INTRODUCTION
Physical and chemical mutagens are used within specific doses and concentrations (low in many studies) in plant breeding programs to obtain new varieties with highest yield and desirable characteristics, such as: yield increasing, early maturity, reducing plant height and disease resistance (Chen et al., 2023), as well as high oil and protein content (Pavadai et al., 2010). Ethyl methanesulfonate (EMS) is an alkylating agent that affects DNA (Shamshad et al., 2023), it has a strong impact on seed composition as observed among soybean germplasms, which offers multiple benefits to breed for desired multiple seed phenotypes (Zhou et al., 2019), also EMS was used to develop high yield soybean variety (Yuan et al., 2020). On the other hand, high concentrations of EMS affect negatively, so we have to protect plants from its damages; consequently, that is an example of plant protection from mutagens which may exist in some environments.
Antimutagenic materials are compounds that counteract the mutagenic effect, by reducing or eliminating the mutations induced by harmful substances. Therefore, it is necessary to understand mutagen mechanisms to better understand the antimutagenic action (Słoczyńska et al., 2014).
In 1952, Novick and Szilard introduced the term "Antimutagens" to describe agents that can reduce the rate or frequency of induced or spontaneous mutations which are undesirable in certain biological experiments such as somaclonal variation in tissue culture (Novick and Szilard, 1952; Duta-Cornescu et al., 2023). Two basic types of antimutagenic substances can be distinguished: Desmutagens, which work outside the cell by inactivating the mutagenic substance immediately before it reaches the DNA, and Bioantimutagens, which work inside the cell, they participate in suppressing mutations after DNA damage (Kada et al., 1982).
Antimutagenic compounds have different possible modes of action, such as: direct chemical interaction with a mutagen, effect on the enzymes involved in the metabolism of mutagens, rapid elimination of mutagenic compounds from the cells before the induction of DNA damage, modulation of DNA-repairing enzymes (Słoczyńska et al., 2014). Phenolic compounds have an antimutagenic action with both extracellular and intracellular mechanisms (De Flora, 1988; Marnewick et al., 2000; De Flora et al., 2001). Phenolic substances play a nucleophilic role by removing electrophilic mutagens. Phenols can bind or insert to cell membrane transporters, blocking the mutagens from reaching the cytoplasm (Hour et al., 1999).
Many evidence suggest that compounds with antioxidant properties can remove reactive oxygen species (ROS) before the interaction of these molecules with DNA and causing mutations (Lee, 2023).
Antimutagenic action could be attributed to the antioxidant activity characterizing several compounds. Therefore, it is important to study the antimutagenic properties of any compound possessing antioxidant activity (Collins et al., 2012). Antimutagenic substances could be synthetic (like organoselenium and bichalcophenes) or natural (like phenolic compounds) (Słoczyńska et al., 2014; Gautam et al., 2016).
Many plants were studied to determine their antimutagenic activity (usually on modified bacteria by Ames Test), such as: Solanum lycopersicum L., Hypericum lydium Boiss. and flos inulae (Inula japonica Thunb.) (Huang et al., 2013; Valdez-Morales et al., 2014; Boran and Ugur, 2017). Antimutagenic substances were tested on microbes such as bacteria (Ames test), yeasts and other organisms (Słoczyńska et al., 2014; Asdaq et al., 2021). The main objective of using antimutagenic substances is to protect (or improve) the characteristics of the organism in the presence of a mutagen. Nevertheless, studies testing antimutagenic substances directly on mutant plants (with EMS) to preserve their physiological, morphological and productivity traits are almost rare or doesn't exist.
Citrus is one of the most widespread genera in the world, especially in tropical and subtropical regions (Kamal et al., 2011). Many Citrus varieties grow in various countries, such as South Asia (Rashid et al., 2013), most of them exist in coastal areas (UNCTAD, 2004). Global production of orange Citrus sinensis L. (other scientific name Citrus × aurantium var. sinensis) was about 47.8 million tons in 2022 (USDA, 2023).
Citrus fruits contain flavonoids, coumarins, limonoids and ascorbic acid (vitamin C), considered as antioxidant, antimutagenic, anticancer and antiallergic materials (Brezo-Borjan et al., 2023; Saleem et al., 2023), especially: naringin, gentisic acid, tangeretin, nobiletin, hesperidin, bergamottin, wakayamalimonol E, wakayamalimonol A-D and limonoxime (Calomme et al., 1996; Olguín-Reyes et al., 2012; Entezari and Ostadzadeh, 2014; Cavalcante et al., 2018; Matsumoto et al., 2017a, 2017b, 2017c; Matsumoto et al., 2018).
Citrus fruits are characterized by a high phenolic content (Sir-Elkhatim et al., 2018), on the other hand, a strong correlation was noted between phenols and antimutagenic activity, this was confirmed by studying the antioxidant activity and antimutagenic activity of 31 plant extracts according to the phenolic content (Makhafola et al., 2016).
The antimutagenic activity of C. sinensis peel extracts obtained by different solvents was studied (Geetha and Santhy, 2013), as well as the methanolic extract of Citrus limonum (ripe Citrus × latifolia or Citrus × limon [L.] Burm. f.) (Mushtaq et al., 2015) and the juice of Citrus nobilis (C. reticulata × sinensis or Citrus × nobilis Lour.) (Entezari and Hosseini, 2014), and essential oil of C. sinensis and C. latifolia (Toscano-Garibay et al., 2017) were also studied.
