Introduction
Sorghum (Sorghum bicolor) is a dual-purpose crop used for both human food and animal feed in many Asian and African countries (Sarfraz et al. 2012; Bean et al. 2013), with key characteristics being wide adaptability across environments and tolerance to biotic and abiotic stresses (Krishnamurthy et al. 2007; Dahlberg et al. 2011; Gill et al. 2014). The crop residue is used mainly for feeding livestock by small farmers in the Asian and African continents (Hassan et al. 2015). Owing to very high crude fiber and very low crude protein concentrations, sorghum stover left after harvesting grain does not provide quality fodder for milking cattle (Manjunatha et al. 2014).
The contribution of sorghum as a fodder crop has increased the value of production in recent years, so selection criteria in breeding programs could include biomass production and quality as well as grain yield (Hassan et al. 2015). This thinking has been applied in breeding programs with emphasis given to forage quality improvement and selection of nutritious varieties for fodder purposes (Bean and McCollum 2006), as sorghum is the most preferred alternative silage crop after corn (Zea mays) (Kurle et al. 1991). However, feed quality data on the newly bred lines are not available, which makes commercialization challenging (Akabari and Parmar 2014). The important feed trait to be considered is potential of the plant to accumulate high dry matter yields of good quality forage. Sorghum displays wide variability for concentrations of protein, fiber, carbohydrates, crude fat and nitrogen free extract as well as in vitro dry matter degradability (Singh and Shukla 2010; Afzal et al. 2012). Assessment for the anti-nutritional factor, hydrocyanic acid (HCN), also known as dhurrin (further used in text), is also of vital importance. The permissible/safe threshold for HCN in sorghum fodder is 500 ppm (dry matter basis) or >200 ppm (fresh weight basis) (Smitha Patel et al. 2013). HCN is rapidly absorbed into the blood stream of grazing ruminants and can cause cellular asphyxiation and eventually death (Hoveland and Monson 1980). Hence, it is necessary to develop varieties or hybrids with high fodder yields, acceptable quality and low HCN concentrations. Sorghum has good ratooning ability from stubble of the plant crop, which is a desirable trait, as it reduces overall inputs in terms of seed for planting and labor for field preparation (Willey 1990).
Hence, the current study focused on the evaluation of forage dry biomass yield, feed quality and HCN concentration in both the main and ratoon crops of a range of sorghum lines, to aid farmers in choosing the most appropriate lines for feeding to their livestock in particular circumstances and to provide background data for planning future breeding programs.
Material and Methods
Field experiment
A total of 36 improved sorghum lines (Table 1) were evaluated for feed quality and agronomic performance, at ICRISAT, Patancheru, India. The field studies were performed during the rainy season (commencing mid-July) on a medium-fertility vertisol. The experimental design adopted was an alpha lattice design with 2 replications and 6 entries in 6 blocks, with 4 rows of each entry in 0.2 ha. The field was fertilized with di-ammonium phosphate at 80 kg/ha and 40 kg KCl/ha at the time of sowing and top dressed with 145 kg urea 21 days after planting. Immediately after the initial harvest, nitrogen was applied at the rate of 45 kg N/ha (top dressing) and 1 irrigation was provided to increase nitrogen absorption. Seed treatment against soil-borne pests and diseases was performed with thiram at 3 g/kg seed. Seedlings were thinned to 1 plant per hill 3 weeks after sowing, maintaining about 20 cm distance between seedlings and 60 cm between rows; gross plot area was 9.6 m2 (4 rows x 0.6 m x 4 m) and net plot area was 4.8 m2 (2 rows x 0.6 m x 4 m), where the observations were recorded. For each sorghum line the population maintained was about 80-85 plants. The crop was irrigated during sowing and at critical growth stages. The first sampling from the main crop was performed at 15 cm from ground level from the middle 2 rows excluding borders at 80 days after sowing, and the second sampling 80 days later. After the initial harvest, the remaining rows were cut at 15 cm above ground, the forage removed and plants allowed to tiller from the stubble. All agronomic and feed quality parameters were assessed on harvested forage. Agronomic traits recorded during the experiment were: plant height (measured after flowering, from ground level to the tip of the plant); tillering ability (measured by the number of tillers produced by the mainstem in a clump); ratoon scoring (measured by the percentage of plants that produced productive tillers after harvesting; 1: 81-100% stubble tillering, 2: 61-80% stubble tillering, 3: 41-60% stubble tillering, 4: 21-40% stubble tillering and 5: <21% stubble tillering) and dry biomass yield (determined by harvesting all plants in the middle 2 rows and drying in forced-air ovens at 60 °C for 4-5 days).
