Introduction
The successful introduction of new forage species into grassland ecosystems or cultivated pasture systems depends on successful establishment, persistence and forage productivity (Baron and Bélanger 2007). In temperate and subtropical areas, tropical (C4) grasses have the potential to increase forage production during summer, when growing conditions are not ideal for C3 temperate grasses (Davies and McNaughton 1980; Johnston 1996; Crush and Rowarth 2007). As well as having better growth potential, some tropical grasses have shown good adaptation to saline soils (Loch et al. 2004), drought conditions (Pitman 2001), soils with low or high pH (Robinson et al. 1993), infertile soils (Loch 1980), seasonal flooding (Baruch 1994; Imaz et al. 2015a) and other environmental stresses, whereas temperate grasses showed poor persistence (Crush and Rowarth 2007). However, most tropical grasses are seriously adversely affected by winter frost, as a result of sub-zero temperatures (freezing stress). Even at temperatures in the range 0-15 °C (chilling stress) (Ivory 1975; Ludlow 1980; Anderson and Wu 2011), these species show little or no growth (Sage and Pearcy 2000). When suboptimal temperatures are recorded for extended periods (i.e. 2-6 months), significant tissue damage and subsequent plant death can occur (Ludlow 1980; Márquez et al. 2006).
Given the constraints to growth of warm-season grasses imposed by temperate climatic conditions, only those tropical grasses able to tolerate and to survive under winter temperatures, especially minimum temperatures, are likely to become useful. Chloris gayana (Rhodes grass) and P. coloratum (Klein grass) are C4 grasses of African tropical and subtropical origin (Cook et al. 2005), that have been incorporated in pastures in lowland areas of humid grasslands and cultivated pastures in temperate livestock systems (Loch et al. 2004; Tischler and Ocumpaugh 2004; Crush and Rowarth 2007; Imaz et al. 2012, 2015a). Over recent years this introduction has been facilitated by the extended warm summers and less restrictive winters (i.e. higher minimal temperatures and less risk of frost) resulting from global warming (Long 1999; IPCC 2006; Chapman et al. 2012). Both grasses are cultivated in the United States (Texas), Africa, Australia, Japan, South America and under irrigation in the Middle East (Boschma et al. 2008). They are regarded as tolerant of soil salinity and drought (Dear et al. 2008) and grow satisfactorily in areas experiencing flooding (Boschma et al. 2008). Chloris gayana and P. coloratum have a lower critical daily mean temperature threshold for growth (8 °C) than other tropical grasses like Pennisetum ciliare (syn. Cenchrus ciliaris) and Megathyrsus maximus (syn. Panicum maximum).
While C. gayana demonstrates high frost tolerance at plant level and medium tolerance at leaf level, P. coloratum has shown a higher ability to maintain its leaves active (tolerance at leaf level) during winter than other tropical grasses (Ludlow 1980). However, leaves of both grasses are damaged at temperatures below -2 °C to -3 °C, mainly with high air humidity, and plants are killed by temperatures about -10 °C. In this sense, while cold tolerance has a major impact on where the species can grow, grazing management designed to protect plants from the direct physical damage of frost and low temperatures could enhance their utilization. We hypothesize that, while making adequate use of the pasture during the growing season, allowing accumulation of forage in late summer and autumn to provide herbage cover in winter (deferred forage) could help to improve pasture survival in winter and productivity in the following spring.
Thus, the aim of this study was to test this hypothesis by evaluating the effects of autumn-deferred forage as winter cover on growth of P. coloratum and C. gayana in spring under temperate environmental conditions in a pot study. A positive outcome could allow grazing management strategies to be devised to minimize the impact of cold winter temperatures on spring growth. To the best of our knowledge, this is the first attempt to assess the effects of autumn-deferred forage on spring growth of different plant components of these grass species.
