) |
DW: dry weight.
Elango et al. (2022) classified impacts of HM toxicity on plants into direct effects, such as reduced mineral uptake, photosynthesis, respiration, protein content, seed germination and plant biomass; and indirect effects, such as damage to lipids and DNA, generation of ROS, chromosomal abnormalities, cell injury or chlorosis, damage to cell membranes and disrupted enzyme activity.
In general, an overload of HM in the soil means a greater stress for crops than a deficiency of these elements, although that some plant species can establish a tool to protect themselves against this excess. The more the plant is tolerant of HM, the slower its growth (Epstein and Bloom, 2004). High Ni toxicity, as well as high Al content, can cause interveinal chlorosis due to induced Fe deficiency (Kabata-Pendias, 2000) while, according to Umar et al. (2005) foliar chlorosis due to Pb toxicity could be generated by the susceptibility of some enzymes to Pb ions, affecting chlorophyll biosynthesis.
Kathpalia and Bhatla (2018) describe hyperaccumulators or metallophytes as plants that can absorb very high concentrations of HM from the soil and store them in their aerial tissues at levels between 100 to 1,000 times higher than a non-hyperaccumulator plant. An example of this is Brassica, which capable of accumulating up to 30,000 μg g-1 of Zn and 1,300 μg g-1 of Cd in its tissues. One of the reasons for this very high translocation of metals to the aerial part is the overexpression of HMA proteins (heavy metal-transporting ATPase) loading the HM to the xylem of these plants (Kathpalia and Bhatla, 2018). According to Lambers and Oliveira (2019), there is evidence that the hyperaccumulation of these metals increases defense against microbial and herbivorous pathogens.
The readiness with which the following HM are bioaccumulated by plants is in the order as follows (a) from an aquatic environment: Hg, Cd, Pb, Cu, Zn, Sr and (b) from soil: Cd, Zn, B, Ni, Sn, Cs, Rb (Kabata-Pendias, 2004). There is much variation in the concentrations of HM in the edible part of the vegetables; the ability to take up and accumulate HM was highest in leafy vegetables and lowest in cucurbitaceous vegetables (Zhou et al., 2016). For groups of plant species that accumulate Cd, Yang et al. (2009) established the following increasing order: legumes vegetables < melon vegetables < alliums vegetables < root vegetables < solanaceous vegetables < leafy vegetables (Fig. 1). The higher accumulation in HM concentrations by leafy vegetables is explained by their higher translocation and transpiration rates compared to fruit vegetables (Yadav, 2021).
Pb contamination does not greatly affect the eating quality of fruits but does harm it in some leafy vegetables such as lettuce, spinach, and cabbage due to the greater exposure of their leaf area to atmospheric particles. Root vegetables (radish, carrot, beetroot, etc.) are more susceptible to accumulating HM in large quantities compared to those that develop fruits (paprika, tomato, aubergine, etc.) and are not recommended for planting in contaminated soils (Aubry and Manouchehri, 2019; Zwolak et al., 2019).
Aromatic herbs, such as parsley, are highly exposed to contamination by air and soil alike, (Aubry and Manouchehri, 2019) (Fig. 2). Urban and peri-urban growers should consider that a plant that develops for a long time absorbs more pollutants from the soil, water and air, as is the case with thyme, compared to basil (Aubry and Manouchehri, 2019).
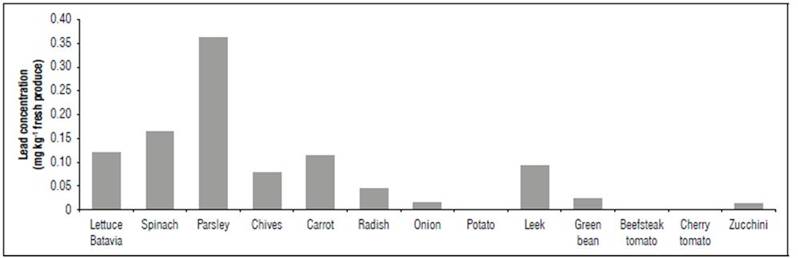
Taken from Aubry and Manouchehri (2019), with permission
Figure 2. Example of results of lead (Pb) concentrations in vegetables growing in the city of Montreuil, near Paris.
