Introduction
The genus Leucaena contains 24 species (Govindarajulu et al. 2011a), but only one species, L. leucocephala, has a pantropical distribution. According to Brewbaker (2016), it was collected originally from Mexico by Spanish conquistadors and distributed to other Spanish colonies in the late XVI century and now has a global distribution across tropical and subtropical regions of the world. In the last 60 years, L. leucocephala has been the target of modern breeding techniques in USA, Australia and Colombia and several cultivars are commercially available, such as Cunningham (released in 1976), Tarramba (released in 1997), Wondergraze (released in 2010) and Redlands (released in 2017) (Cook et al. 2005; IP Australia 2018).
Leucaena leucocephala is highly valued as a multi-purpose tree for wood and forage production. In the major leucaena-growing area of Australia (central and southeast Queensland), about 130,000 ha of L. leucocephala has been planted for forage production in single or twin rows with inter-row spacings of 6‒12 m with tropical grasses in the inter-rows (Beutel et al. 2018). Bowen et al. (2018) suggest that a L. leucocephala-grass mixture is the most productive forage option for beef production in Queens- land.
Unfortunately, the attributes of L. leucocephala that make it so successful as a forage, e.g. being long-lived, very productive with high nutritive value, competitive with weeds once established and prolific seeding and seedling recruitment, are the same attributes that are beneficial for a successful environmental weed. Hughes (1998) reported that L. leucocephala ssp. leucocephala, in particular, is a well-known invasive weed species in more than 20 countries.
While Leucaena leucocephala is a permitted species in Western Australia (WA), in the north of the state and, in particular, the Kimberley and Pilbara regions, it has not been approved for use on pastoral land leased from the government due to its potential to become an environmental weed (Munday et al. 2018; Revell et al. 2019). Pastoral lease is the dominant form of land tenure for cattle production in the rangelands of WA, with only very small areas of freehold land.
Breeding a sterile forage that will not set viable seeds within the Leucaena genus for use on a pastoral lease would enable the exploitation of the productive potential of leucaena in the region without posing a weed threat. This approach is strongly supported by the Department of Primary Industries and Regional Development of Western Australia and the northern beef industry through Meat & Livestock Australia.
Plant materials
All 24 species of Leucaena can potentially be utilized for breeding and possess a wide range of useful characteristics for breeding purposes being derived from widely differing environments, e.g. low to high rainfall, low to high elevation, intense heat to intense cold (even frost), alkaline, neutral and/or acid soils, a range of pests and diseases, low to high nutritive value, etc. (Hughes 1998; Revell et al. 2019). There are 5 tetraploid and 19 diploid species in the genus and there is a high level of interspecific crossing compatibility among them (Sorensson and Brewbaker 1994).
Three tetraploids and 8 diploid species were selected for their forage potential for the WA breeding program (Table 1).
Table 1 Key descriptive characteristics of the tetraploid and diploid species of leucaena selected for the WA breeding program.
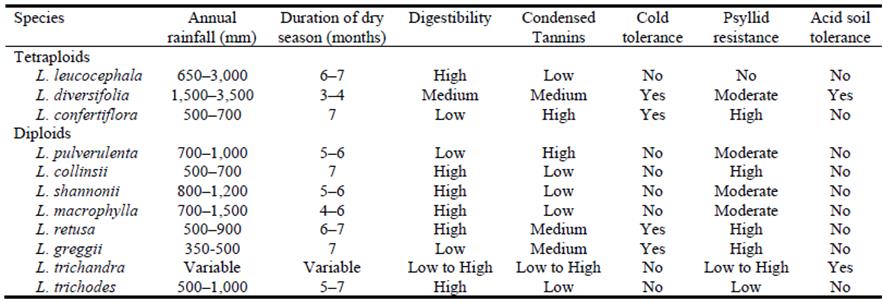
Source: Hughes (1998).
Molecular markers and ploidy level
Modern high-throughput genotyping techniques (Mammadov et al. 2012) are now available for plant breeders. We are taking advantage of existing genomic and transcriptomic materials for Leucaena (Abair et al. 2019) to develop species-specific high-throughput SNP-based marker systems for all taxa. Such markers will aid in the design of specific interspecific crosses and the validation of seeds subsequently produced.
