1. Introduction
Concrete is the main material used in construction, as other materials are limited compared to it, by either cost, ease of execution, durability or flexibility to adapt to various geometric shapes [1]. However, concrete is subject to pathological manifestations, which compromise its integrity and durability [2]. Among these manifestations, cracking is a frequent problem, which in addition to an aesthetic damage, can cause leakage and may compromise the entire structure [3].
Moreover, in environmental terms, the production of concrete is unsustainable [4], for the manufacture of the main component, Portland cement, is a major global carbon dioxide emitter [5]. The emission of CO2 from the calcination process of limestone and from the combustion in the furnace of the cement industry accounts for about 7% of global CO2 emissions, making it an important sector for mitigation strategies concerning this pollutant gas [6].
In view of these problems and the need to make concrete a more durable and sustainable material, researches are being conducted on the addition of different materials that provide healing of concrete cracks [7].
In this context, there arises a technological innovation that seeks to minimize the problem of cracks in concrete structures, the bioconcrete. The incorporation of bacteria to the concrete matrix has been used from the bio-precipitation of minerals, with the goal of closing pores and cracks. Thus, the bacteria are considered a self-healing agent of concrete cracks, because they have the ability to precipitate minerals that close the cracks autonomously [8].
However, obtaining a greater understanding of the basic mechanisms and principles of materials that are able to close concrete cracks is still a challenging goal. If this goal is achieved, it will undoubtedly lead to the development of a new generation of self-healing materials [7].
The mineral precipitated by the bacteria in cement materials is from calcium carbonate (CaCO3), which can be precipitated by microorganisms from several mechanisms. The precipitation of CaCO3 promoted by bacteria is classified as a Biologically Induced Mineralization (BIM) [9]. This process will result in minerals formed from three possible ways: enzymatic hydrolysis of urea, dissimilation of nitrates and aerobic metabolic conversion of calcium salts [10]. This last one results in minerals that are formed from an involuntary metabolic activity [11].
After investigation about equations that could summarize possible ways for CaCO3 bioprecipitations, researchers found three main results (Eqs. 1-3) for these biochemical reactions in which the cell surface works as a nucleation site [12].
This bioprecipitations happen as the bacteria finds a calcium source such as limestone rocks or cements. This source, due its negativity, tends to approach the cell wall of the bacteria forming a complex. This complex precipitates calcium carbonate due to the reaction with ion CO3 (Eq. 1). The carbonate and biocarbonate ions dissolved in solutions are formed mainly from the CO2 produced during the microbial respiration (Eq. 2 and 3) [12].
Some bacteria that produce minerals by BIM have been used to repair limestone monuments [13-16] and to fill pores and cracks in concrete and other cementitious materials [17-22].
The success of the use of bacteria to close pores in cement materials is directly related to their ability to form spores [8]. The pH of the concrete is highly alkaline, has no nutrients and there is oxygen only in its open pores and on its surface, tending to restrict bacterial growth [19].
Thus, this research aims at evaluating the crack filling and the compressive strength of two concrete mixtures with addition of B. subtilis spores isolated in Brazil [23] at different concentrations.
2. Microrganisms and growth conditions
2.1. Materials
This study was conducted with B. subtilis AP91, isolated in Brazil from leaves of long grain rice. This bacterium has the potential to bioprecipitate CaCO3 according previous researches [23]. Initially the bacteria was reproduced from two culture medium: Mueller Hinton (MH) (Sigma-Aldrich, USA) and Trypton Soy Broth (TS) (Sigma-Aldrich, USA). These two culture medium were tested to verify in which one the bacteria have the more effectively reproduction ability. From the analysis of this tests, it was select the best culture medium, which provided the highest bacterial growth, enabling to obtain the experiment.
The culture media (MH and TS) were formulated to 500 mL and autoclaved for 15 minutes at 121°C and 1 atm. After sterilization, the bacteria were inoculated in the culture medium and samples were placed in shaker TE 400 (Tecnal) for 66 hours at an average temperature of 29.1ºC to induce bacterial growth.
After the stir period, the solution with the bacteria was washed in order to remove the culture medium and to obtain a more concentrated solution of bacteria. For this, the culture media was centrifuged in Falcon tubes for 20 minutes at 3600 rpm spin, the supernatant was removed and replaced with 0.85% saline solution [1177]. The experiment was repeated twice (n = 2).
Then, the solution containing bacteria was stored for two days at the temperature of 8ºC to induce spore formation. It is important to mention that spores formation are fundamental for the survival of the bacteria in the cementitious materials.