Materials and Methods
Experimental details
Chloris gayana (cv. Finecut; Rhodes grass) and Panicum coloratum (cv. Klein) plants were extracted from a commercial beef farm located in Chascomús (35°34'42.9" S, 58°0'49.9" W), Buenos Aires province, Argentina, and transplanted into 15 L plastic pots (1 mature plant per pot) filled with a mixture of sand with top soil (1:2) from a lowland grassland of the Flooding Pampa of Argentina (organic carbon 3.3%; further details in Soriano 1991). There were 10 replicates. Pots were transferred to the experimental garden of INFIVE, the Plant Physiology Institute of La Plata National University, Buenos Aires (34°55'7" S, 57°57'17" W; 45 masl). In order to avoid nutrient limitation, plants were fertilized with di-ammonium phosphate (dose equivalent to 150 kg/ha) 10 days after transplanting. Randomly arranged plants grew outdoors for 4 months from late summer (2 February) until late autumn (23 May), when they were subjected to the following treatments: (i) Control, autumn-deferred forage retained (DF); and (ii) autumn-deferred forage removed (DFR) at 15 cm from top soil. This cutting height corresponds with pasture height at high-intensity grazing or cutting for hay, both of which are common farm practices in late autumn (Chaparro et al. 1995; Sollenberger et al. 2004). All pots were kept at field capacity during the study and plants allowed to grow during winter. In order to promote plant regrowth in early spring, plants were cut again at 15 cm height on 27 September and then allowed to grow during spring. The final harvest was performed when 50% of tillers showed evidence of reproductive structures (Flores et al. 1993), which occurred after 53 (20 November) and 35 (2 November) days for C. gayana and P. coloratum, respectively.
Daily maximum, minimum and mean air temperatures and relative humidity (RH) were registered by a micrometeorological station located in the experimental garden. Daily mean air temperature and RH were used to calculate the air vapor pressure deficit (VPD; Figure 1) in order to characterize the air evaporative demand during the experimental periods, i.e. late autumn, winter and spring.
Biomass responses
Shoot dry matter biomass of initial plants was determined at the beginning of the experiment, when treatments were applied (23 May). At the end of spring growth (20 November and 2 November for Rhodes grass and Klein grass, respectively) plants were harvested by cutting at ground level (final harvest) and biomass determined. Biomass from both early winter (only DFR plants) and spring cuts (both species) was registered and used to calculate the total shoot biomass accumulation, considering both cuts (early winter and spring) and the final harvest. Shoot biomass was separated into upper and lower layers (above and below 15 cm) as recorded in previous grazing studies (Imaz et al. 2015b). Stolons were separated only in C. gayana. Subsequently, shoot biomass was divided into leaf blades and sheaths. There was no root accumulation at the bottom of the pots at the final harvest, which suggested no potential constraints on plant growth due to pot size (Poorter et al. 2012).
Statistical analysis
Shoot biomass data were analyzed separately for each harvest by using Student's T-test (P<0.05).
The relationships between total shoot biomass and: (i) lower layer biomass; (ii) upper layer biomass; (iii)sheath biomass; and (iv) leaf blade biomass, were studied through linear regression using log-transformed data (Poorter and Nagel 2000). Slope tests were carried out to compare these relationships among treatments. Whenever slopes and intercepts among linear adjustments did not differ, data were pooled and a single linear equation was presented. Shoot biomass results are presented as non-transformed means (± standard errors) of 10 replicates. Allometric analysis was used to determine whether shoot biomass differences among plant compartments were due to a size reduction or changes in biomass allocation.
Results
The experimental period extended from late autumn to spring, showing average daily minimum temperature of 7.8 ± 5.6 °C, average daily maximum temperature of 18.5 ± 5.1 °C and daily mean temperature of 12.8 ± 4.8 °C. During the experiment, 13 frost events were recorded, when minimum temperature was lower than 0 °C (between -0.2 and -3.9 °C). The atmospheric evapo-transpirative demand, estimated through the air vapor pressure deficit, gradually increased till spring, ranging from 0.42 to 2.10 kPa (mean of 1.10 kPa; Figure 1). In addition, average daily temperatures by month from 2 February to 23 May were 21.7, 20.1, 16.8 and 12.4 °C for February, March, April and May, respectively.
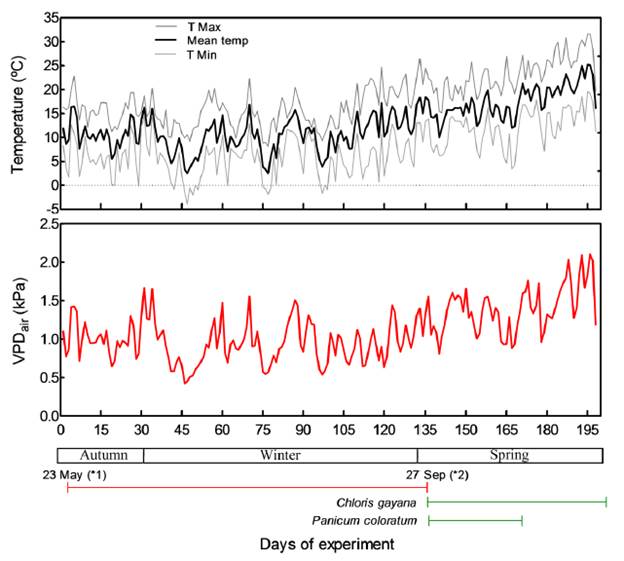
Figure 1. Daily maximum, mean and minimum air temperatures (upper panel) and air vapor pressure deficit (VPD, lower panel) during the study. (*1) Deferred forage cut (autumn); (*2) Early spring cut.