Encapsulation can be defined as a process of holding active agents (bioactive compounds) within a food-grade carrier/matrix to improve the delivery of these active compounds into food products. The most popular delivery systems among encapsulation techniques are: pickering emulsions, cross-linked polymer gels, complex coacervates, core-shell structure microcapsules and self-assembled structures, those processes enhance the bioavailability and the stability of bioactive compounds (Dahiya et al., 2023), with strong protection, which is required in the delivery of bioactive compounds (Mezerji et al., 2023).
The encapsulating material used in probiotics capsules is classed as macro (>5,000 μm), micro (0.2 to 5,000 μm) and nanocapsules (<0.2 μm), encapsulating material protects the probiotics from environmental stress or harmful environmental factors (Abbas et al., 2023).
The most commonly used as wall materials in encapsulation processes are carbohydrates (maltodextrin, starch, dextran, alginate, chitosan, and gums), gums (arabic, karaya, and xanthan), fibers (pectin and carrageenan), proteins (whey, casein, and gelatin) and waxes (beeswax, carnauba, and candelilla) (Díaz-Montes, 2023).
Nanoantioxidants are materials produced by conversion of antioxidant materials to nanoparticles, and this conversion increases their stability and capacity to scavenge free radicals (Deligiannakis et al., 2012; Khan et al., 2019). On the other side, encapsulation enables active materials to reach some mediums, which are not easily accessible for different reasons (Adefegha et al., 2022; Sun et al., 2023).
Converting phenolic compounds into nanoparticles increases their resistance to dissociation, their thermal stability and antioxidant activity, as well as the possibility of penetrating the cell membrane (Deligiannakis et al., 2012; Medhe et al., 2014; Ghahfarokhi et al., 2016; Phull et al., 2016; Shimizu et al., 2016). Increasing these properties, may also enhance the antimutagenic activity when phenolic compounds are converted into nano dimensions. Besides, the encapsulation of phenols allows their gradual release, which reduces their possible negative effects.
Pectin is a complex polysaccharide mainly consisting of methoxy esterified α, d-1, 4-galacturonic acid units (Cassimjee et al., 2020). This biopolymer has many industrial applications in food, agriculture and medicine. It also participates in important biological functions, such as ensuring cell wall porosity, influencing surface tension, pH regulation, balance, and ion transport through the wall (Chan et al., 2017).
When pectin is used in encapsulation process, it is often used with crosslinking agents such as calcium chloride, because of the electrostatic bonds formed between the negatively charged carboxyl groups of pectin and the positively charged crosslinkers (Gutierrez-Alvarado et al., 2022).
Glycine max (L.) Merr. is one of the ancient crops cultivated by humans. Its original homeland is Southeast Asia. It belongs to Fabaceae family. Soybean is characterized by a rapid life cycle of about 3-4 months and self-pollination, in addition to the presence of a large number of measurable morphological, physiological, chemical and molecular parameters, these advantages make it ideal for mutation studies (Nleya et al., 2019).
Mutations may give positive results, especially if they are directed, but in most cases, results couldn't be predicted. Thus, plants must be protected from unplanned changes, especially by using bioantimutagenic materials.
This research aims to extract polyphenols from orange peels, to measure their antioxidant activity and their capacity to prevent the negative effect of EMS in soybean by studying the persistence of some physiological, morphological and productivity characteristics. Nanoencapsulation of phenols as a protector agent was also studied, to better understand the role of orange peels extract as a protector from EMS damages.
MATERIALS AND METHODS
Fruit sampling
Citrus (sweet orange: C. sinensis) fruits were collected in January 2023 from farms existing in Lattakia and Tartous governorates (areas with a coastal climate) in Syria. Experiments were carried out by using the first layer (flavedo) and the second layer (albedo) of the fruit, without pulp. They were cut into small pieces (1-2 cm2) and used in extraction methods without drying.
Peels percentages and moisture content
The total weight of fruits was measured, then the peels (flavedo and albedo) weight was measured without pulp, the following equation (1) was applied:
A part of the peels was placed in a hot air incubator (JSGI-250DT -JSR, Gongju-City, Korea) at 50°C for 3 d, till a constant dry weight was obtained, moisture content was calculated by the following equation (2):
Extraction methods
Maceration: 50 g of moist peels and 500 mL of distilled water were placed in a glass flask for 48 h (250 mL for every 24 h) on an orbital shaker (JSOS-500 JSR) up to 150 rpm at room temperature (Safdar et al., 2017).
Reflux extraction: carried out by Clevenger apparatus (triangle type), 50 g of moist peels and 500 mL of distilled water for 4 h (Clevenger, 1928).
Reflux assisted extraction: carried out as in reflux extraction method again, but the resulting peels were re-extracted by maceration using distilled water (3:1) v/w for 44 h on orbital shaker 150 rpm at room temperature (total time of extraction is 48 h). The extracted liquid was then collected from each stage and put together.