Feed quality parameters
Quality analysis of forage was performed with 15 plants per line selected at random from each replication, hand-cut into pieces of 4-5 cm length, dried at 60 °C for 4-5 days, later ground in hammer mills to pass through a 1-mm mesh and analyzed at the livestock nutritional laboratory of ILRI in Patancheru. Concentrations of N, acid detergent fiber (ADF), neutral detergent fiber (NDF), acid detergent lignin (ADL) and metabolizable energy (ME) were determined by Near Infrared Spectroscopy (NIRS), calibrated for this experiment against conventional wet laboratory analyses. The NIRS instrument used was a FOSS Forage Analyzer 5000 with software package Win ISI II. Biological fodder quality traits of the forage samples were analyzed for apparent in vitro digestibility using in vitro gas production procedures (Menke and Steingass 1988).
Dhurrin estimation
The youngest leaf (1 leaf from 3 plants per plot) at the booting stage was cut from plants and 100 mg of fresh leaf sample was placed in Eppendorf tubes (2 mL) containing 750 uL of 50% methanol and inserted in a hot water bath at 75 °C for 15 min. The tubes were then cooled to room temperature and 750 uL of 50% methanol was added, to make up the volume to 1.5 mL. Later the tissue was lyzed and centrifuged @ 11,000 rpm for 5 min. One mL of the supernatant was transferred to fresh tubes and stored at 4 °C prior to analysis in Acquity UPLC (Waters, Model D13 CHA 708 G). The mobile phase was 10% acetonitrile and column C-18, with detector-PDA. The dhurrin was detected by monitoring the absorbance at 232 nm (De Nicola et al. 2011).
Statistical analyses
Analysis of variance was performed in linear model for Lattice Design: Yijl = \i + t¡ + y¡ + vi® + Uiji, where: Tj = Treatment effect i= 1, 2,..., t; y¡ = Replicate effect j = 1, 2; Ti(j) = Block within replicate effect l = 1, 2,..., s; and Uiji = Random error. Statistical package, GENSTAT 17 edition for Windows (VSN International, Hemel Hempstead, UK, 2015), was used to analyze the data generated.
Results
Agronomic results
Average plant height (Table 1) was greater in the ratoon crop (2.59 m; range 1.75-3.62 m) than in the main crop (2.28 m; range 1.9-2.7 m). The line IS 31553 (2.70 m) recorded the greatest plant height in the main crop, while IS 13553 (3.62 m) was the tallest in the ratoon. Mean ratoon score in the ratoon crop (shoot regeneration from the stubble of the first harvest) was 3 with a range of 1-4 between lines (P<0.05). Tillering ability (number of additional shoots from main shoot) was higher in the main crop than in the ratoon (5 vs. 3) with ranges of 1 -19 and 1 -14, respectively. The ratoonability of plants was negatively associated (P<0.05) with the number of tillers in the ratoon, reflecting to some extent the scoring system employed. Mean dry biomass yield for the main crop at 80 days after planting was 22.87 t/ha with a range for different lines of 17.32-33.82 t/ha (P<0.05) (Table 1), while mean dry biomass yield for the ratoon crop at 80 days after the first harvest was 8.47 t/ha with a range of 3.2-17.42 t/ha (P<0.05). Individual lines which performed well for the main crop were ICSSH 28 (33.82 t/ha), IS 31553 (27.54 t/ha), Star (28.13 t/ha) and CO 31 (28.40 t/ha), while highest yields for the ratoon crop were recorded with IS 34638 (17.42 t/ha), RSSV 9 (14.13 t/ha) and COS 28 (16.53 t/ha). In terms of total yield (main + ratoon crop) the highest yields came from ICSSH 28 (45.91 t DM/ha), IS 31553 (44.97 t DM/ha) and COS 28 (41.11 t DM/ha).
Table 1 Mean values for agronomic parameters plant height (PH, m), dry biomass yield (DB, t/ha) and dhurrin concentration (DH, ppm) of 36 sorghum lines in main and ratoon crops.