Plants that retained autumn-deferred forage during winter (DF) attained a higher shoot biomass (C. gayana, P<0.01; P. coloratum, P<0.05) following spring growth than plants whose deferred forage was removed at the beginning of winter (DFR). This effect was expressed in both species with average increases of 55 and 83% in C. gayana and P. coloratum, respectively (Figure 2). Noticeably, no plants died throughout the course of the experiment. The change in shoot biomass occurred only in the lower biomass layer in C. gayana (lower layer, P<0.05; upper layer, P=0.73) and in both layers in P. coloratum (lower layer, P<0.01; upper layer, P<0.01) (Figure 2A). The biomass of stolons in C. gayana was not affected by the removal of deferred forage (P>0.05). Total shoot biomass accumulated during the experimental period including growth in spring, winter and that removed at the beginning of the experiment (DFR treatment only), was 53 and 80% higher in C. gayana and P. coloratum, respectively, for the treatment where deferred forage was retained (Figure 2B).
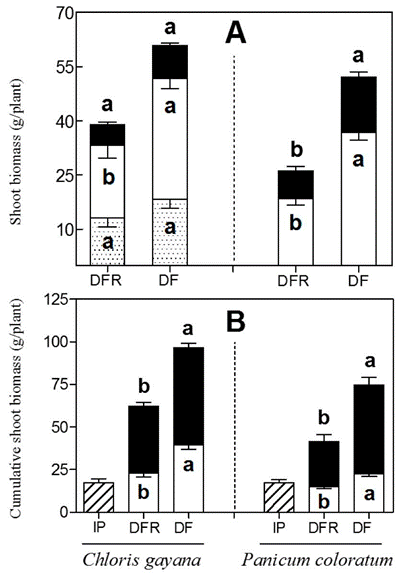
Figure 2 A) Shoot dry matter biomass after spring growth of Chloris gayana and Panicum coloratum plants, allowed to grow during autumn and then subjected to deferred forage removal at beginning of winter (DFR) or left as control plants (DF), then cut in early spring. Forage is divided into upper layer (black bars, >15 cm), lower layer (white bars, <15 cm) and stolons (shaded bars, C. gayana only). B) Cumulative shoot dry matter biomass during the experiment, including spring growth (black bars), removed autumn forage and early spring cut (DFR plants) and early spring cut (DF plants) (white bars) and initial plant biomass (IP). Within plant species and components, bars with different letters are significantly different based on Student's T-test. Values are means and s.e. based each on 10 replicates at each harvest.
The relationships between the biomass located in different plant layers (upper and lower) and both blade and sheath biomass were studied in correlations with total shoot biomass during spring growth. There were significant linear relationships (Table 1, R values higher than 0.80) between accumulated biomass (log-transformed data) of both layers (above and below 15 cm) and total shoot biomass (Figure 3, left panel) in C. gayana exposed to the DF treatment. No linear relationships between measurements of biomass were found in plants in which deferred forage was removed (DFR) in this species (Figure 3, empty points; Table 1, R values lower than 0.17). The situation with P. coloratum was different, since there were significant linear relationships for both treatments across all compartments (Figure 3, right panel; Table 1).
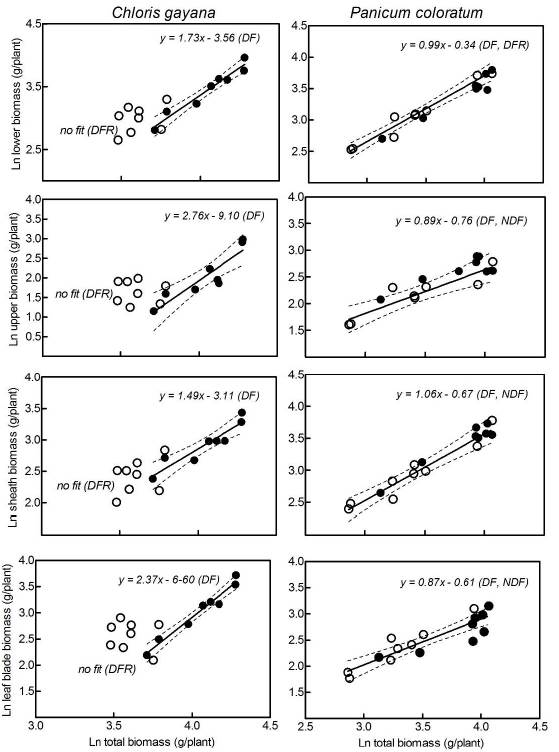
Figure 3 Allometric relationships between Ln of total shoot biomass at the end of spring and: (i) Ln of lower layer biomass, (ii) Ln of upper layer biomass, (iii) Ln of sheath biomass and (iv) Ln of leaf blade biomass of Chloris gayana and Panicum coloratum plants subjected to deferred forage removal at the beginning of winter (open symbols, DFR) and control plants (closed symbols, DF). Note: when slopes and intercepts among fitted regression lines did not differ, data were pooled and a single regression line for both species is presented for clarity, i.e. for P. coloratum.