The extracted liquids resulting from all extraction methods were filtered and centrifuged at 5000 rpm for 5 min by centrifuge BOECO (type 1610-13, Boeco, Hamburg, Germany) to get rid of impurities and concentrated by rotary evaporator (RV 10 digital IKA, Staufen, Germany). Essential oils were also extracted by reflux extraction and reflux assisted extraction, then they were preserved at -20°C for other uses.
Total phenolic content and DPPH assay
Folin-Ciocalteu method was used to determine total phenolic compounds, 1 mL of the sample was combined with 4 mL of 2% Na2CO3 and 4.8 mL of distilled water, then 0.2 mL of 2 M Folin-Ciocalteu reagent (Sigma-Aldrich) was added to the mixture and mixed thoroughly. After incubation for 60 min in the dark, absorbance at 760 nm was measured by a UV-visible spectrophotometer (Optizen, Mecasys-Korea). Total phenolic content was determined as milligrams of gallic acid equivalents per gram of extract (GAE mg g-1 extract) using a standard calibration curve between 0 to 300 ppm (AlHafez el al., 2014). Free radical scavenging activities of extracts on the DPPH radical were measured, 0.2 mL of tested samples at different concentrations (from 0.1 to 5 mg mL-1) was added to 2 mL of DPPH solution (39 μg/100 mL of ethanol). After the mixture was shaken and left at room temperature for 30 min in the dark, the absorbance was measured at 517 nm with a spectrophotometer. The results were compared to ascorbic acid (vitamin C) which was prepared as standard with different concentrations from 0 to 50 mg L-1 in ethanol. The antioxidant activity of the tested samples was calculated by determining the decrease in absorbance at different concentrations by using the following equation (3):
where, A1= the absorbance of the control reaction, A2 = the absorbance in the presence of the sample.
The IC50 value, defined as the amount of antioxidant necessary to decrease the initial DPPH concentration by 50%, was calculated from the results and used for comparison (Azaat el al., 2022).
Preparation of orange peel water extract (OPWE) nanocapsules
Pectin-calcium nanocapsules containing OPWE were synthesized as described by Singh et al. (2018) with some modifications:
Dissolving 0.15 g of crude water extract (resulting from reflux assisted extraction of orange peels) in 50 mL of distilled water.
1.5 g of pectin (Sigma) was dissolved in 50 mL of distilled water using a magnetic stirrer for 24 h at room temperature (until pectin gets swelling).
Pectin solution was put in a stirring device (Orion Sage Auxiliary Dispensing Machine 960, Thermo Fisher Scientific, Bremen, Germany) at 8,000 rpm, then the solution of crude water extract was added by 1,000 µL/10 s, stirring continued 30 min after the last 1,000 µL addition.
The solution of crude water extract in pectin solution was put into an ultrasonic bath (Rocker, Ultra sound cleaner, Soner 206H, New Taipei City, Taiwan) at 50 Hz for 1 min.
100 mL of 1% calcium chloride solution was prepared and added on a magnetic stirrer at 200 rpm.
A solution of crude water extract in pectin was added to the calcium chloride solution by dropping 100 µL successively from 5 cm height, the stirring was continued for 15 min after the last 100 µL addition.
Capsules were filtered and washed with distilled water to remove the residue of pectin and calcium chloride, then dried in the oven until weight stability.
The same steps of nanoencapsulation were repeated, but without adding orange peel water extract for negative control preparation.
Note: when these capsules (encapsulated with pectin and calcium) were used in orange peel extract efficiency against ethyl methanesulfonate damages assay, capsules were added to 150 mL of sodium citrate 0.01 M (to ensure pectin dissolution and phenols releasing).
Encapsulation efficiency (%), encapsulation yield (%) and cumulative release (%) were determined by the following equations (4):
Encapsulated phenols weight was determined by the estimation of total phenols after 150 min from releasing in an alkaline medium (sodium citrate 0.01 M) by Folin-Ciocalteu method, on the other hand, the weight of phenols used in encapsulation was determined in the orange peel water extract before encapsulation process (5).
Materials weight used in encapsulation = capsules weight after drying - encapsulated phenols weight (6).
Cumulative release (%) of orange peels water extract nanocapsules was determined by determination of total phenols which were released in an alkaline medium (sodium citrate 0.01 M) at 30, 60, 90, 120 and 150 min by Folin-Ciocalteu method. Also, DPPH assay was used to determine the free radical scavenging activity of released extracts.
Nanostructure characterization: the atomic force microscope (AFM, Nanosurf easyScan2, Switzerland: tapping mode (Tap190 Al-G), NanoSensors™, Neuchatel, Switzerland) was used to determine the morphology and surface topography, particle sizes, and particle size distribution of OPWE nanocapsules after dilution in water and drying in the open atmosphere at room temperature on a clean glass surface overnight.
Orange peel extract efficiency against ethyl methanesulfonate damages assay
Pre-soaking was firstly conducted for seeds activation, 150 soybean seeds (about 23 g) variety SB-337 (obtained from General Commission for Scientific Agricultural Research, Syria) were added to 10 glass flasks containing 150 mL of distilled water, flasks were put on orbital shaker (JSR, JSOS 500) 150 rpm for 4 h at room temperature. All seeds were washed with distilled water (to remove impurities), then the test was started according to the following steps:
Three concentrations (0.01, 0.1, 1%) (w/v) of orange peels water extract resulting from reflux assisted extraction were prepared in 1,000 mL glass flask by adding 0.015, 0.15, 1.5 g of crude extract to 150 mL of distilled water.