To identify informative species-specific markers we are employing a 2-step process. First, interspecific variation across the coding regions for 18 of the 19 diploid species is being described via transcriptomic (mRNA-seq) resources. For the 19th species, L. pueblana, a lack of available seed resources has resulted in the characterization of overlap-ping variation using gDNA-seq, rather than RNA-seq data. These RNA and DNA data are being utilized in conjunction with the draft genome from L. trichandra (Bailey et al. unpublished data) to conduct variant detection. Accepted uniquely mapped regions are being used to target orthologous loci. The filtered results are then merged for variant calling. Insertions and deletions (Indels) and single nucleotide polymorphisms (SNPs) that pass a minimum mapping quality threshold (phred ≥30) will be retained for post processing and filtered once more for taxonomically informative variants.
Since the RNA-seq resources are derived from just 3 samples per species, the second step towards the development of effective species-specific SNP markers is the screening of available gDNA samples that represent individuals whose identities have been previously confirmed by morphological and molecular approaches (Govindarajulu et al. 2011b). This expanded sampling is critical for the identification of markers that broadly represent each species, rather than subsets of individuals or populations.
Molecular markers will be developed based on the Indel/SNP database and then validated in the Leucaena species. The Indel markers resulting in different amplicon sizes can be efficiently analyzed with agarose or polyacrylamide gel electrophoresis. For SNP screening, the KASP (Kompetitive Allele Specific PCR) genotyping assay will be employed, which is a novel competitive allele-specific PCR for SNP scoring based on dual FRET (Fluorescent Resonance Energy Transfer). Taking advantage of low cost, high throughput and high specificity, KASP assay has been used extensively in SNP genotyping studies in the major crops such as wheat (Neelam et al. 2013), rice (McCouch et al. 2010) and barley (Hill et al. 2018). A final set of diagnostic markers with specific polymorphisms amongst the leucaena collection will be filtered and used for identification tests. Early identification of desirable plants at the seedling stage will save time and costs for the breeding program. DNA markers also have the potential to improve the efficiency and precision of conventional breeding via marker-assisted selection (MAS), if they are associated with desirable agronomic characteristics (Collard and Mackill 2007).
Confirming the ploidy level of the different species and specific elite parents selected from regional field evaluations is also very important, as some of the breeding strategies will involve crossing tetraploids with diploids to produce triploids. Ploidy levels will be measured using a Flow Cytometer. Ploidy and genome size estimates for a few leucaena species have been conducted previously using a FACS flow cytometer (Govindarajulu et al. 2011a). The samples were chopped and stained with propidium iodine, and the species Lactuca sativa was used as a size reference. The flow cytometry was effective for the estimation of most diploid species, but failed for some species, including L. esculenta, L. pulverulenta, L. retusa and L. greggii, that routinely released excessive mucilage upon homogenization (Govindarajulu et al. 2011a). Therefore, the estimates for representatives per species remain unresolved. Recently, a real-time quantitative PCR-based method for the estimation of genome sizes has been developed, based on the absolute quantification of genetic elements in a known amount (mass) of genomic DNA (Wilhelm et al. 2003). The estimation requires specific primer sets that amplify a single copy gene for each species, which would be available from the generated Indel/SNP database and the draft genome of L. trichandra. The real-time PCR-based method is a useful tool for the analyses of large numbers of species, individuals and tissues to investigate the changes in leucaena genome size during phylogenesis and is an effective alternative for samples that cannot be analyzed by flow cytometry.
Breeding strategies
To breed sterile leucaena, we are proposing the following strategies by ploidy level:
Tetraploids
We propose to work with L. leucocephala to develop a non-flowering cultivar. The availability of extensive genomic resources for some legume species such as pea (Pisum sativum) and soybean (Glycine max), and well-documented genetic synteny has enabled a comprehensive inventory of genes potentially relevant for flowering behavior, with specific roles in light perception, photoperiod response, signal integration and inflorescence development (Weller and Ortega 2015). The flowering genes with known functions in legumes and other plant species and a reference genome of L. trichandra would contribute to the gene prediction and identification through homologous alignment. Thus, the finding would shed light on the manipulation of candidate genes for development of non-flowering leucaena lines. New genome-editing biotechnologies, including the clustered regularly interspaced short palindromic repeat (CRISPR), that allows breeders to target specific locations in the genome, hold great potential to speed up crop innovation. In 2018, the Australian Office of Gene Technology Regulator (OGTR) review proposed that organisms modified using site-directed nucleases, without templates to guide genome repair (i.e. SDN-1), would not be regulated as GMOs. The prospect of rapid and efficient genome editing raises concerns related to off-target effects; therefore researchers have engineered changes to the CRISPR-Cas9 genome-editing system that significantly cut down on “off-target” editing errors (Slaymaker et al. 2016). The ‘enhanced specificity’ SpCas9 variants could be useful for precision plant breeding that requires a high level of specificity. Additionally, the newly emerged base editing technology has been used for point mutation repair. Together with previously described base editors such as BE4, Targeted-AID and dCpf1-BE, adenine base editors (ABEs) greatly expand the scope of base editing that enables the programmable installation of all 4 transitions (C→T, A→G, T→C and G→A) in genomic sequences (Gaudelli et al. 2017). If using the reference genome of L. trichandra does not yield the desired outcome, we will endeavor to sequence the genome of the allo-tetraploid L. leucocephala.