There are physicochemical similarities between the different ions, which is why the transport proteins in the plasmatic membrane of root cells are not capable of efficiently differentiating between Ca2+ and Ba2+, K+ and Rb+ or between phosphate and arsenate (Marschner, 2012). For this reason, many toxic ions cannot be excluded in the symplasm of the roots, allowing HM to enter plants and thus the food chain. A special case is Cr, which is essential for humans as Cr3+ as it promotes the action of insulin. Derivatives of Cr6+ (chromates and dichromates) are generally of anthropogenic origin, which at excessive levels produce toxicity in plants (Tab. 2) (Lora and Bonilla, 2010).Among the leafy vegetables, lettuce has been shown to be the most vulnerable to Cr6+ pollution (Yu et al., 2023).
Cd can be easily absorbed by roots and leaves (Kabata-Pendias, 2000; Bautista et al., 2013). In addition, these authors reported that root vegetables as a group are relatively highly susceptible to the accumulation of Cd. Lundgren et al. (2023) developed regression models to predict the uptake of the highly mobile Cd and the less mobile Pb in lettuce, which suggest that Cd uptake is predominantly via roots, in contrast to the physical contamination of Pb, which is primarily in the leaves.
Plants show specific mechanisms to take up, translocate and accumulate HM. Several metals and metalloids that are not essential for plants are absorbed, translocated and accumulated by electrochemical behavior similar to that of essential nutrients (Miranda et al., 2008a), competing, in many cases, with the essential elements of the active side of the enzyme (Umar et al., 2005). The toxicity of HM in plants, according to Tadeo and Gómez-Cadenas (2008), occurs mainly in acid soils, altering growth and the appearance of lateral and secondary roots.
Some vegetables have mechanisms through which they accumulate more HM in the roots than in the aerial part, as is the case for tomato and eggplant with Cd (and several other HM). Thus, a study in Egypt of soils contaminated with HM (Tab. 3) showed that tomatoes accumulated 3.27 and 2.62 mg kg-1 Cd in dry weight of root and stem+leaves, respectively, whereas eggplant accumulated 2.56 and 0.67 mg kg-1 in root and stem, respectively (Hamad et al., 2021), significantly exceeding the level allowed by the EU and the FAO (0.2 mg kg-1 dry weight).
Table 3. Results of some studies in different countries on heavy metals in harmful concentrations in the edible part of the plant, found in vegetables of urban and peri-urban production.
Analyzed elements | Study and country | HM exceeding international standards | Authors |
---|---|---|---|
Cd, Pb, Cr, Cu, Zn, Ni | Analysis on tomato, bean, carrot, potato, kohlrabi, white cabbage, parsley, chard, basil, mint, thyme within the inner-city neighborhoods of Berlin, Germany | Pb in 52% of all samples (EU standard). >50% of the carrot, potato, chard, mint, thyme and parsley samples exceeded EU standards. <33% of the tomato, bean, kohlrabi, and white cabbage samples, but no basil sample, exceeded these values | Säumel et al. (2012) |
Cd, Pb, Cr, Co, Ni, Mn | Irrigation of pumpkin, spinach, cauliflower, luffa and coriander with water from the Chenab River, Pakistan | Cd in all species; Cr in coriander, luffa and cauliflower; Pb in cauliflower (FAO standard) | Aftab et al. (2023) |
Cd, Pb, Ni | Analysis of leek, cress, parsley, basil, mint, chives and radish in three supermarket brands in Tehran, Iran | Cd and Pb in all vegetables and herbs (FAO/WHO and Codex Alim. standards) | Mostafidi et al. (2021) |
Cd, Pb, Cu, Zn | Analysis of spinach, okra and cauliflower in production and markets in Varanasi, India | Cd and Pb in the three vegetables in production and in the market (EU standard). Cd, Zn and Cu in the three vegetables on markets (PFA1 standard) | Sharma et al. (2009) |