The same three concentrations were prepared again, but with adding 1.05 mL of EMS (Sigma) to get 0.7% (v/v) as a final concentration.
One concentration (0.1%) was tested in encapsulated (by pectin) form by adding capsules obtained from stage ‘Preparation of orange peel water extract (OPWE) nanocapsules’ to 150 mL of sodium citrate 0.01 M, then 1.05 mL of EMS was added to get 0.7% as final concentration, the control of this step contains capsules without orange peels extract, with 0.7% of EMS and 0.01 M of sodium citrate.
One glass flask contains just 150 mL of distilled water.
One glass flask contains 0.7% EMS in 150 mL distilled water.
Activated soybean seeds were added to 10 glass flasks, each containing 150 seeds.
All flasks were put on an orbital shaker, 150 rpm for 4 h at room temperature.
All seeds were washed with distilled water, regarding EMS solutions they were destruction by adding NaOH 4% for 24 h at 25°C (De Méo et al., 1990).
Plant cultivation in pots
Cultivation was carried out between June and October, 2023.
The cultivation was done in pots (50 L) with 5 replicates containing soil with the following properties: pH=7.9, electrical conductivity=0.346 ms/cs, organic matter=3.96%, calcium carbonate=56.28%, total nitrogen=0.198%, available phosphate=107 mg kg-1, available potassium=945 mg kg-1. Physically: sand=57.3%, silt=22.6%, clay=20%. 1 L of 0.5g/L iron was added to each pot.
All pots were put in the cultivation room and distributed in randomized block design with controlled conditions (temperature 30°C, humidity 50%, lighting 6,000 lux), irrigation was at the rate of 3 L of water to each pot every week.
Studied traits
Physiological and morphological traits: germination rate (%), plant height (cm) after 3 weeks (were studied in 70 plants of each concentration), number of leaves per plant after 3 months from germination (were studied in 70 plants of each concentration), anomalies in leaves morphology (high probably due to mutations in genes controlling leaf morphology): color, number of pinnules, pinnules fusion, pinnules shape, presence of wrinkle, presence of mottle (mottles) (number of leaves which studied of each concentration approximately: 500).
Productivity traits: total number of flowers after 40 days from germination (were studied in all germinated plants), number of pods per plant, number of seeds per plant, number of seeds per pod (were studied in 60 plants of each concentration), weight of 100 seeds (g).
Statistical analysis
SPSS V.22 software was used to study the differences between means using One-way ANOVA, P<0.05 values indicate statistically significant differences. Analyses were carried out on extraction yields and chemical properties for all extraction methods. In addition, the differences between the EMS effect and the protective effect of OPWE results were analyzed. All experiments of extraction, total phenolic content, DPPH assay and preparation of nanocapsules were done in triplicates. Regarding the morphological and physiological traits, the number of studied samples was mentioned next to each one in the traits studied section. The results were presented as mean ±standard deviation.
RESULTS AND DISCUSSION
A lot of research indicated that juice constitutes about 50% of the fruit weight, so peels and seeds represent approximately 50%. However, the percentage doesn't reach 50% after removing the third layer and seeds (Ademosun, 2022). The results in table 1 show that peels percentage was not in agreement with those obtained by Ahmad et al. (2016)) who indicated nearly 40%, this is mainly due to the part of peels used in each research, three layers and seeds in their study, two layers (flavedo and albedo) in the experiments.
Table 1. Orange peels properties.
Parameter | Value |
---|---|
Peels (%) | 21.31±1.31 |
Moisture (%) | 63.10±2.66 |
Essential oil yield (%) (g oil/100 g dried peel) | 1.12±0.09 |
The moisture percentage reported by Abdel Wahab et al. (2018) for orange peels ranged between 71 and 72%, indicating a high moisture content, however, was do not align with this percentage, possibly due to differences in fruit maturity, variety, and environmental factors across different locations.
The essential oil yield was 1.1%. This value is in proximity to the 0.8% reported by Abd Elghani et al. (2023). The difference in oil yield could be due to the differences in plant variety, extraction time and the size and thickness of peels.
Moist peels were used in all extraction methods because the water in moist peels facilitates phenols extraction. When peels are dried, all cell components (membranes and organelles) adhere to each other which makes the extraction more difficult (Li et al., 2006).
Results in table 2 showed that, extraction by reflux assisted method (reflux followed by maceration for the same sample) has a significantly higher yield (40.42%) in comparison with the other extraction methods. This could be explained by the mechanism of this method, which initially depends on high temperature, especially during the first step (reflux extraction). Reflux assisted method is the most economical method; because essential oil is extracted (as a by-product) during phenolic compounds extraction (Traversier et al., 2020).
Table 2. Yield and some chemical properties of orange peels water extract (with different methods).