Another potential use for gene-editing technology is to develop male- and/or female-sterile L. leucocephala. These gene-edited lines will be useful for producing commercial hybrid seeds with the added benefit of exploiting hybrid vigor as has been extensively used in crops (Horner and Palmer 1995; Saxena et al. 2010; Kim and Zhang 2018).
The CRISPR technology is a new frontier for crop improvement and would serve as a proof-of-concept study on generation of non-flowering leucaena lines and/ or male/female genic sterile lines to produce hybrids.
Triploids
The strategy for producing triploids is to cross tetraploid species (L. leucocephala and/or L. diversifolia) with diploid species that have good forage attributes such as L. collinsii, L. macrophylla, L. shannonii and others.
The main tetraploid species being utilized in the crosses to generate triploids are L. leucocephala, L. diversifolia and L. confertiflora. Leucaena leucocephala is the main commercial species with long-term demonstrated forage attributes (Brewbaker 2016). Leucaena diversifolia is a very productive plant with slightly lower forage quality than L. leucocephala (Hughes 1998), but has already been used as a forage plant (Jones et al. 1998; Sotelo 2017). Jones et al. (1998) evaluated the animal production from L. diversifolia and L. leucocephala for 192 days at Landsdown (North Queensland, Australia). The mean liveweight gain from L. diversifolia CPI 33820 (539 g/d) was not significantly different from that for L. leucocephala cv. Tarramba (664 g/d) though significantly lower than for L. leucocephala cv. Cunningham (723 g/d). In a grazing experiment, at the International Center for Tropical Agriculture (CIAT), the production of a pure grass (Brachiaria hybrid cv. Cayman) was compared with that of a mix of the same grass and L. diversifolia (2,000 plants/ha). Beef production for the grass only treatment was 227 kg/ha in 207 days, while the mix with L. diversifolia produced 552 kg/ha in 207 days (Sotelo 2017).
Leucaena confertiflora is a multi-stemmed tree with maximum height of 4 m, highly resistant to psyllid (Heteropsylla cubana) and possibly cold-tolerant as well (Hughes 1998).
Diploid parents can be utilized directly or following interspecific crosses between diploid species to combine the positive attributes of different diploid species (Table 1). The diploid or interspecific diploid hybrid can then be crossed with a tetraploid to generate a triploid hybrid with combined attributes of 2 or 3 Leucaena species (Table 2).
Table 2 Interspecific crosses conducted in Western Australia during 2018 and 2019 within the Leucaena genus.
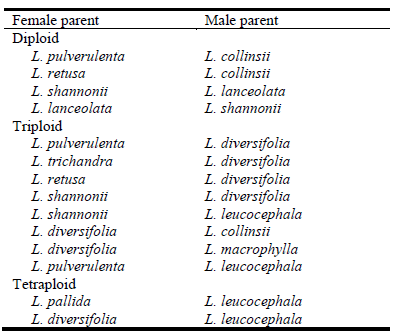
Species and individuals within species will be selected according to their performance at 3 Western Australian field sites located in Carnarvon, Broome and Kununurra representing the target soils and climate. More than 200 accessions from 15 leucaena species have been established as spaced plants in 2018 at each of these locations and will be evaluated for 3‒4 years.
Some crosses are more effective in one direction than in the reciprocal direction and using the tetraploid parent as a female is usually more effective (Sorensson and Brewbaker 1994). We have observed in some cross combinations that, when L. collinsii was used as the female parent, the resulting hybrid pods contained flat and aborted seed. One way forward is to develop an embryo-rescue protocol to rescue the hybrid embryos or, alternatively, do reciprocal crosses with L. collinsii as the male parent to obtain the required hybrid embryos.