2.2. Quantification of bacteria
Quantification of the bacteria grown in MH and TS were performed with the spectrophotometer UV340GX (Gehaka), with reading at 600 nm. For this test, three samples were used: the control with 0.85% of saline solution, the bacteria grown in MH dissolved in 0.85% saline solution and the bacteria grown in ST dissolved in 0.85% saline solution. To calculate the concentration of bacteria in the medium through the absorbance, Equation 4 was applied [17].
Where:
After complete this stage, the culture medium that resulted in the highest cell growth was identified and then the procedure was repeated with this medium to obtain the required concentrations in the experiment, which are 0.3x108 and 1.2x108 spores/mL according to previous experiment with the bacteria B. pasteurii and Pseudomonas aeruginosa [17].
2.3. Concrete mixture proportions
For the production of concrete mix, the following materials were used: Portland cement, high early strength (CPV ARI, Brazilian denomination); fine aggregate: medium quartz sand; coarse aggregate: gravel 19 mm of basalt, water and B. subtilis spores dissolved in 0.85% of saline solution. The aggregates were characterized according to Brazilian standard [24].
The cement specifications and the requirements of NBR 5733 (Brazilian standard) [25] are presented in Table 1.
Table 1 Characteristics of cement CPV ARI (Brazilian denomination) and the requirements of NBR 5733 (1991).
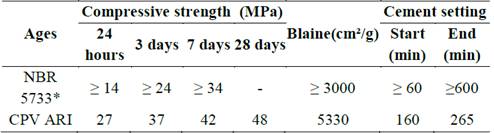
Source: Brazilian standard [25].
Mixture proportions are presented in Table 2. The mix proportions used were 1:1:2 and 1:2:3 (cement: sand: gravel), in mass, with water to cement ratio of 0.33 and 0.45, respectively. For each composition used, two treatments were made with the addition of B. subtilis spores to the mixing water in the concentration of 0.3x108 and 1.2x108 spores/mL. Besides, in the mixing water it was added 0.85% saline solution to maintain the medium isotonic for the bacteria.
The concrete mixture was performed in a vertical shaft mixer and bacteria concentrations were added to the mixing water.
For each treatment, six cylindrical specimens (100x200 mm) were made, being three for visual analysis of crack filling (n=3) over the curing time and the other three for the compressive strength test at 28 days (n=3). It should be noted that, for each mix compositions, reference specimens were made without bacterial spores, with 0.85% sodium chloride to ensure the same conditions as the specimens with spores.
Specimens were molded in 100x200 mm cylindrical samples according to NBR 5738/2015 (Brazilian standard) [26]. The specimens were demolded after 24 hours and stored in a dripping wet chamber at 20ºC ± 2ºC and relative humidity greater than 95% until the test ages, which was 7 days for cracking, 8, 15, 22, 29, 36 and 43 days for the monitoring visual of crack filling, and 28 days for the compressive strength test.
2.4. Simulation and analysis of cracks
In order to evaluate the cracks filling by spores addition compared to reference, a 2 centimeters slice was extracted from each concrete (n=3). These slices were sent to the hydraulic press on the seventh day of healing to apply a strength to simulate a crack, as shown in Fig. 1.
The visual monitoring of cracks during the curing period, at 8, 15, 22, 29, 36 and 43 days, was conducted by images taken from an optical microscope with an attached camera (Olympus), with magnification of 100x. To ensure that the analysis was always held in the same position of the crack, it was demarcated. It is worth mentioning that from this method of cracking simulation it was not possible control it’s thickness.
2.5. Compressive strength
The compressive strength test was performed with non cracked specimens of 100x200mm at the age of 28 days (n = 3) according to a NBR 5739/2007 (Brazilian denomination) [27].
2.6. Statistical design
A statistical analysis was performed only for the evaluation of compressive strength, from a completely randomized design experiment (CRD), in a 2x3 factorial scheme, consisting of two concrete mixtures (1:1:2 and 1:2:3) and three bacteria content (0, 0.3x108 and 1.2x108). In this statistical scheme, the two concrete mixtures were evaluated with all bacterial contents, with three replications.
For the statistical analysis, the software Sisvar was used to perform an analysis of variance [28].
3. Results and discussion
3.1. Quantification of spores
From the two culture medium used in the experiment, the spores quantification of each medium was performed in accordance with Eq. 1., Fig. 2 shows the results of absorbance and concentration of spores/mL of culture media MH and TS.
Through the analysis of Fig. 3, it was observed a higher absorbance values and concentration of spores in the medium MH, of 1.28 and 1.21x108 spores/mL, respectively, while under the same conditions, the medium TS presented, respectively, 0.29 and 1.61x107 spores/mL. From this result, only the culture medium MH was selected for this experiment.