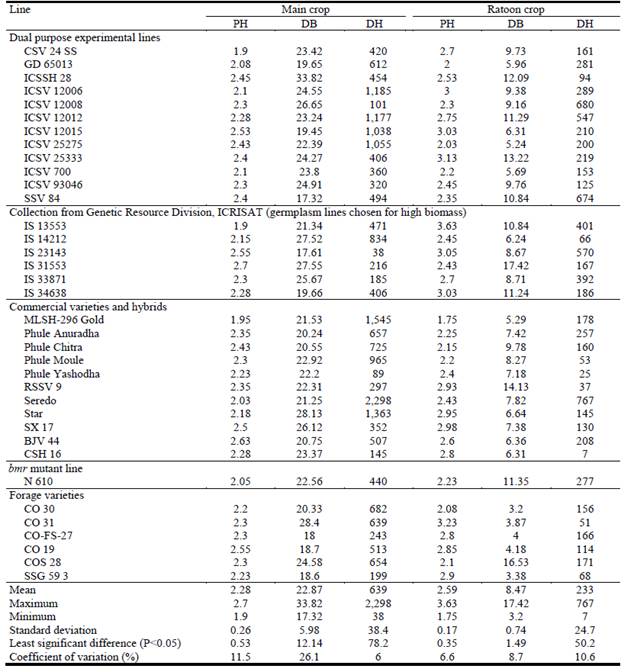
Table 2 Means, ranges and statistical differences for nitrogen, fiber (NDF, ADF) and lignin (ADL) concentrations, metabolizable energy (ME), in vitro organic matter digestibility (IVOMD), dhurrin concentration, plant height, ratooning ability, tiller numbers and dry biomass yield in 36 sorghum lines in main and ratoon crops.
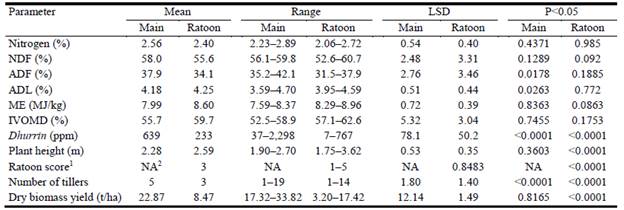
1Scale: 1: 81-100% stubble tillering, 2: 61-80% stubble tillering, 3: 41-60% stubble tillering, 4: 21-40% stubble tillering and 5: <21% stubble tillering. 2NA = not applicable.
Forage quality traits
Nitrogen concentration ranged from 2.23 to 2.89% (mean 2.56%) in the main crop and from 2.06 to 2.72% (mean 2.40%) in the ratoon (Table 2). Similarly, NDF concentration varied from 56.1 to 59.8% (mean 58.0%) in the main crop and from 52.6 to 60.7% (mean 55.6%) in the ratoon. The ADF concentrations also varied between sorghum lines in the main crop (35.2-42.1%; mean 37.9%) and in the ratoon (31.5-37.9%; mean 34.1%) (Figure 1). Acid detergent lignin concentrations varied from 3.59 to 4.70% (mean 4.18%) in the main crop and from 3.95 to 4.59% (mean 4.25%) in the ratoon.
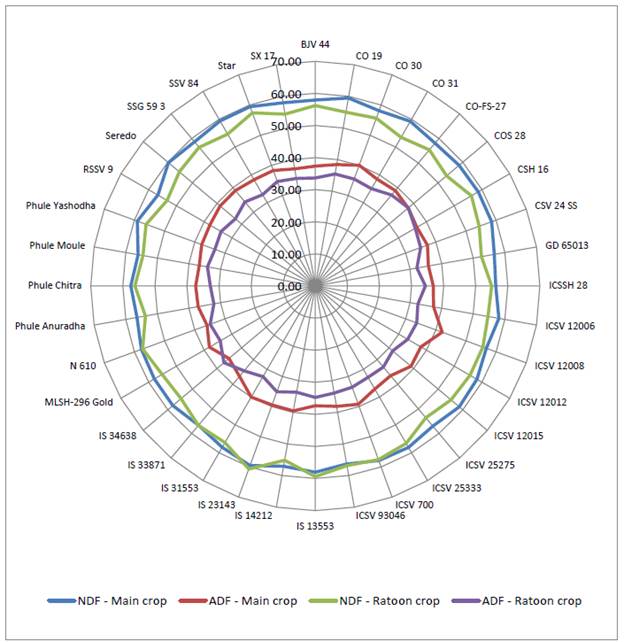
Figure 1 Ranges of neutral detergent fiber (NDF %) and acid detergent fiber (ADF %) concentrations of 36 sorghum lines in main and ratoon crops.