Embryo rescue
Embryo rescue is an in vitro technique that aids in the development of weak or immature hybrid embryos that may not survive and develop in vivo into viable seeds and plants. It has been widely used to prevent embryo abortion caused by interspecific incompatibility between the genomes of male and female parents resulting in improper endosperm development and hybrid embryo death (Shen et al. 2011). Embryo rescue involves excising the immature embryos and placing them on nutrient media under sterile conditions for growth and development. Embryo-rescue technology is a valuable tool that can be used for producing seed from interspecific crosses that are not fully compatible. It can also be used to decrease duration of each breeding cycle in compatible crosses. Leucaena seed development takes about 4 months from pollination to seed harvest (Real unpublished data). If this seed-filling phase can be reduced by embryo rescue and in vitro germination, it would accelerate the breeding cycle and allow us to process more than one generation per year (Castello et al. 2015; Pazos-Navarro et al. 2017). We aim to pinpoint the exact time of embryo physiological maturity so we can utilize this technique in leucaena breeding.
Propagation of sterile leucaena
The tetraploid non-flowering L. leucocephala will require vegetative propagation, while triploids can be established by seeds or by vegetative propagation.
Vegetative propagation
In vitro tissue culture and plant regeneration systems have been established previously for the L. leucocephala cultivar K636 (cv. Tarramba). Saafi and Borthakur (2002) reported that green shoots were generated from friable calli derived from cotyledon explants. Shaik et al. (2009) further optimized the system using cotyledonary nodes as explants with a focus on the selection and concentration of auxins and cytokinins in the medium. The tissue culture system facilitated the clonal propagation of tetraploid L. leucocephala and has the potential to be adapted for other species. Recently, an Agrobacterium-mediated transformation protocol to produce transgenic leucaena plants using immature zygotic embryo segments of green seeds as explant material has been developed (Jube and Borthakur 2009).
In order to successfully produce single-cross hybrids in self-incompatible (SI) crops, breeders must be able to generate homogeneous and homozygous parental inbred lines to produce the hybrid. The generation of these inbred lines is impossible in the case of SI. However, the use of doubled haploid technology offers opportunity to develop homozygous lines by generating plants directly from haploid gametes, such as microspores. Following duplication of the haploid genome, or chromosome doubling, the resulting plants are fertile and 100% homozygous. The resulting doubled haploid plants have significant value for plant breeding and gene mapping. The technology has been used for the successful production of spring wheat and barley doubled haploids in Australia (Broughton et al. 2014). A literature review enabled us to identify some general similarities between the protocols regarding explants, medium compositions and culture conditions for haploid plant induction across the various legumes including soybean, chickpea (Cicer arietinum) and lupin (Lupinus angustifolius) (Croser et al. 2006). The haploid research already conducted in species of the Fabaceae family provides hope for further developments for leucaena in the future.
Seed production
Triploid seed production orchards can be established with alternate rows of the selected tetraploid parent and rows of the diploid parent. The diploid parent has to be of a single genotype to exploit the gametophytic self-incompatibility system (Brewbaker 1982) and to achieve self-sterility of the diploid trees. Single genotypes of the diploid parent can be planted via vegetative propagation or by seed. To sow seed, doubled haploid plants will need to be produced and crossed to have a single genotype as follows: S1S1 × S2S2 = S1S2 that can be sown as seed, while producing sterile progenies.
Seeds harvested from the single-genotype self-sterile diploid trees can be produced only by insect pollination with the tetraploid parent. All seed harvested from the diploid rows will be triploid seed. Seeds from the tetraploid trees will be mainly self-pollinated and these seeds would not be harvested.
Conclusions
Strategies to breed sterile leucaena for Western Australia include plant breeding and biotechnology tools to generate sterile lines at both the tetraploid and triploid ploidy levels.
For tetraploids, the main target species is the commercial L. leucocephala that is well known for its potential as a high-quality, productive and persistent forage. Gene editing technologies (CRISPR) will be utilized to edit out flowering genes and develop a non-flowering L. leucocephala and/or create male/female genic sterile lines of L. leucocephala.
For triploids, the strategy is to cross tetraploid species (L. leucocephala and/or L. diversifolia) with diploid species that have good forage attributes, such as L. collinsii, L. macrophylla, L. shannonii and others. Several of these triploid crosses have already been created and will be evaluated in the Kimberley and Pilbara regions of Western Australia for their agronomic performance and sterility.
Successful production of sterile populations will enable the benefits of sowing leucaena on pastoral leases in the Kimberley and Pilbara areas to be realized without concerns about the plants becoming another environmental weed like prickly acacia.