Table 1 Relationships between Ln of total shoot biomass and: (i) Ln of lower layer biomass, (ii) Ln of upper layer biomass, (iii) Ln of sheath biomass and (iv) Ln of leaf blade biomass of Chloris gayana and Panicum coloratum plants subjected to deferred forage removal at the beginning of winter (DFR) and control plants (DF) (Slopes ± s.e.). R2 values and F values are presented for each fitted regression line.
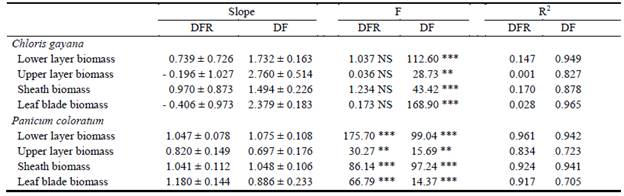
Discussion
The presence of the standing deferred forage during winter produced a positive effect on plant regrowth of both species during spring that could be a result of maintaining the viability of meristems, which produce new vegetative growth after winter, and protecting stem bases from cold (Ferro et al. 2015). Tropical grasses grow very slowly under cool temperatures, as a result of markedly low rates of leaf formation, leaf expansion and photosynthesis (Moore et al. 2004). Since grass regrowth depends mainly on current carbon assimilation (Schnyder and Visser 1999), stem bases are important as a reserve compartment of non-structural carbohydrates (NSC). After the cool season, when tropical grasses begin to grow, these reserves are the main source of carbon until leaves appear and rates of photosynthesis increase as the warm season progresses (de Visser 1997). Some studies with tropical grasses suggest that seasonal variation in the allocation of photo-assimilates is a mechanism used by plants to ensure their survival during winter. Carvalho et al. (2001) observed that Cynodon spp. plants increased the NSC in root bases from mid-spring, while NSC in the base of stems began to decrease as a result of plant growth. In this experiment, removing the deferred forage in early winter possibly promoted vegetative growth at this time, reducing reserves which had accumulated in stem bases during the previous growing season (Ferro et al. 2015). Therefore, DFR plants could have faced winter and early spring with lower stem-base reserves than DF plants.
Regarding the physical effects of herbage cover, we hypothesize that deferred forage may protect plant meristems from the pernicious action of frosts, allowing them to largely remain viable. Thus, the physical barrier provided by deferred grass creates a microenvironment, which safeguards the active meristems from the cold temperatures (Davies and McNaughton 1980). In fact, temperature measurements carried out on stem bases (between 3 and 5 cm above soil surface) support this hypothesis, showing that temperatures recorded in DF plants were 1-3 °C higher than those in DFR plants, where air temperatures were below zero (data not shown). Since active plant meristems are sinks of C and N compounds and more relevant than roots (Wardlaw 1990), DF plants might be able to achieve a rapid transition when temperatures increase in spring, and utilize the stored nutrients to produce early spring growth.
Spring regrowth differences between species could be associated with the better cold tolerance of P. coloratum, which suffered less death of tissues during winter. This species achieved faster regrowth than C. gayana due to its ability to retain more green leaf area at the commencement of spring (Kobayashi et al. 1978). Despite the temperature increase during spring and resulting response in shoot biomass, temperatures had not reached optimal values for maximum growth. This is one possible reason why statistically significant differences in biomass in the upper layer between treatments in C. gayana were not observed. Sustaining plant activity, even if rates of photosynthesis are low, could contribute to preserving NSC reserves located in the roots/crown fraction (top 5 cm of roots and 2.5 cm above-ground stubble) during cold winter conditions. Previous studies have shown that Brachiaria decumbens could increase NSC reserves by maintaining plant activity during less restrictive winter conditions in a tropical climate (Andrade and Marques Neto 1989; Soares Filho 1991), while NSC reserves in Cynodon spp. declined in order to ensure vital functions of tissues, when reduction in leaf area was severe as a result of a hard winter in mid-west USA (Missouri) (Dunn and Nelson 1974). Additionally, structures like stolons or rhizomes are important for the survival of tropical grasses which lack hardy aerial shoots, because their location is generally near soil level where temperatures are warmer (Davies and McNaughton 1980). Biomass of stolons was not reduced in either DF or DFR C. gayana plants; this finding could be important not only in terms of winter plant survival but also for pasture recovery during the warm season.