Parameter | Maceration | Reflux | Reflux assisted |
---|---|---|---|
Yield, g/100 g dried peels | 22.54±0.22 | 24.11±0.13 | 40.42±1.72 |
Total phenolic content, GAE mg g-1 extract | 76.3±2.1 | 37.6±1.1 | 23.5±0.9 |
Antioxidant activity (mg mL-1)-vitamin C IC50: 0.0467 mg mL-1 | 1.16±0.03 | 1.40±0.09 | 2.24±0.10 |
Phenols are with big importance due to their effect on bacteria, fungi and other harmful organisms. Extraction by maceration results in a significantly higher phenolic content of 76.3 GAE mg g-1 extract. However, the reflux method yielded a relatively moderate amount of phenolic content (37.6 GAE mg g-1 extract), while the reflux assisted method resulted in a lower amount (23.5 GAE mg g-1 extract). This difference occurred because other compounds, such as carbohydrates, were also extracted due to the longer extraction time and the initial high temperature. As a result, the phenolic content decreased relatively. The results are almost in agreement with that of Sir-Elkhatim et al. (2018) indicating approximately concentration of 40 GAE mg g-1 extract. On the other hand, fixed oils, non-polar pigments and proteins can be sequentially extracted from the resulting peels of all extraction methods (Ramadan et al., 2018).
Antioxidant compounds neutralize free radicals and protect the biological system (Ladaniya, 2023). The antioxidant activity shown in table 2 is in accordance with Park et al. (2014) result, who indicated IC50= 1.3-1.4 mg mL-1, IC50 for vitamin C was 0.0467 mg mL-1 after drawing a linear standard curve. There is a positive correlation between total phenolic content and antioxidant activity (Camacho et al., 2022). On the other hand, there is a strong positive correlation between antioxidant activity and antimutagenicity (Asdaq et al., 2021).
The results of the three extraction methods show that they can be used to extract bioactive compounds (as phenols) from orange peels with notable antioxidant activity, but using reflux assisted extraction method may considered the most economical; it increased the total extraction yield, and has antioxidant activity comparable to other methods. In addition, the essential oil was extracted (as a by-product) which has several applications. So we recommend the use of reflux assisted extraction method due to its many advantages compared to other methods, accordingly it was adopted in orange peel extract efficiency against ethyl methanesulfonate damages assay as a direct extract, and in a nanoencapsulated form.
Encapsulation efficiency was 99.65±0.30%, the higher value of the (EE%) means that the coating material is more capable of protecting the core material, so (EE%) is important to calculate the efficacy of active compound encapsulation (Al-idee et al., 2022). Encapsulation yield (EY%) is the percentage of encapsulates produced over the total weight of materials used in the process. It was 72.54 ±0.21%, there is a positive relation between (EY%) and the total polymer concentration (Saeed et al., 2023).
The cumulative release (%) of orange peels water extract from nanocapsules (measured by total phenols) is shown in figure 1. It is important to analyze the relationship between the concentration of the active compound in the medium and time for two main reasons. Firstly, to understand the gradual release rate of the active compound in the medium, and secondly, to determine the time required for the release of all active compound particles. This relationship is generally affected by several factors: composition, composition ratio, ingredient interactions, preparation methods and the pH of the releasing medium (Rajabi et al., 2019; Herdiana et al., 2022).
Antioxidant activity should be studied to estimate the efficiency of bioactive compounds, and to confirm their resistance during the encapsulation process. There is a relative stability in antioxidant activity before encapsulation (IC50= 2.240 mg mL-1) as shown in table 2, and after encapsulation, IC50= 2.236 mg mL-1, as shown in figure 2.
Topographical properties. The surface detailed topography of OPWE-Pectin-Calcium nanocapsules (which were measured using AFM) was shown in figure 3 proving that pectin was able to successfully form capsules in nanometer dimensions via the ionic gelation method. AFM images showed uniformly distributed structure and nearly spherical shape. The particle size distribution histogram of the nanocapsules infers that the particles are distributed in a very narrow range (Fig. 3), and the mean size dimensions of the nanocapsules ranged from 50 to 60 nm, this size may enhance the cumulative release of bioactive materials (Farahmandghavi et al., 2019).
The size and shape of the nanocapsules formed by ionic gelation of biopolymers could be affected by many factors: the variations in the size and molecular weight of the bioactive compounds, core material concentration and the ratio of the wall materials, and molecular weight (Al-idee et al., 2022).
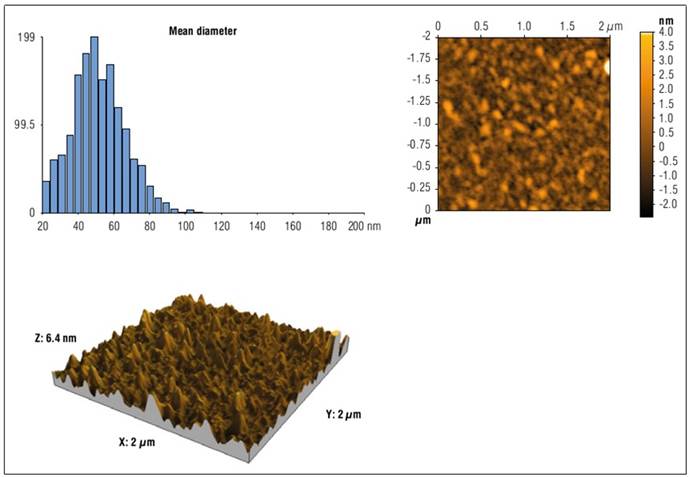
Figure 3. 2 × 2 μm2 (2D and 3D) AFM images and particle size distribution of OPWE-pectin-calcium nanocapsules.