The reproduction procedure was repeated only with the medium MH, which presented the highest concentration of spores/mL, until it reached the concentrations of 1.2x108 and 0.3x108 spores/mL, which was quantified by spectrophotometer.
3.2. Characterization of aggregates
Sand was performed by Particle size analysis [24]. The results of these test were presented in Fig. 3.
The fine aggregate used was a medium sand. The particle size analysis is shown in Fig. 3. It was found that the grading curve is inside the limits established by NBR 7211/2009 (Brazilian standard) [29]. Analyzing the grading curve it should be noted that it has a continuous particle size, with desirable characteristic for use in cementitious materials due to the better packaging of the grains in the structure of the material. For the grading curve, it were calculated the maximum size, specific mass and unit mass. The values found were 2.36 mm, 2.62 g/cm³ and 1.57 g/cm³, respectively. Besides that, the gravel has maximum size of 19 mm.
3.3. Crack filling visual analysis
The visual analysis of crack filling was performed with one crack per specimen until 43 days of curing, being that three specimens of each concrete were analyzed (n=3). The images obtained from an optical microscope with attached camera are shown in Figs. 3 and 4.
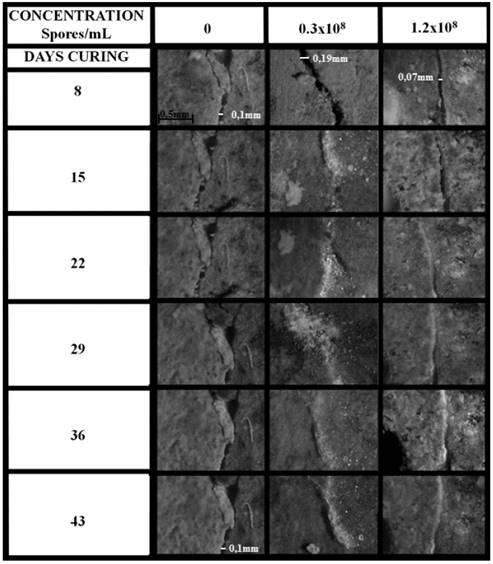
Source: The authors.
Figure 4 Visual monitoring of crack filling in the specimens of composition 1:1:2.
It can be seen that in all the compositions in which bacteria were added, there was a crystal precipitation from the 15th day on, possibly calcium carbonate (CaCO3) according to literature [20,22,30-35]. Besides that, the same strain was used in another study [23] that performed an analysis in vitro of the bioprecipitation of calcium carbonate by pH change during biofilm formation. The authors found that the B. subtilis AP91 were able to precipitate crystals of CaCO3 with the same morphology as other researches [11,36-40].
Other investigators conducted the studies in the same way, first studying whether the bacterium has the ability to precipitate CaCO3 and in the sequence analyzing how that addition would behave in the cement matrix [9,41].
The filling of cracks up to 0.19 mm were visible in concretes with addition of spores of B. subtilis. However, in the samples without spores addition, precipitated material was not visible and the crack remained with the same feature throughout the curing period analyzed.
Although the cracks have different thickness sizes, it was visually found that in the concretes with addition of B. subtilis spores there was filling of the cracks by precipitation of crystals, even if the crack were of greater thickness compared to the reference concrete, without spores. Besides, in the reference concretes this filling was not visualized in this analysis. It is noteworthy that this analysis was just visual. To a better detailing a microstructural analysis would be necessary.
Fig. 6 shows the crystals formed by calcium carbonate precipitation promoted by the bacterium B. alkalinitrilicus added with a nutrient source and curing totally submerged under water for the healing regime [3]. It was observed that there was closure of the cracks from 0.13 to 0.40 mm thickness after 100 days of curing. Similar results were found in this research. Besides the above referenced authors, similar results using the bacterium B. sphaericus have been presented [18].
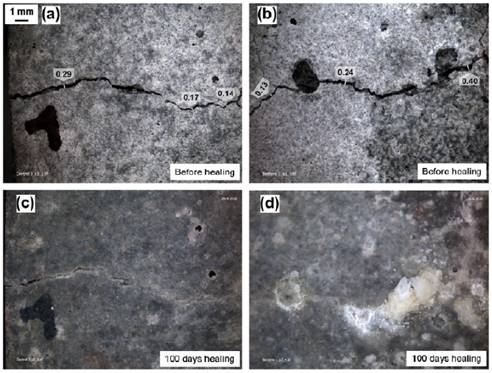
Source: [3].