Cd, Pb, Hg, As, Cr | Analysis of the groups of bulb, stalk, stem, cabbage and brassica vegetables, leafy vegetables, fruiting solanaceous vegetables, legumes, root and tuber vegetables, fruiting cucurbits and aquatic vegetables in 21 cities in Guangdong province, China | Cd (2.14%), Pb (1.61%), Hg (0.55%) and Cr (0.48%) of all samples (MACs2 standard) | Liang et al. (2018) |
Cd, Pb, Cr, Cu, Zn, Ni, Fe, Mn | Analysis of lettuce, eggplant, cucumber, tomato, pepper, pumpkin, mint, and dill near effluent from a wastewater treatment plant inEgypt | Cd in tomato and eggplant (FAO standard) | Hamad etal. (2021) |
Cd, Pb, Cr, Cu | Irrigation of potato, tomato, onion, cabbage, carrot, beetroot and lettuce with lake and river water, near Bahir Dar town, northwestern Ethiopia | Pb in carrot, potato and beetroot and Cd in carrot (FAO/WHO standard) | Asrade and Ketema (2023) |
Cd, Pb, Cr Cu, Zn | Irrigation of spinach and kale with water from two rivers near Machakos, Kenya | Pb, Cr and Zn in spinach; Cr and Zn in kale (WHO standard) | Tomno et al. (2020) |
Cd, Pb, Hg, As | Irrigation of lettuce, broccoli, cabbage and celery with water from the Bogotá River, Colombia | Cd in lettuce and celery, 74 days after transplanting (EU standard) | Miranda et al. (2008a) |
Cd, Pb, As, Cr, Co, Zn, Ni | Analysis of carrot, artichoke and parsley in crops near the middle basin of the Bogotá River, in Sibate, Colombia | Cd and Pb in the three vegetables (EU and Colombian standards). High concentrations in the other HM as well | Herrera and Lizarazo (2018) |
1PFA, prevention of Food Adulteration Act (New Delhi) with 30, 50, 1.5 and 2.5 µg g-1 for Cu, Zn, Cd and Pb, respectively; 2MACs, maximum allowable concentrations established by the Chinese government.
The toxic concentration depends greatly on the species and variety (Marschner, 2012). Margenat et al. (2019), comparing trace elements (TE) in tomato, lettuce, cauliflower and broad beans growing in peri-urban areas of Barcelona with vegetables from more widely distanced rural vegetable fields, found that the occurrence of chemical contaminants in vegetables depended more on the commodity rather than whether the location was peri-urban or rural. Tomato fruits contained the highest concentration of TE.
Given that HM affects not only the physiology, development and quality, but also the safety and health risks of vegetables, the results of studies from countries of four continents on these contaminants and the permissible HM levels in the tissue of the vegetables are shown below (Tab. 3). The different levels in the same species can be explained mainly by the different sources of contamination, according to the study area, plant age and season of the year (Miranda et al., 2008a; Liang et al., 2018). As international and many national standards include, in most cases, only Cd and Pb in the different types of vegetables, these two toxic metals are largely referred to in table 3.
Hamad et al. (2021) found that the transfer factor (the relationship between the concentration of HM in the aerial part and in the roots that coincides with the percentage translocated from the roots to the aerial part) was, in the case of paprika, greater than 1 (because of its high accumulation) for Cu, Fe and Zn; interestingly, this species did not absorb Cd and Pb. In the other species analyzed by Hamad et al. (2021) (Tab. 3), the transfer factor for the HM was less than 1. In the case of Pb, Kabata-Pendias (2000) reported that only 3% of the Pb is translocated from the roots to the stem.
Begum et al. (2019) studied spinach in contaminated soil near the Buriganga river in Dhaka (Bangladesh), evaluating the transfer factors from the soil to the roots and from the roots to the leaves (Tab. 4). They found that the transfer from the soil to the root was highest for Cr and from the root to the leaves highest for Cd.
Table 4. Transfer factors (TF) of HM from soil to roots and from roots to leaves of spinach.
TF | Cd | Pb | Cr | Cu | Ni | Fe | Zn |
---|---|---|---|---|---|---|---|
Soil-roots | 0.19 | 0.17 | 0.35 | 0.04 | 0.02 | 0.04 | 0.63 |
Roots-leaves | 0.82 | 0.87 | 0.34 | 0.96 | 0.79 | 0.36 | 0.37 |
Source: data taken from Begum et al. (2019).