Cultivation was carried out in a cultivation room to avoid the influence of uncontrolled environmental factors (FAO, 2023).
EMS concentrations (from 0.1 to 1%) were studied on soybean, 0.7% gave the highest mutation (damages) rate intersecting with the highest germination rate. On the other hand, using EMS should be less than 1.07% (100 mM) because it is more poisonous at this concentration than mutagenic (Arici and Kara, 2021).
It is important to pre-soak seeds before adding EMS, this process improves the effect of EMS in plants according to the increase of the pre-soaking time (Sağel et al., 2017).
After testing 5 concentrations (0.001, 0.010, 0.100, 1.000 and 2.000%) of orange peels water extract on soybean seeds germination, it was noted that 0.01, 0.10 and 1.00% allowed the best germination rate in petri dishes. On the other hand, phenols concentration in plant extracts used as a protector against EMS should be less than 50 ppm or 0.01-0.001 mol, to avoid phenol poisoning effect on plants as mentioned by Krogmeier and Bremner, (1989) and Colpas et al. (2003). Phenols concentrations in this research were thus 2.35, 23.5 and 235 ppm respectively in peels extract concentrations 0.01, 0.100 and 1.00% prepared in 150 mL of distilled water. For the same reason we prepare nanocapsules in 0.1% which contain referential safe phenols concentration for plants (23.5 ppm).
The importance of studying the morphological and physiological parameters of plants lies in their ability to be clearly measured during the cultivation period. Results in figure 4 showed a significant increase in the germination rate to 93.3% when orange peel water extract (OPWE) was used at 0.01%, however, control plants had a germination rate of 80.7%, it significantly decreased to 75.6% with 1% OPWE. It was noted that, the increase in OPWE concentration to 1% as a protector (with 0.7% EMS) led to a significant increase in germination rate to 64.6% in comparison with 0.7% EMS (51.4%). Using nanocapsules OPWE at 0.1%, showed a significant increase in the germination rate (70.5%) when compared to the control (capsules without OPWE + 0.7% EMS) which was 52.4%. The gradual release of OPWE was more efficient than the whole presence of OPWE in the medium at the same concentration.
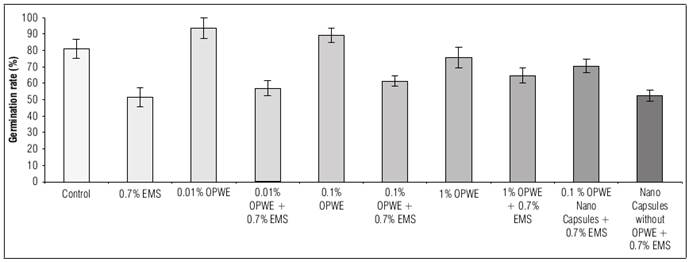
Figure 4. Effect of orange peels water extract (OPWE) on germination rate of soybean seeds, and with EMS.
The results in figure 5 showed a significant increase in plant height (week 2) to 23.9 cm when OPWE was used at 0.01% in comparison with the control which was 21.3 cm, then it decreased to 19.2 cm when OPWE concentration was 1%. However, using this concentration (1%) of OPWE as a protector (with 0.7% EMS) led to a significant increase in plant height to 13.1 cm when compared to 0.7% EMS (9.5 cm). Using nanocapsules (OPWE at 0.1%) showed a significant increase in plant height to 16.7 cm in comparison with the control (capsules without OPWE + 0.7% EMS) which was 9.6 cm.
There are many factors affecting flowering in plants: photoperiod, temperature, insolation and stress (abiotic and biotic). In addition, the lack of supra-optimal levels of nutrients or saturating water levels can also have an impact on the progression towards flowering (Erwin, 2007), so accelerating the flowering process with protector material in the presence of EMS could be positive.
The results in figure 6 showed a significant increase in the number of flowers (after 40 days of germination) to 81 with OPWE at a concentration of 0.01%, in comparison with the control which was 44, then it significantly decreased to 62 with OPWE at 0.1%, then to 39 when OPWE was 1%. When OPWE was used as a protector (with 0.7% EMS), increasing of concentrations to 1% led to a significant increase in flowers number to 35 in comparison with 0.7% EMS (9).
Regarding the use of nanocapsules (OPWE at 0.1%); there was a significant increase in flowers number to 36 when compared to the control (capsules without OPWE + 0.7% EMS) which was 10.
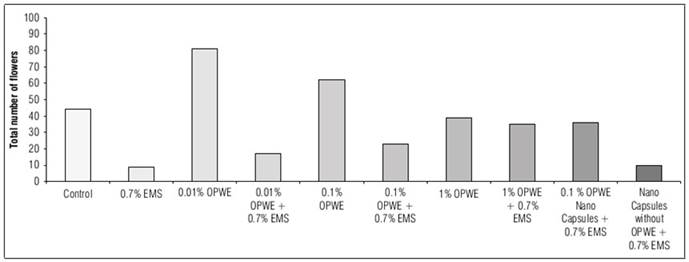
Figure 6. Effect of orange peels water extract (OPWE) on soybean flowers number, and with EMS (in day 40).