Figure 6 Stereomicroscopic images of crack-healing process in control mortar specimen before (a) and after 100 days healing (c), in bio-chemical agent-based specimen before (b) and after 100 days healing (d).
Thus, it was observed that the crystals precipitation promoted by the addition of B. subtilis spores under the conditions analyzed was visually satisfactory in crack filling. However, for more specific studies a microstructural analysis would be required.
3.4. Compressive strength
The compressive strength test was performed on the 28th day of curing and the results are shown in Fig. 7. There was a difference in resistance between the compositions due to the proportions of materials used and the water/cement ratio (w/c), with higher resistance for the mix proportion 1:1:2 compared to w/c of 0.33 and without addition of bacteria, and the lowest resistance for the mix proportion 1:2:3 with w/c of 0.45.
Through the analysis of the results, it was noted that there was a decrease in the mean resistance of samples with added spores when compared to the reference. This decrease in the mix proportion 1:1:2 was of 20.06 and 16.58% for the treatments 0.3x108 and 1.2x108 spores/mL, respectively. For the mix proportion 1:2:3, the difference was greater, corresponding to 63.63 and 23.28% for the treatments 0.3x108 and 1.2x108 spores/mL.
There was an increase in the resistance of the samples with 1.2x108 spores/mL compared to the ones with 3.0 x107 spores/mL in both mix proportions, demonstrating that the amount of bacteria added to concrete influences the compression strength. However, it is necessary to test a larger number of bacteria concentrations for a better understanding. According to the literature [31], calcium hydroxide (Ca(OH)2) is the most important source of calcium for concrete, but it does not alter mechanical resistance. On the other hand, calcium carbonate (CaCO3) contributes to increase this resistance, which makes the bioprecipitation a positive contribution [31]. It should be noted that the crystals precipitated by bacteria in cementitious materials, calcium carbonate stand out [12, 20, 22-23, 30-35]. But, to confirm that the crystal precipitated by B. subtilis was calcium carbonate more studies need to be conducted, since this research is a preliminary test.
3.4.1. Statistical analysis
The statistical analysis was performed in Sisvar [28] and the results of analysis of variance are shown in Table 3. The sources of variation were the mix proportions of concrete and concentration of added spores, as well as the interaction of these two sources of variation.
There was a significant difference (P < 0.05) at 5% probability, for the parameter compressive strength in the variation of Mix proportion (M) and Spores Concentration (C), indicating that there are variations to compressive strength according to the concentration of spores added and to the mix proportion analyzed.
Table 4 presents the tests of means performed for the parameter compressive strength with variation of Mix proportion (M) and Table 5 present the test of means for the parameter Spores Concentration (C).
Table 5 Tukey test for compressive strength with the addition of B. subtilis spores
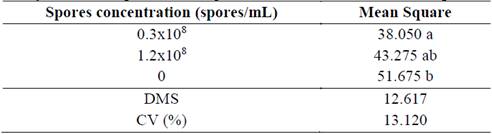
Source: The authors.
It was noted that there were significant differences in compressive strength by varying both the concentration of spores added and the mix proportion. A significant difference between the compressive strengths of the two mix compositions were expected, since the w/c ratio is a factor directly related to it [6].
Regarding the addition of bacterial spores, it was observed that the lowest concentration of spores resulted in a minor compressive strength, significantly differing from the reference (without addition of spores). The concentration of 1.2x108 spores/mL presented minor compressive strength than the reference; however, there was no significant difference between them.
Based on this analysis (Tukey test), it’s possible that the addition of spores of B. subtilis could be used in concretes with these specific mix proportions without significant influences in compressive strength, nevertheless it is necessary more investigations about the use of this kind of material, since this study is a preliminary analysis.
4. Conclusion
From the experimental results, it was verified that there was a visual crack filling when the B. subtilis spores were added in two studied concentrations (0.3x108 and 1.2x108 spores/mL). However the analysis by microscope with attached camera is just a visual analysis. To confirm that there was a closure of cracks a microstructural analysis would be necessary.
The addition of 0.3x108 spores/mL resulted in a statistically significant decrease (at 5% probability) of the compressive strength of the specimens, while the addition of 1.2x108 spores/mL did not present statistically significant differences when compared to the reference concrete. It possible happened because the highest concentration of spores was capable to produce more crystals that could increase the strength. Although further analysis need to be performed to confirm this theory.
Thus, these preliminary study has shown that the addition of B. subtilis spores isolated in Brazil to unarmored concrete could be beneficial and this practice should be developed in order to obtain a material capable of self-healing and consequently increase its lifetime. For this, further studies on the concentration of addition of these spores are required, as well as on the adding procedure, curing process and microstructural analysis.