The practice of retaining deferred forage to reduce cold damage under freezing conditions proved effective in C. gayana. While C. gayana plants, where deferred forage was removed (DFR treatment), suffered significant death of shoot biomass and were strongly stressed, DF plants showed a considerable proportion of green foliage in the lower layer. On the other hand, P. coloratum was able to maintain green foliage on almost whole plants during the cool season in both treatments. Although high cardinal base temperature can be the factor limiting winter growth of tropical grasses to minimal levels, water deficit can be a limiting factor in areas where a marked dry season coincides with the cool season (Jones 1985; Pearson et al. 1985). Accumulation of shoot biomass in late autumn and winter in both species was considerable and was greater under grass deferral (Figure 2B, white bars). However, it is important to mention that the rate of growth in spring was much greater than in winter (see dates in experimental details and Figure 1). The presence of adequate soil moisture during the study probably resulted in pasture growth mainly in late autumn and early spring, when no frost events were recorded (Figure 1, T min line). Other studies (Kobayashi et al. 1978; Ostrowski and Fay 1979; Tischler and Ocumpaugh 2004) under high water availability (rainfall) in southeast Queensland (Australia) found that P. coloratum produced forage growth longer into winter than other tropical grasses.
In tropical and subtropical climates with a marked dry winter season, soil moisture conditions are usually inadequate to support pasture growth, but soil moisture is often adequate in temperate climates (Soriano et al. 1991). This can have a positive impact on productivity because grass response to environmental stresses depends not only on the plant's tolerance of the actual event, but also on the ability to grow after the stress is removed, when water supplies could be adequate for growth (Striker 2008). Studies that analyze growth patterns and water use efficiency in tropical pastures under temperate climates are scarce or even non-existent. The fact that DF plants produced more shoot biomass than DFR plants as well as suffering reduced green leaf death should contribute to identifying management strategies to allow tropical plants to cope better under hard winter conditions. Thus, producing leafy pastures for utilization during the warm season, when forage quality and availability are high, and resting pastures during the cool season, when herbage protects plants from cold and forage quality is low, could be an effective alternative management strategy (see also O'Reagain et al. 2009).
Poorter and Nagel (2000) highlighted the importance of incorporating allometric analysis in order to study biomass allocation under different stress conditions, as many morphological and biomass ratios change based on plant size. Some factors could affect plant growth rate, but not affect biomass allocation to different structures at a given size. This allometric analysis identified that C. gayana was severely stressed in winter, when deferred forage was removed (absence of fitted data, Figure 3), while DF plants of this species were not. We associate the lack of fit between total plant biomass accumulation and different plant compartments with a stressful condition for plant growth in DFR plants (Figure 3, left panels; Table 1, R values). On the other hand, P. coloratum showed a good fit in both treatments supporting previous statements about the good tolerance of this species to these experimental conditions (Figure 3, right panels; Table 1, R values). An allometric analysis in P. coloratum subjected to other environmental stress (complete flooding) showed a strong correlation among shoot biomass reductions, oxidative stress and the absence of fit (Imaz et al. 2012). Similarly, Kollmann et al. (2002) reported that some allometric relationships were radically altered in the ornamental species, Kochia scoparia, in response to over-crowding. These findings agree with previous studies, which demonstrated that P. coloratum can tolerate both moderate chilling and freezing stresses, when used as cultivated forage in temperate livestock systems.
Conclusion
The results obtained in this experiment appear significant for the ecophysiology and grazing management of these tropical grasses. This pot study showed a positive effect on spring growth of retaining forage accumulated during autumn as winter cover. In this sense, P. coloratum was less stressed than C. gayana as reflected in reduced foliage damage and increased shoot biomass accumulation during spring growth, as the allometric analysis has shown. We suggest the following grazing management strategies should be tested for these tropical grasses in temperate environments where frosts occur: (i) allow forage to accumulate in autumn and do not graze or cut it during winter (especially between the first and last frost events) in order to reduce the damage caused by cold and to achieve faster spring regrowth; (ii) commence grazing or cutting in late spring or early summer when temperatures increase and shoot biomass is fully recovered. These strategies need to be verified under field conditions with grazing animals or cutting before recommendations are made to farmers. Future work should also examine forage availability and quality at different times of the year, as part of a temperate pastoral system.