Plants leaves number affects the surface exposed to light, and so do plants productivity. The results in figure 7 showed a significant increase in leaves number per plant (after three months of germination) to 9.5 with OPWE at 0.01% in comparison with the control (7.9), then it not significantly decreased to 7.3 with OPWE at 1%. When OPWE was used as a protector (with 0.7% EMS), the increasing in concentration to 1% led to a significant increase in leaves number to 7.7 in comparison with 0.7% EMS (6.9). When nanocapsules (OPWE at 0.1%) were used; a significant increase in leaves number to 7.8 was noted in comparison with its control (capsules without OPWE + 0.7% EMS) which was 7.
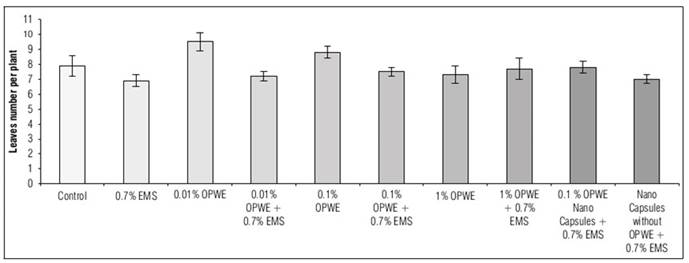
Figure 7. Effect of orange peels water extract (OPWE) on leaves number of soybean, and with EMS (in month 3).
Using OPWE at 0.01% resulted in a statistically insignificant increase in the number of pods per plant, seeds per plant, seeds per pod and 100 seeds weight (g) compared to the control group. The values for these parameters were 7.5, 9.5, 2.2 and 13.8 for OPWE and 6.9, 8.8, 1.5 and 12.7 for the control group, respectively (Fig. 8, 9, 10, and 11). On the other hand, there is a not significant decrease to 6.7, 8.2, 1.1 and 12.3, respectively for 1% OPWE. However, when OPWE was used as a protector at 1% (with 0.7% EMS), a not significant increase in all these traits to 6.6, 6.6, 0.88 and 11.97 was noted respectively, while EMS 0.7% gave less values 5.6, 4.5, 0.64 and 11.2, respectively. On the other hand, there is a significant increase in these traits to 6.5, 7.5, 0.96 and 11.9, respectively with nanocapsules (OPWE at 0.1%), nevertheless using capsules without OPWE + 0.7% EMS (as control) showed less values 5.5, 4.7, 0.62 and 11.1, respectively.
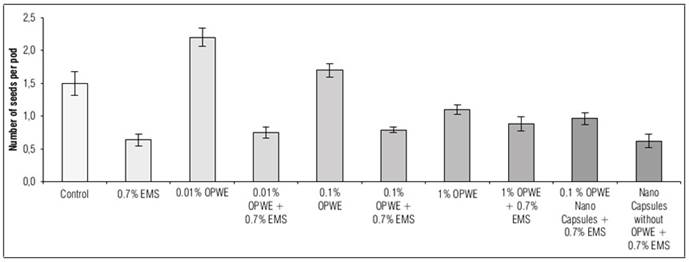
Figure 10. Effect of orange peels water extract (OPWE) on seeds number per pod of Soybean, and with EMS.
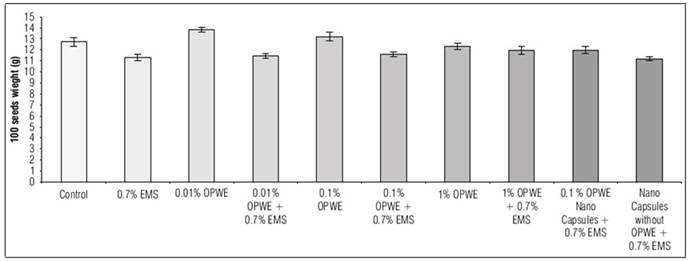
Figure 11. Effect of orange peels water extract (OPWE) on 100 seeds weight (g) of Soybean, and with EMS.
Studying the modifications in leaf morphology (like pinnule shape, number of pinnules per leaf, and leaf color) is the most important indicator of the mutation occurrence (or damages by EMS), due to their sensitivity to stimulators (Hu et al., 2020; Pucci et al., 2021). It was already mentioned by Cooper et al. (2008) and Espina et al. (2018) that EMS makes changes in leaf morphological characters in soybean, as observed in this research. Figure (12) shows some changes in leaf morphology.
The number of morphological changes in leaves (Fig. 13) was very low or completely absent in low concentrations of OPWE. Some anomalies were noted, that could be due to spontaneous mutations or the effect of stress or epigenetic changes. OPWE at 1% showed an increase in negative effect, which could be due to the high content of phenols existing in the extract. The negative effect of phenols could considered as mutagenic effect which was confirmed by many researchers (Zhang et al., 1991; Mbaveng et al., 2014), anyway, it is assumed to be negligible. Using OPWE as a protector (with 0.7% EMS), the number of morphological changes in leaves significantly decreased from (5.1) to (0.8) with the increasing OPWE concentration from 0.01 to 1%. Encapsulated samples of OPWE (0.1%) showed significantly reduced the number of morphological changes in leaves to 0.3 when compared to its control (capsules without OPWE + 0.7% EMS) having 6.1 as the number of morphological changes in leaves.