Metabolizable energy concentrations were similar in the main and ratoon crops (mean values 7.99 and 8.60 MJ/kg DM) with significant differences between lines. Mean in vitro organic matter digestibility for the main crop was lower than for the ratoon (55.7 vs. 59.7%) with significant (P<0.05) differences between lines. The dhurrin concentration in the main crop was higher than in the ratoon crop (639 vs. 233 ppm, respectively) (Figure 2). There was extreme variation in dhurrin concentration in different sorghum lines with the commercial hybrid Seredo (2,298 ppm) recording the highest concentration in the main crop and IS 23143 recording the lowest (38 ppm).
Similarly in the ratoon crop, concentration in Seredo was highest (767 ppm), while CSH 16 exhibited the lowest dhurrin concentration (7 ppm). Across the different lines of sorghum evaluated in the experiment, highest N concentrations were recorded in bmr line N 610 (2.90%), the forage line SSG 59 3 (2.86%) and SX 17 (2.81%). Highest ADF concentrations were recorded by ICSV 12008 (42.1%), CO 31 and IS 34638 (40.0 %). The lowest ADL concentrations were observed in MLSH-296 Gold (3.59%), ICSV 700 (3.75%) and ICSSH 28 (3.83%). ME concentration was highest in N 610 (8.38 MJ/kg DM), Phule Yashodha (8.36 MJ/kg DM) and SX 17 (8.29 MJ/kg DM). Similarly, IVOMD was highest in N 610 (58.9%), SX 17 (58.4%) and Phule Yashodha (58.3%).
Correlations
The only significant correlations (P<0.05) between parameters for main and ratoon crops were: positive correlation (r = 0.384) between dhurrin concentrations in main and ratoon crops; positive correlation (r = 0.806) between tiller numbers in main and ratoon crops; negative correlation (r = -0.407) between ratoon score in ratoon crop and number of tillers in main crop; and negative correlation between number of tillers in ratoon crop and ratoon score in the ratoon crop (r = -0.501) (Table 3).
Table 3 Correlations between main and ratoon crops for plant height, dry biomass yield, dhurrin concentration, ratooning score and number of tillers.
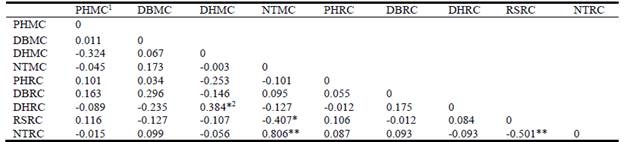
1Plant height main crop: PHMC; dry biomass yield main crop: DBMC; dhurrin main crop: DHMC; no. of tillers main crop: NTMC; plant height ratoon crop: PHRC; dry biomass yield ratoon crop: DBRC; dhurrin ratoon crop: DHRC; ratoon score ratoon crop: RSRC; no. of tillers ratoon crop: NTRC.
2Significant correlations at the P<0.05 (*) and P<0.01 (**) levels.
Discussion
Agronomic results
This study has shown that the sorghum lines that we tested have great potential for production of good quality forage and show sufficient variation to allow selection within breeding programs for further improvement. Quality differences were not as great as dry biomass yield differences, but there were large differences in dhurrin concentration, indicating that there are much greater risks in feeding some lines than in feeding others. While there were marked differences between lines in both main crop and ratoon crop dry biomass yields, total yield (main + ratoon) is probably the most relevant. Lines like ICSSH 28, IS 31553 and COS 28 seemed the most promising in terms of total production and had dhurrin and NDF concentrations which were generally below average. The much higher dry biomass yields in the main crop than in the ratoon may be related to the change in seasonal conditions for growth of the 2 crops and possibly depletion of nutrient levels in the soil. Escalada and Plucknett (1975b), Srinivasa et al. (2011) and Afzal et al. (2012) suggested that higher levels of inputs (nitrogen application) are needed to prevent production differences between main and ratoon crops of sorghum. It was of interest that the ratoon crop was taller than the main crop; thus the higher yields in the main crop were a function of a greater number of tillers and possibly thicker tillers. Despite the lower yields produced, ratooning of sorghum crops for forage production has the advantages of rapid tiller initiation and early maturity but requires more fertilizer application than a corn crop (Ketterings et al. 2004). However, these traits are supplementary to the main objective, i.e. high DM yield of forage for livestock (Undersander et al. 1990; Whish and Bell 2008; Saberi 2014).