Higher transfer factors for Cd from the soil to the harvestable part of spinach and kale, 2.23 and 4.10, respectively, were found by Tomno et al. (2020) in crops irrigated from two rivers near the town of Machakos in Kenya (Tab. 4). In comparison, Herrera and Lizarazo (2018) found, in parsley grown near the Bogota River, transfer factors from the soil to the plant of 0.23 for Cd, 0.11 for Pb, 0.14 for Cr and 0.11 for As; and for carrot in the same location, transfer factors of 0.23 for Cd, 0.08 for Pb, 0.05 for Cr and 0.08 for As.
Since grafting could alleviate the toxicity of HM to the aboveground parts to some degree (Savvas et al., 2013) the grafting of melon on more HM resistant pumpkin (Cucurbita moschata) rootstock can reduce contamination of melon fruits. Guo et al. (2023) observed that the ZF1 pumpkin hybrid rootstock had a Cd accumulation ability of roots higher than that of MB3 and FB1 parents and the transfer coefficient of the shoot was lower, which decreased the Cd concentration in leaves, increased the photosynthetic assimilation of leaves and Cd tolerance of these seedlings, and augmented the antioxidant enzyme activities (POD, SOD, CAT).
Added to the factors mentioned above that affect contamination of the soil and the plant by HM, is the influence of climatic factors, such as wind that transports atmospheric pollutants (Fischer and Beltrán, 2021), and rain that drops these toxic substances to the surface and leaches them to deeper depths or causes their runoff to other lands and crops. In extreme cases, heavy rains and storms can cause chemical contamination in surrounding water bodies and watersheds (Noyes et al., 2009) from flooding that distribute HM over wider terrain. In addition, atmospheric humidity conditions contribute to the absorption and translocation of HM (as with any other essential nutrient) within the plant, due to the upward flow of transpiration, especially for plants with larger leaf area (Marschner, 2012). In general, drier conditions and higher temperatures generate greater contamination by air pollution (Emekwuru and Ejohwomu, 2023).
CONCLUSIONS
For the rapidly growing population of cities, urban and peri-urban horticulture is an important opportunity for the supply of fresh vegetables. This type of horticulture is capable of mitigating the effects of climate change by growing crops most adapted to urban and peri-urban conditions. However, on the other hand, growing urbanization and industrialization increase environmental pollution in the cities and surrounding lands.
In nature, HM are widely distributed; when they progressively enter the soil-plant-consumer continuum, they can no longer be easily removed from the system, accumulating at toxic levels.
Apart from an excessive accumulation of the essential HM for the plant (Fe, Zn, Cu, Ni), the most toxic for consumers are Cd, Pb, Hg, Cr and As. HM such as Cd, Pb and Hg cause, neurological and kidney damage as well as cancer and other adverse effects on health, generating even more damage when ingested throughout prenatal development and childhood, leading to irreversible alterations in the central nervous system.
HM can come from air, water and soil. Air is most highly polluted near busy roads and industry, while polluted irrigation water comes from industry and wastewater. Polluted soil arises from contaminated irrigation water, vehicle emissions, industrial processes, application of pesticides and fertilizers containing HM.
High contamination of plants occurs in particular from the accumulation of HM in their tissues, with differences between the absorption and retention of HM in the roots, depending on species and variety.
Plant poisoning causes, most significantly, decreased root growth and plant biomass and chlorosis, among other physiological alterations. Leafy vegetables (including aromatic herbs) and Solanaceae accumulate the most HM, while cucurbits and legumes are the vegetables that least accumulate HM.
Examples from four different continents show high contamination with HM, especially Cd and Pb, of vegetables grown in urban and peri-urban areas. There are still no international standards from the EU, FAO and WHO for the other HM (Hg, Cr, As) in fresh vegetables, only in other foods or in processed form.
In many studies, irrigation water and soils do not exceed the permissible HM concentration but, due to the accumulation in crops with a longer duration, HM content in the plant can exceed these permissible standards.It is suggested that reclaimed industrial wastewater could be a significant and useful source for irrigation of vegetable crops, which will reduce discharge of freshwater resources and contribute to protecting the environment.
In general, to increase the food safety of urban and peri-urban horticulture, more studies are needed on HM contamination, soil aptitude, risk assessment for ingesting intoxicated vegetables, as well as appropriate instructions for the clean handling of these crops in cities and surrounding areas. Not washing vegetables before consumption alone increases the average daily intake of Pb by 130%.