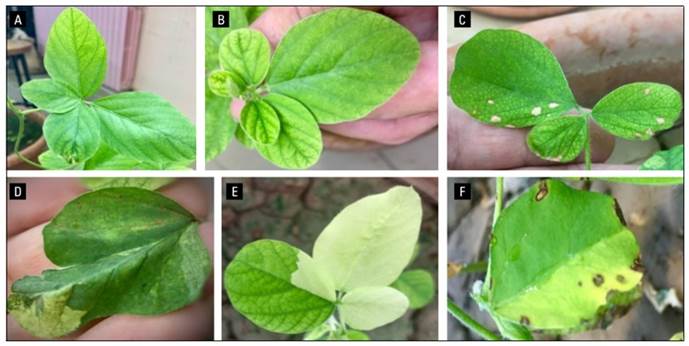
Figure 12. Some morphological changes in leaves (by EMS 0.7%) in soybean. A, tetra-foliate. B, penta-foliate. C, multisize-foliate. D, pinnules fusion. Chlorophyll changes: E, albina and Albina green; F, yellow viridis.
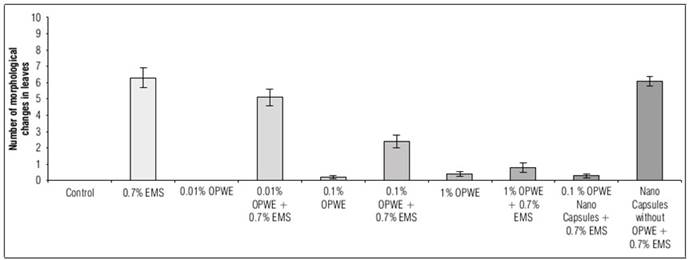
Figure 13. Effect of orange peels water extract (OPWE) on number of morphological changes in leaves per plant of Soybean, and with EMS.
Ethyl methanesulfonate (EMS) is commonly used as an alkylating agent that can randomly induce chemical modifications to nucleotides by introducing active alkyl group, and it results in converting GC pairs into AT pairs (point mutations) (Chen et al., 2023).
The effect of EMS on soybean has been studied by many researchers, and the results revealed in this paper were in accordance with many referenced results. The germination rate decreased to 20% with the increasing concentrations of EMS to 0.32% (Espina et al., 2018), plant height also decreased with the increasing concentrations of EMS to 0.64% (Koshika et al., 2022), however, the number of days from germination to first flower appearance decreased with EMS increasing concentrations (Gopinath and Pavadai, 2015), as well as pods number and seeds yield that decreased also with the increasing concentrations of EMS (Sağel et al., 2017). Leaves mutations number increased with the increasing values of EMS concentrations (Patil et al., 2004). For this reason, high concentration (0.7%) of EMS was used in this research to make a strong effect on plant traits.
A possible hypothesis could be developed regarding the mechanism of action between phenols and EMS reaction: when EMS is dissolved in water, the alkyl group (-CH2CH3) is released from EMS (Elder et al., 2012), and this group acts as an unstable free radical (which causes DNA alkylation and mutations) (Shen, 2019), phenol binds with this group and produces a phenolic radical, which undergoes resonance stabilization (Fernando et al., 2016), by this way phenols could inhibit the mutagenic effect of EMS.
Low concentrations of OPWE (0.01%, 0.1%) were beneficial for soybean, probably due to the presence of phenols (as protector), in addition to the carbohydrates extracted by water (Saïed et al., 2020). A high concentration (1%) of OPWE didn't show positive effects on the plant, except when EMS exists in the medium.
The gradual release of OPWE (0.1%) showed better effectiveness as a protector than the complete release in the medium. It is important to study the cumulative release of OPWE (of phenols) from nanocapsules to verify if the complete amount of phenols was released during the orange peel extract efficiency against ethyl methanesulfonate damages assay time (4 h), to be sure about their coexistence in the medium figure (1). The results indicated that phenols were completely released in the medium after 2.5 h. On the other hand, encapsulating materials (pectin + calcium chloride) had no effect on EMS, as confirmed by the similarity between EMS effects and encapsulation materials (without OPWE) + EMS results. Generally, using OPWE at precise concentrations as a protector substance directly on plant in the presence of EMS could preserve its physiological, morphological and productivity traits.
Our research confirms that it is possible to benefit from orange peels as a source of protective materials against EMS effects, and to use their water extracts to protect plants in some areas containing mutagenic chemicals such as (pesticides, fungicides, food additives, synthetic drugs and pollutants in the atmosphere and water). The protective effect of orange peel water extracts (in well studied concentrations) could be beneficial in tissue culture, where somaclonal variation is not desired, in addition to plant protection in polluted areas.
CONCLUSION
Orange peels contain important phenolic substances that have antioxidant and protective properties in the presence of EMS. High concentrations (0.1 and 1%) of orange peel water extract can protect plants from the effects of EMS. Encapsulating the orange peel water extract increases its effectiveness in protection. Therefore, it is possible to use plants for plant protection from mutagenic substances in some environments and mediums (tissue culture).