Forage quality traits
A shortcoming of this study was that leaf and stem were not separated to assess the yields and quality parameters of these plant parts independently. In our environment farmers chop the fodder and feed it to livestock as a mixture of leaf and stem, which annuls the leaf: stem separation effect. High quality silage can be produced from sorghum by making 2 harvests per season, as opposed to making a single cut at physiological maturity (McCormick et al. 1995). Although the number of tillers produced declines in each succeeding ratoon crop, acceptable yields can be obtained by increasing the plant population (Escalada and Plucknett 1975a). All lines evaluated in the current study recorded N concentrations (both in main and ratoon crops) above that required for effective rumen microbial activity (1-1.2%), a value below which feed intake can be affected (Van Soest 1994; Rai et al. 2012). A total of 26 lines in the main crop and 20 lines in the ratoon recorded N concentrations above 2.4%. The average N, NDF and ADF concentrations were higher in the main crop than in the ratoon crop, possibly mainly due to the relative advantage of fertilizer applied and more favorable weather environment during the growth period of the main crop. Contrastingly, ADL concentration was higher in the ratoon crop than in the main crop. Sweet sorghum lines have recorded high ADF and low ADL, so breeding studies to improve these lines by enhancing the fodder quality traits will expand utilization of dual-purpose lines (Blümmel and Reddy 2006). These differences in quality parameters were not significant across main and ratoon crops, as reported earlier by Srinivasa et al. (2011), even with the various fertilizer levels applied during crop growth. Harvesting the crop immediately post flowering rather than at physiological maturity will produce better quality forage, due to low lignin levels (McCormick et al. 1995). Moreover, the current evaluation was performed in vitro only, and animal feeding trials which measure intake, feed preferences/acceptance, digestibility and absorption are needed to take these preliminary results closer to the adoption stage (Miron et al. 2007).
Interestingly, mean dhurrin concentrations in the main crop far exceeded those in the ratoon crop (mean 639 vs. 233 ppm). However, the extreme variation between lines in dhurrin concentrations, especially in the main crop (37-2,298 ppm), indicates the great potential for selecting lines which are safe for feeding fresh to livestock as either the plant crop or as a ratoon crop. None of the lines recorded levels of dhurrin regarded as lethal (>1,000 ppm DM basis; Smitha Patel et al. 2013) in the ratoon crop. Dhurrin is the main anti-nutritional factor in sorghum, but is known to act as a nitrogen reserve once the crop has overcome the influence of abiotic stress (Park and Coats 2002). It limits the flexibility of using sorghum as a fodder due to its toxic effect when sorghum containing high concentrations is fed to livestock. However, since the dhurrin concentration in sorghum decreases with increase in maturity and the enzyme is deactivated by the process of ensiling as well (Wheeler and Mulcahy 1989), this issue can be managed when fodder is conserved for feeding later. The positive relationship between dhurrin concentrations in main and ratoon crops indicates that a particular line will have a consistent relative concentration whether fed as a plant or ratoon crop. The absence of significant correlation between dhurrin concentration and biomass yield (Table 3) indicates that attempts to lower dhurrin concentrations by breeding should not necessarily affect dry biomass yields of sorghum crops.
Conclusion
While dry biomass yield differences between lines were observed in both main and ratoon crops, there was little quality difference between lines except for the anti-nutritional compound dhurrin. The lines ICSSH 28, IS 31553 and COS 28 were consistently high yielding and could be tested more widely to verify these findings. Use of these lines to develop higher yielding varieties in a forage program would seem appropriate. The wide variation in dhurrin concentration in the various lines and absence of a strong relationship between dhurrin concentration and dry biomass yield indicates a significant potential to breed superior lines with lower dhurrin concentrations without jeopardizing yield.